(1)
Department of Pediatric Dentistry, Selcuk University, Faculty of Dentistry, Konya, Turkey
Abstract
Both SHED and DPSC have characteristic indistinguishable fibroblastic characteristics (Fig 6.1). They preserve plasticity during at least 25 passages, while maintaining the normal karyotype and the rate of expansion characteristic of stem cells (Kerkis et al. 2006).
6.1 Phenotypic Characteristics
Both SHED and DPSC have indistinguishable fibroblastic characteristics (Fig 6.1). They preserve plasticity during at least 25 passages, while maintaining the normal karyotype and the rate of expansion characteristic of stem cells (Kerkis et al. 2006).
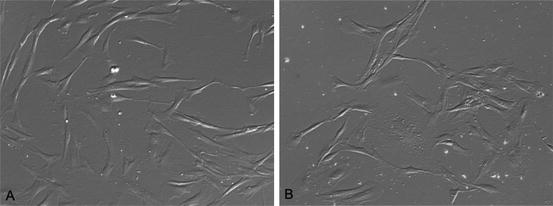
Fig. 6.1
Indistinguishable fibroblastic phenotypes of SHED (a) and DPSC during the first days in culture (b) (inverted phase-contrast microscopy, 400×)
Using flow cytometry, cell surface markers analysis of DPSC shows consistently that they are positive for CD73, CD90, CD105 (endoglin), CD13, CD29 (integrin b1), CD44, HLA-A, B, and C. They are negative for CD45, CD34, CD14, CD54, CD 133 (Oktar et al. 2011; Chen et al. 2012), thus they fulfill the criteria of MSC set by the ISCT (Dominici et al. 2006). Besides confirming the findings described above, it has been shown that SHED and DPSC express pluripotency markers including Oct4, Nanog, c-Myc, Sox2, stage specific embryonic antigens (SSEA-3, SSEA-4), and tumor recognition antigens (TRA-1–60 and TRA-1–81) (Kerkis et al. 2006; Nakamura et al. 2009; Govindasamy et al. 2010; Liu et al. 2011). However, it is important to note that these expression levels might not be compatible with the values observed in embryonic stem cells (Lengner et al. 2007). For further in vitro phenotypic characteristics of DPSC, the paper from Huang, Gronthos, and Shi provides clear ideas regarding the differences between DPSC, SHED, and the other tooth-originated stem cells (Huang et al. 2009).
Moreover, a cDNA microarray system-based comparison between human DPSC and human MSC has been published recently (Yamada et al. 2006). Broadening the previous gene expression profile study for 4,000 human genes in DPSC and bone marrow stroma stem cells by Shi et al. (2001), Yamada et al. revealed several genes which encode the extracellular matrix components, cell adhesion molecules, growth factors, and transcription factors including Msx1 and Jun-B. According to their cluster analyses Yamada et al. concluded that there are differences in gene expression levels for cell signaling, cell communication, and cell metabolism (Yamada et al. 2006). Using integrated miRNA and mRNA expression profiles, Chen et al. compared DPSC and UCSC (Chen et al. 2012). They determined by functional network analyses that two genes (SATB2 and TNFRSF11B), which are involved in ossification, bone development, and actin cytoskeleton organization, are signatures of DPSC. They also showed upregulations of NEDD4 and EMP1, which are shown to be involved in neuroectodermal differentiation in DPSC (Chen et al. 2012). Additionally, Pivoriuonas et al. revealed cytosolic and nuclear proteins of SHED and reported a close resemblance between SHED and MSC-like cells from other tissues (Pivoriuunas et al. 2010). Despite the fact that dental pulp is a heterogeneous tissue, availability of “omics” data might help to develop more targeted isolation methods of DPSC in the future.
6.2 Multidifferentiation Capacity
DPSC are multipotent since they are capable of giving rise to various lineages of cells, when exposed to the appropriate environmental cues. Most of the DPSC have been shown to differentiate in vitro into the desirable cells through a well-defined transcriptional cascade. Albeit the majority of the studies have focused on the ability of DPSC to differentiate into odontoblast/osteoblast-like cells (Couble et al. 2000; Gronthos et al. 2000; Laino et al. 2005; Cordeiro et al. 2008; Bakopoulou et al. 2011; d’Aquino et al. 2011; Min et al. 2011), they can differentiate into functionally active neurons (Arthur et al. 2008; Kadar et al. 2009; Kiraly et al. 2011; Nourbakhsh et al. 2011; Osathanon et al. 2011), mature melanocytes (Stevens et al. 2008; Paino et al. 2010), smooth muscle cells (Kerkis et al. 2006; d’Aquino et al. 2007; Gandia et al. 2008), islet-like aggregates (Govindasamy et al. 2011), and hepatic cells (Ishkitiev et al. 2010) as well.
The classical differentiation recipes are as follows: Osteogenesis can be initiated experimentally with DMEM-LG (low glucose) with 10 % (v/v) FBS, 100 nM dexamethasone, 0.2 nM ascorbic acid, 10 mM β-glycerophosphate; in the case of chondrogenesis 10 ng/mL TGFβ3, 100 nM dexamethasone, 50 μg/mL ascorbic acid, 1 mM sodium pyruvate, 6.25 μg/mL insulin, 6.25 μg/mL transferrin, 6.25 ng/mL selenous acid, 1.25 mg/ml bovine serum albumin, and 5.35 mg/ml linoleic acid in DMEM-high glucose can be used. Last but not least, adipogenesis can be initiated with the xenobiotics such as 1 μM dexamethasone, 500 μM IBMX, 60 μM indomethacin, and 5 μg/ml insulin in DMEM-LG supplemented with 10 % FBS. Oil red O staining of 4 % PFA fixed cells and toluidin blue staining in cryosections of cell nodules in micropellet systems are universally accepted visualization methods for lipid droplets in adipogenically induced cells, and accumulation of extracellular matrix composed of mucopolysaccharides in chondrogenically-induced cells, respectively (Karahuseyinoglu et al. 2007a, 2008; Oktar et al. 2011; Yildirim et al. 2012). Despite some pitfalls in von Kossa and Alizarin Red S (Bonewald et al. 2003; Wang et al. 2006), they are still in use for expected collagenous extracellular matrix formation and mineralization in osteogenically induced cells (Karahuseyinoglu et al. 2007a, 2008; Oktar et al. 2011). While von Kossa shows only calcium accumulations, Alizarin Red S reacts with calcium cations to form a chelate. Although other stains such as two fluorescent dye combinations (xylenol orange and calcein blue) have been suggested, staining methods alone are not appropriate for the identification and quantification of bonelike minerals. Hence, using more specific diagnostic techniques such as X-ray diffraction, electron microscopy, or Fourier transform infrared spectroscopy (FTIR) may better verify the presence and quality of calcium phosphate phases (Bonewald et al. 2003).
6.2.1 Problems in Adipogenesis
While most of the differentiation assessments revealed strong osteogenic and condrogenic potential for DPSC (Gronthos et al. 2000, 2002; Huang et al. 2006; Iohara et al. 2006; Kerkis et al. 2006; Liu et al. 2006; Honda et al. 2007), there are no consistent reports of their adipogenic potential (Gronthos et al. 2000; Pivoriuunas et al. 2010; Oktar et al. 2011; Struys et al. 2011). We compared the differentiation potentials of human DPSC and UCSC (Oktar et al. 2011). While chondrogenic and osteogenic differentiation of both cell types were similar, their adipogenic differentiation within the same conditioned media resulted in different outcomes. While UCSC started to display specific intracytoplasmic lipid granules in the central cytoplasm after only 48–72 h following adipogenic treatment, DPSC protected their fusiform shape without showing any lipid granules even after prolonged treatments of up to 5 weeks. Furthermore, following successful adipogenic induction of UCSC, we found that nucleostemin (NS), which has been shown as a nucleolar protein expressed in stem and cancer cells, could no longer be detected in forming preadipocytes. However DPSC that did not respond to adipogenic induction displayed a stable NS expression (Oktar et al. 2011). Other groups also noted the non-responsiveness of DPSC to adipogenic stimuli. Pivoriunas et al. reported the same results for adipogenic differentiation of DPSC after several trials (Pivoriuunas et al. 2010).
Although DPSC have been reported to possess an adipogenic differentiation capacity under our conditions (Huang et al. 2009; Koyama et al. 2009), this discrepancy may be due to several factors including individual differences. The environmental conditioning or predisposition of the stem cells might be reflected in their distinctive differentiation ability. Their fate in vivo might be dependent of their embryonic origin and their anatomical localization. Moreover, teeth are derived from the ectoderm of the first branchial arch and the ectomesenchyme of the neural crest. Deciduous teeth start to form between the sixth and eighth weeks of gestation, and permanent teeth begin to form in the twentieth week (Thesleff et al. 1995). Umbilical cord, on the other hand, develops from the extra-embryonic mesoderm at days 12–13 after fertilization. Likewise, Struys et al. claimed that dental pulp is confined by a hard dental tissue (dentin) which is more likely to create a tendency for osteogenic events in those cells (Struys et al. 2011). However, the observed strong osteogenic response of umbilical cord intervascular cells in our experimental conditions does not support this hypothesis (Yildirim et al. 2012). Osteogenic differentiation was robust in both MSC, while the staining pattern of von Kossa was completely different. DPSC displayed more heterogeneous widespread small, round, and dark stained mineralized structures, whereas UCSC showed bone-nodule like formation (Yildirim et al. 2012). Therefore, the diverse developmental lineage and differential potential of UCSC and DPSC may reflect stem cell heterogeneity. It has been suggested that the propensity of mesenchymal stem cells derived from various tissue sources toward a specific lineage is different from each other (Kerkis and Caplan 2012). This hypothesis deserves further exploration.
6.2.2 Odontogenic Differentiation
Spontaneous differentiation of DPSC toward several lineages has been shown (Kerkis et al. 2006; Paino et al. 2010; Yu et al. 2010; Lizier et al. 2012). Odontoblasts are not one of those lineages assuming that the heterogeneous nature of DPSC cultures, and growth and proliferation conditions could not provide the necessary permissive signals for odontoblast terminal differentiation. Papaccio’s group has shown that in the presence of 20 % of FBS, bone is the main commitment of dental pulp stem cells, but not dentin (Laino et al. 2005, 2006; Papaccio et al. 2006; d’Aquino et al. 2007). On the other hand, DPSC and SHED have been shown to have odontogenic potential with several different in vitro and in vivo evaluation methods, in addition to quite different isolation, culturing, and differentiation methods (Table 6.1). What is more, no specific marker(s) is known to differentiate between odontoblasts and osteoblasts. While beta-glycerophosphate, ascorbic acid, and dexamethasone have been used for odontoblast differentiation, researchers are trying to find a unique way for in vitro odontoblast differentiation. Table 6.1 shows different odontogenic induction techniques and evaluation methods.
Table 6.1
Evaluation of odontogenic differentiation by different research groups
Group
|
Odontogenic induction by
|
Evaluation markers
|
Evaluation methods
|
Results
|
---|---|---|---|---|
Couble et al. (2000)
|
Culturing DSPC in EBM supplemented with 10 % or 15 % FCS ± β-GP
|
Coll type I, DSP
|
Electron microscopy, X-ray microanalysis, RT-PCR
|
Inducing odontoblastic features including spatial organization similar to odontoblastic layer
|
Gronthos et al. (2002)
|
In vivo: transplantation of DPSC with HA/TCP ceramic powder subcutaneously into immunocompromised mice
|
Histology
|
Generation of ectopic dentin/pulp-like complexes
|
|
Batouli et al. (2003)
|
Transplantation of DPSC with HA/TCP ceramic powder subcutaneously into immunocompromised mice
|
DSP
|
Histology, histochemistry
|
Formation of vascularized pulp-like tissue surrounded by a layer of odontoblast-like cells expressing DSP
|
Huang et al. (2006)
|
DPSC cultured in α-DMEME including 20 % FBS 2 mM KH2PO4, 20 mM HEPES, and 100 nM dexamethasone; or these three chemicals plus 5 × 10−8 M Vit D3
|
Alizarin red S staining
|
Promoted mineral nodule formation
|
|
Cordeiro et al. (2008)
|
SHED seeded in dentin slice/PLLA scaffolds were transplanted into immunodeficient mice
|
Human factor VIII, DSP
|
Histochemistry, B-galactosidase staining, TEM
|
Pulp-like tissue with functional blood vessels lined with odontoblastic cells
|
Nam et al. (2011)
|
DPSC were populated on porous calcium phosphate granules
|
DSPP, DSP, Col type I, DMP1, OCN
|
RT-PCR, WB, Alizerin red S staining
|
Odontogenic development supported by the upregulation of DSPP, DMP-I, Coll type I, and OCN
|
Lee et al. (2011)
|
Preameloblast-derived factors
|
DSPP, BSP, Nestin, Coll type I, RUNX2, ALP, OC, mCpne7
|
Real time PCR, WB, Alizerin red S staining, dentin sialophosphoprotein promoter activity, liquid chromatography–mass spectrometry
In vivo: transplantation into immunocompromised mice
|
Facilitated odontoblast differentiation and generation of pulp-like structures lined with human odontoblast-like cells showing typical odontoblast processes
|
Many other factors have been shown to promote odontoblastic differentiation of DPSC, such as porcine tooth germ conditioning medium (Wang et al. 2011), the combination of MTA and enamel matrix derivatives (Min et al. 2009), FG2, TGFβ1, BMP-2, GDF-11, DMP-1 derived peptide, asporin and EphB/ephrin-B (Iohara et al. 2004; Nakashima et al. 2004; Yang et al. 2007; He et al. 2008; Arthur et al. 2009; Chaussain et al. 2009; Yang et al. 2009; Casagrande et al. 2010), inhibition of Delta 1 (Wang et al. 2011), TWIST1 over expression (Li et al. 2011), Bmi-1 transduction (Mehrazarin et al. 2011), the inhibition of mammalian target of rapamycin (mTor) complex Torc 2 (Kim et al. 2011), pro-inflammatory cytokines (IL-1 and TNF) (Yang et al. 2012), simvastin (Okamoto et al. 2009), conditioned medium extracted from tooth germ cells (Duailibi et al. 2004; Ohazama et al. 2004
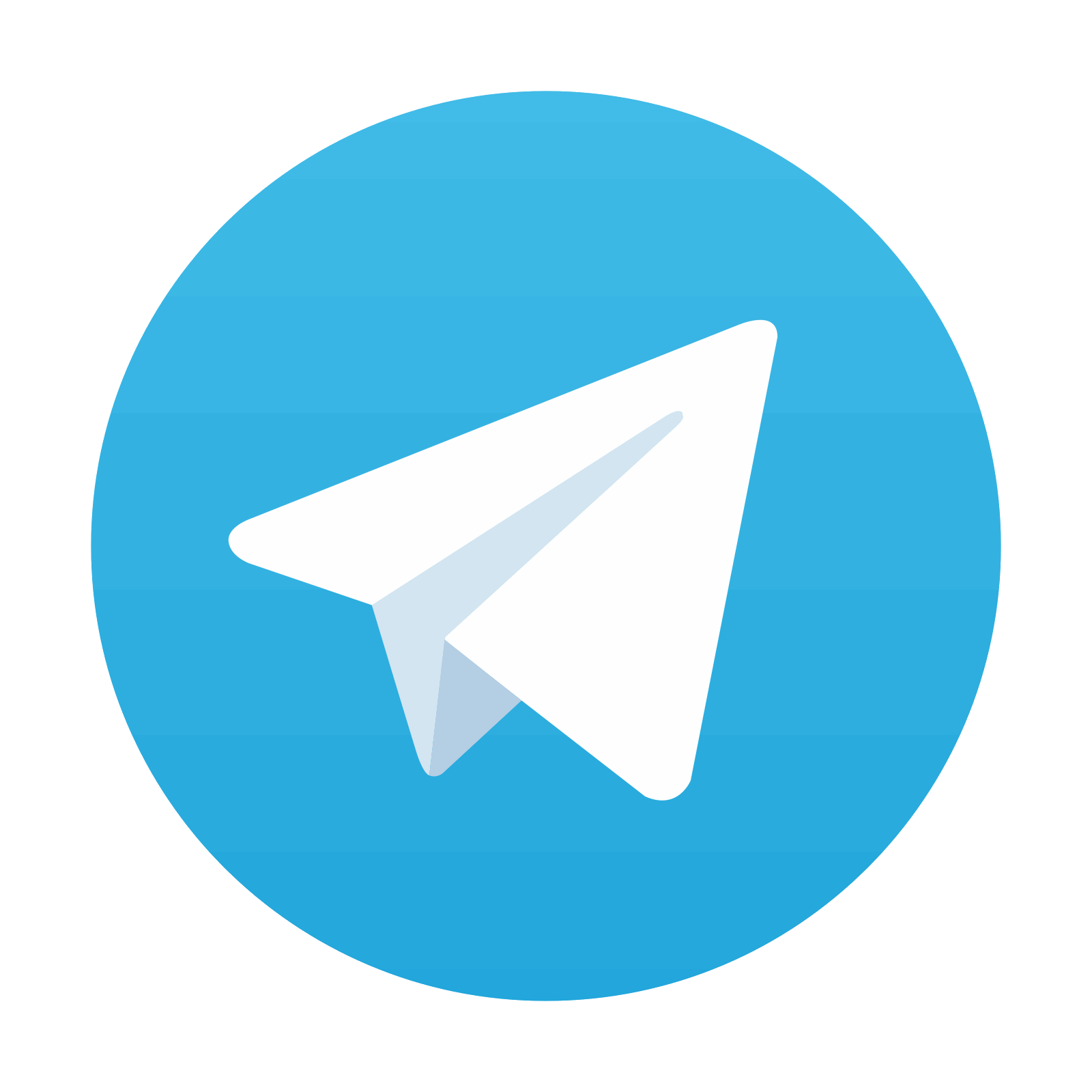
Stay updated, free dental videos. Join our Telegram channel

VIDEdental - Online dental courses
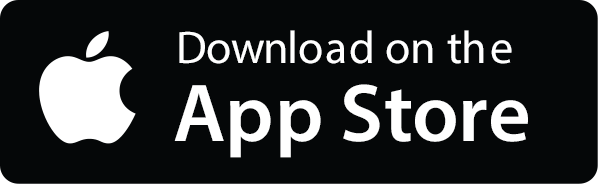
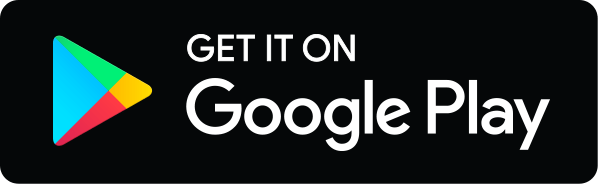