Fig. 10.1
(a) SEM micrograph of sclerotic dentin lesion showing a dentinal tubule that was heavily occluded with large cubic whitlockite crystallites (pointers) (Modified from Tay and Pashley (2004a)) (b) SEM of a fractured surface of sclerotic dentin showing how many small mineral deposits can coalesce into a solid cast within dentinal tubules in a sclerotic lesion. T dentinal tubule (Modified from Tay and Pashley (2004a))
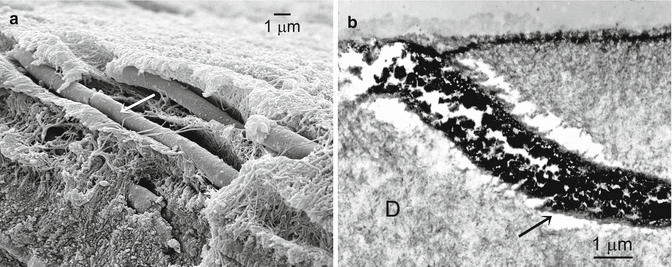
Fig. 10.2
(a) SEM micrograph of intratubular sclerotic casts (white arrow) that blocked the dentinal tubules along the surface of a sclerotic lesion (Modified from Tay and Pashley (2004a)) (b) TEM micrograph taken from an undemineralized section of the surface of a bonded sclerotic lesion. Tubules were obliterated with sclerotic casts that consisted of both fine electron-dense crystallites and spherical aggregates of crystals (black arrow). A thin, electron-dense, hypermineralized layer was also evident along the surface of the lesion (Modified from Tay and Pashley (2004a))
10.3.2 Hypermineralized Surface Layer in Shiny Sclerotic Lesions
Unlike tubular occlusion, the presence of a hypermineralized surface layer in natural cervical sclerotic wedge-shaped lesions can only be seen through the use of microradiography (Weber 1974) or FTIR photoacoustic spectroscopic analysis (Mixson et al. 1995). Although it has been speculated that the hypermineralized surface layer is devoid of collagen fibrils (Duke and Lindemuth 1991), the ultrastructural features of this layer were only verified recently. Figure 10.3 is a toluidine blue-stained, undemineralized thin section taken from the deepest part of a wedge-shaped defect. In the preparation of the specimen, the surface of the lesion was not cleaned, but was fixed in Karnovsky’s fixative prior to the preparation (Duke and Lindemuth 1991). A surface layer composed of stained, unmineralized filamentous bacteria could be seen, beneath which was an approximately 15 μm thick hypermineralized layer. Mineralized bacteria could be faintly seen within this layer. At a higher magnification (Figs. 10.4a, b), platelike minerals could be recognized within the matrix around the bacteria.
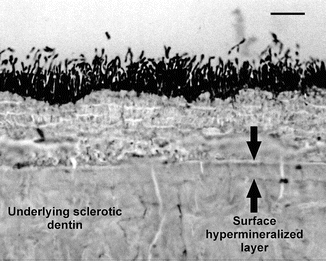
Fig. 10.3
Light microscopic image of an undemineralized section taken from the deepest part of a stained, unmineralized wedge-shaped sclerotic lesion. Bacteria cover the underlying hypermineralized layer of an untreated noncarious cervical sclerotic lesion. The hypermineralized nature of the surface layer was recognized by comparing its electron density with the underlying sclerotic dentin (Modified from Tay and Pashley (2004a))
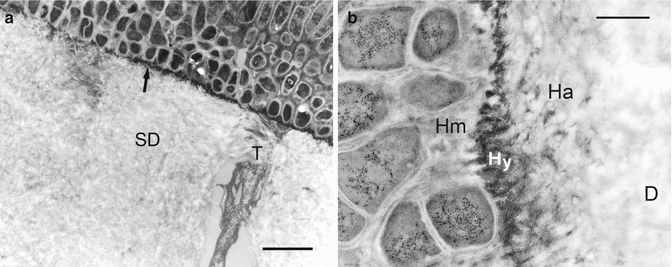
Fig. 10.4
(a) Undemineralized TEM micrograph of multiple layers of bacteria growing on top of a hypermineralized layer (arrow). The intermicrobial matrix (i.e., the material between adjacent bacteria) was partially mineralized. Note lamina limitans sheath within an open dentinal tubule (Modified from Tay and Pashley (2004a)) (b) Resin-hybridized sclerotic dentin. Ha designates an authentic hybrid layer created by a mild self-etching primer/adhesive. Hy designates the resin-hybridized hypermineralized layer on the dentin surface. Hm refers to resin hybridization of the bacterial organic matrix. D underlying sclerotic dentin; bar = 300 nm (Modified from Tay and Pashley (2004a))
The ultrastructure of the surface hypermineralized layer was highly variable within the deepest part of the wedge-shaped lesions. The mineralized layer was retained after complete demineralization of the underlying sclerotic dentin. The hypermineralized (HM) layer in Fig. 10.5 consists of several thin, discontinuous layers that were sandwiched among different bacterial species. Each colony of bacteria was mineralized prior to the deposition of the next colony. It is pertinent to point out that the specimens that contained bacteria were brushed cleaned with a mixture of chlorhexidine and pumice prior to laboratory processing. Such lesions appeared as clean, highly shiny lesions when examined under magnification.
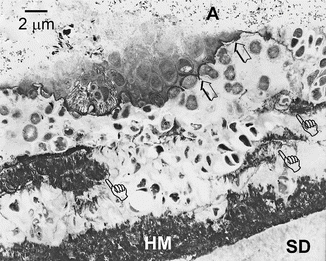
Fig. 10.5
Variation in the ultrastructure of the complex hypermineralized layer in noncarious cervical sclerotic lesions. Demineralized TEM micrograph showing a hypermineralized layer (HM) within the deepest part of a wedge-shaped lesion that was 14 μm thick. Bacteria colonies were trapped inside this thick layer (open arrows). Another species of bacteria (pointers) accumulated along the surface of the hypermineralized layer (Modified from Tay and Pashley (2004a))
The presence of bacteria on the surface of sclerotic wedge-shaped lesions is consistent with the report of Spranger (Spranger 1995). The structures beneath bacterial plaque change over time depending on the metabolism of the microorganisms. This results in substantial pH fluctuations along the tooth surface (Scheie et al. 1992). Bacterial products may trigger gingival inflammation. If a plentiful supply of fermentable carbohydrates is available, the microbes would release organic acids that lower the plaque pH and tend to demineralize the underlying dental hard tissue. When the carbohydrate source is depleted, the local salivary buffering would increase the pH of the lesion, and remineralization of the dental hard tissue and mineralization of the dental plaque could occur. In the absence of carbohydrates, these bacteria may remain viable for prolonged periods (Svensäter et al. 2001), utilizing glycogen-like intracellular polysaccharide (IPS) as a metabolizable source of carbon (Spatafora et al. 1999). They may also metabolize amino acids and other nitrogenous substrates, creating ammonia and other basic chemicals that may elevate plaque pH and promote mineralization and, perhaps, hypermineralization (Sanz and Newman 1994).
Along the occlusal wall of the wedge-shaped lesions, both the surface bacterial layer and the hypermineralized layer are usually thin (1–2 μm thick). Similarly, the gingival wall of a wedge-shaped lesion, which is usually more accessible to toothbrushing, is usually devoid of bacteria. However, a very thin surface hypermineralized layer may sometimes be observed (Fig. 10.6) on the gingival wall. Such a layer, if present, is 200–300 nm thick and may be readily seen in undemineralized sections by its increased electron density, as well as the characteristic arrangement of the crystallites, which will be discussed in the next section.
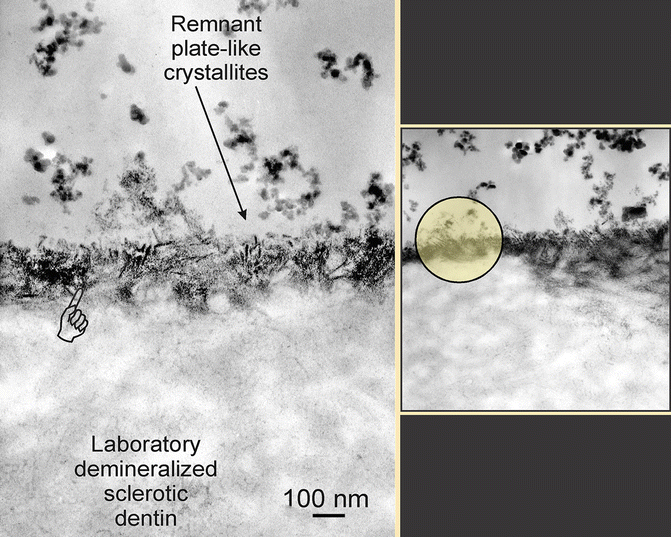
Fig. 10.6
Crystallites (arrow) within a surface hypermineralized layer (pointer) were platelike and were aligned along the c-axis. The lesion surface was etched by a self-etching primer, causing blunting and rounding of the partially dissolved crystallites
10.3.3 Mineral Distribution
Mineral crystallites present within the hypermineralized layer are larger in size compared with those within the underlying sclerotic dentin (Fig. 10.6). Unlike crystallites within the underlying dentin that are randomly arranged, those found in the hypermineralized layer were longitudinally aligned along the c-axis of the crystallites. Piezoelectric potentials have been reported to be generated when teeth are subjected to parafunctional loading (Marino and Gross 1989). Some have speculated that polarization of the remineralized crystallites caused by piezoelectric potentials created during eccentric tooth flexure results in their attraction and repulsion by dipole interaction.
10.3.4 STEM/EDX Analysis
Hypermineralization implies that the density of the mineral within the surface layer of the defect is higher than that of the underlying sclerotic dentin. This is confirmed by a qualitative STEM/EDX line scan of the calcium and phosphorus distribution longitudinally across the surface layer of the defect into the underlying sclerotic dentin (Fig. 10.7). Also evident in the line scan is the presence of a region of decreased calcium and phosphorus counts along the top 500 nm of the hypermineralized layer that is attributed to partial demineralization by a self-etching primer (Fig. 10.7) that was used to bond to sclerotic lesions (Tay and Pashley 2004a).
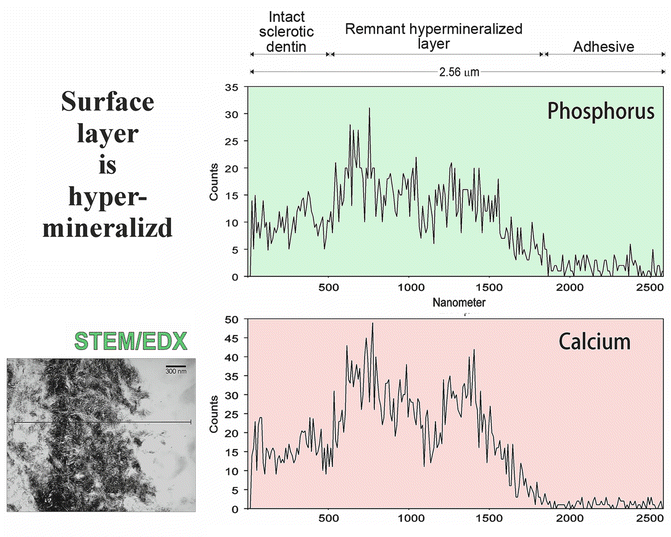
Fig. 10.7
Bright-field STEM image showing the location of a line scan that was performed across the surface hypermineralized layer of a noncarious cervical sclerotic lesion. Qualitative energy dispersive line scans showing the distribution of phosphorus (upper scan) and calcium (lower scan) along the adhesive, the surface hypermineralized layer of the lesion, and the underlying intact sclerotic dentin (Modified from Tay and Pashley (2004a))
Quantitative energy dispersive X-ray spectra of the elemental content of crystallites present within (a) the surface hypermineralized layer, (b) the underlying intertubular dentin, and (c) within the dentinal tubules of the wedge-shaped defect are illustrated in Fig. 10.8 (Tay and Pashley 2004a). As the analysis was performed using 70 nm thick undemineralized sections, this enabled estimation of the calcium/phosphate ratio of these crystallites without additional ZAF correction (Karan et al. 2009). The Ca/P ratios of the crystallites within the hypermineralized layer and the underlying dentin approach the theoretical value of 1.67 calculated for hydroxyapatite (Daculsi et al. 1979). The larger apatite crystallites observed in the surface hypermineralized layer are similar to larger apatite crystallites reported in remineralized carious dentin (Daculsi et al. 1979) and cementum (Tjäderhane et al. 2013b). Conversely, the Ca/P ratio of crystallites from the sclerotic casts within the dentinal tubules is slightly lower than the calculated value of 1.50 for tricalcium phosphate (Daculsi et al. 1979). The additional presence of about 5 % magnesium suggests that these crystallites are whitlockite (Mg-substituted β-tricalcium phosphate). All trivalent phosphate solids should be regarded as solid buffer that is difficult to solubilize. A recent microRaman study of the mineral distribution of noncarious, sclerotic cervical lesions came to similar conclusions (Karan et al. 2009).
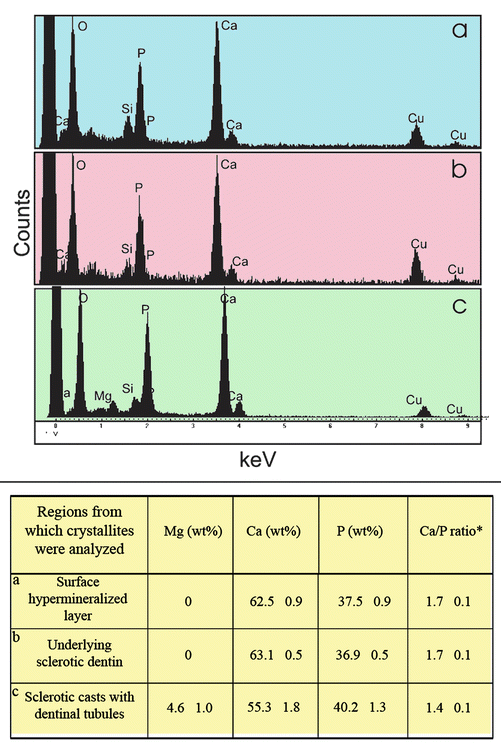
Fig. 10.8
Energy spectra from different locations of a noncarious cervical sclerotic lesion. (a) Spectrum showing composition of crystallites within the surface hypermineralized layer. (b) Spectrum of crystallites within the underlying intact sclerotic dentin. (c) Spectrum of crystallites occupying the lumen of a dentinal tubule. Spectra were obtained using a 7 nm spot for 200 live seconds at 200 kV. The relative concentration of Ca, P, and Mg and the calculated Ca/P ratios are shown in the table beneath the spectra (Modified from Tay and Pashley (2004a))
10.3.5 Status of the Collagen Fibrils Within the Surface Hypermineralized Layer
An important question that has remained unanswered is whether the surface hypermineralized layer is devoid of collagen (Duke and Lindemuth 1990). Using special staining for collagen, it can be seen that the supporting matrix for the crystallites within the hypermineralized layer consists of a bed of denatured collagen (Fig. 10.9a). The transition from denatured collagen (gelatin) to intact collagen with crossbanding is evident at the base of the hypermineralized layer, where some of the collagen fibrils are observed to unravel into microfibrillar subunits (Tay and Pashley 2004a). This transition from banded collagen to denatured microfibrils is further illustrated beneath a layer of bacteria in Fig. 10.9b, in which unraveling of collagen fibrils created a network of microfibrillar strands that no longer showed crossbanding.
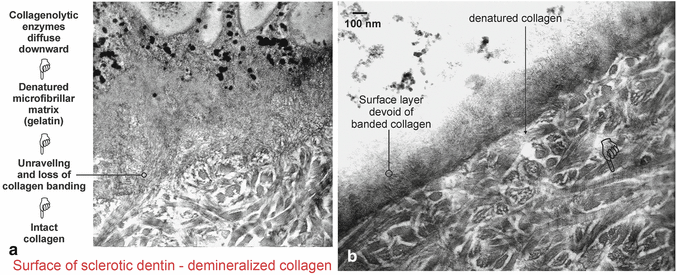
Fig. 10.9
(a) TEM micrograph from a demineralized specimen, showing that the supporting matrix of the hypermineralized layer (top half of image) consisted of a bed of denatured collagen microfibrils. These subunits of collagen were formed by unraveling of collagen fibrils (circle). Within the underlying sclerotic dentin, intact larger banded collagen fibrils could be seen. (b) A thin hypermineralized layer showing the transition from intact, banded collagen fibrils (pointer) into denatured collagen (gelatin), appearing ultrastructurally as microfibrillar strands
It is possible that colonization of bacteria along the surface of the wedge-shaped defect results in the production of acidic by-products that demineralize dentin collagen fibrils (Fig. 10.9a, b). It is now thought that bacterially derived organic acids expose and activate previously inactive proforms of the endogenous matrix metalloproteinases of dentin (Pashley et al. 2004; Breschi et al. 2008; Tjäderhane et al. 2013a, b). The loss of phosphoproteins (Brackett et al. 2010) and subsequent remineralization of the surface bed of denatured collagen under the possible influence of piezoelectric charges generated from eccentric flexural deformation (Spranger 1995) may result in the characteristic orientation of the crystallites within the denatured microfibrillar matrix of the hypermineralized layer.
10.3.6 Summary of the Microstructural Changes in Noncarious Sclerotic Cervical Dentin
Sclerotic dentin is an abnormal bonding substrate that exhibits a high degree of variability both in terms of occlusion of the dentinal tubules as well as the thickness of the surface hypermineralized layer. The latter, in particular, is invariably associated with bacteria. It is possible that bacteria are involved in the pathogenesis of the hypermineralized layer. That is, dentin may first require demineralization before it can be hypermineralized. Also, mineral crystallites cannot accumulate without a scaffold. The presence of bacteria, apart from demineralizing the dentin, also denatures the existing collagen matrix, resulting in a bed of denatured collagen. This may act as a scaffold upon which the demineralized dentin may be subsequently remineralized. The formation of the hypermineralized layer is probably also enhanced by the presence of high concentrations (10 ppm) of fluoride ions (Perdigão et al. 1994; Goncalves et al. 1999; Pashley and Carvalho 1997). As denaturing of the collagen fibrils probably removes some of the restrictions on the size of crystallite formation, this may enable larger crystallites to be formed during the process of remineralization. The unique arrangement of the crystallites further suggests the complex role of parafunctional stress on the formation of the surface hypermineralized layer in these natural wedge-shaped lesions (Rees 1998; Gwinnett and Jendresen 1978). The presence of bacteria, mineralized bacterial matrices, hypermineralized surfaces, and dentinal tubules occluded with mineralized crystals makes sclerotic cervical dentin a unique multilayered bonding substrate. This implies that bonding studies which attempt to generate hypermineralized dentin in vitro by immersing partially demineralized dentin in a remineralizing solution containing a high fluoride content (Perdigão 2002; Van Meerbeek et al. 2003) do not simulate the actual bonding conditions that clinicians are likely to encounter when bonding to noncarious cervical sclerotic dentin.
10.4 Bonding Adhesive Resins to Sclerotic Dentin
Current dentin adhesives employ two different means to achieve the goal of micromechanical retention between resin and dentin (Nakabayashi et al. 1982; Gwinnett and Kanca 1992b; Kanca 1992). The first method, the total-etch or etch-and-rinse technique, attempts to remove the smear layer completely via acid etching and rinsing. The second approach, the self-etching technique, aims at demineralizing and incorporating the smear layer as a bonding substrate.
10.4.1 Total-Etch Technique
Most self-priming, single-bottle adhesives available to date attempt to bond to dentin that is etched with 37 % phosphoric acid. Following rinsing of the conditioners with water, retention is accomplished by means of resin infiltration into the exposed, demineralized collagen matrix to form a hybrid layer of resin-impregnated dentin (Gwinnett and Kanca 1992b; Kanca 1992; Gwinnett 1994). Systems containing hydrophilic primer resins solvated in acetone or ethanol were found to produce higher bond strengths when acid-conditioned dentin was left visibly moist prior to bonding (Titley et al. 1994). This technique is often referred to as “wet bonding” (Pashley et al. 2000). The benefit of wet bonding stems from the ability of water to keep the interfibrillar channels within the collagen network from collapsing during resin infiltration (Pashley et al. 2003; Tao et al. 1988). These channels, which are about 20 nm wide when fully extended (Fig. 10.10), must be maintained open to facilitate optimal diffusion of resin monomers into the demineralized intertubular dentin (Eick et al. 1991; Watanabe et al. 1990).
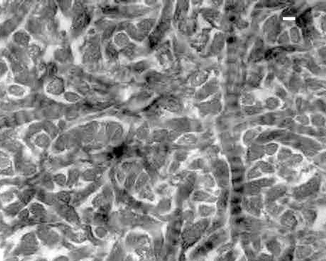
Fig. 10.10
Dentin collagen fibrils from a hybrid layer in normal dentin that was produced using a total-etch technique. Randomly oriented interfibrillar channels must be maintained open for optimal acid etching and resin infiltration. This is achieved using the wet-bonding technique. Bonded with One-Step (Bisco). Bar = 50 nm
10.4.2 Self-Etching Technique
Self-etching techniques in dentin bonding have reintroduced the concept of utilizing the smear layer as a bonding substrate, but with improved formulations that could etch through the smear layer and beyond, into the underlying dentin matrix. Failure to etch beyond the smear layer, exemplified by some of the early adhesives that were applied directly to the smear layer, resulted in weak bonds due to the complete absence of a hybrid layer in underlying intertubular dentin (Chigira et al. 1994; Finger and Balkenhol 1999). Contemporary self-etching adhesives have been developed by replacing the separate acid-conditioning step with increased concentrations of acidic resin monomers in a primer. Two-step self-etching primers combine etching and priming into a single step. The primed surfaces are subsequently covered with a more hydrophobic, solvent-free adhesive layer that is light cured. In the presence of water as an ionizing medium, these adhesives etch through smear layers and bond to the underlying intact dentin (Fig. 10.11) (Peschke et al. 2000; Ferrari and Tay 2003). The recent introduction of single-step, single-bottle, self-etching adhesives represents a further reduction in bonding steps that eliminates some of the technique sensitivity and practitioner variability that are associated with the use of total-etch adhesives (Watanabe et al. 1994; Nakabayashi and Saimi 1996; Sano et al. 1999; Tay and Pashley 2001).
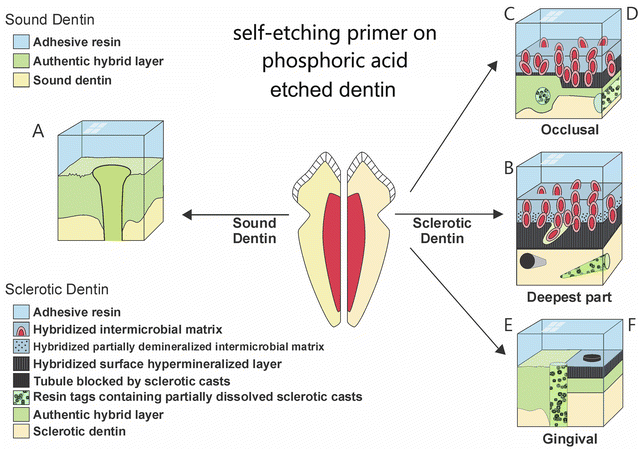
Fig. 10.11
Schematic showing the ultrastructural differences seen when bonding normal cervical dentin with 40 % phosphoric acid (a) versus bonding to wedge-shaped cervical sclerotic lesions preconditioned with 40 % phosphoric acid and then bonded with a mild self-etching primer/adhesive to (c) the occlusal wall of specimen with thin hypermineralized layer or (d) thicker hypermineralized layer or (b) to the deepest part of the wedge-shaped lesion or (e) to the gingival with or without a hypermineralized layer or (f) with a hypermineralized layer
When applied to sound dentin, the milder self-etching adhesives produce a hybridized complex that consists of a surface zone of hybridized smear layer and a thin, subsurface hybrid layer in the underlying intertubular dentin (Prati et al. 1998; Yoshiyama et al. 1998; Pereira et al. 1999). Despite the presence of a hybrid layer that was generally less than 2 μm thick, high initial bond strengths have been reported for sound dentin (Tay et al. 2000a; Ogata et al. 2002; Toida et al. 1995; Igarashi et al. 1997). The more aggressive self-etching adhesives completely dissolve smear plugs and demineralize dentin to the extent that is comparable with phosphoric acid etching (Pereira et al. 1999). There has been some concern that mild self-etching adhesives may not be able to penetrate through thick smear layers, such as those produced clinically by rough diamond burs (Prati et al. 1998; Yoshiyama et al. 1998; Pereira et al. 1999; Tay et al. 2000a; Ogata et al. 2002; Toida et al. 1995; Igarashi et al. 1997; Miyasaka and Nakabayashi 1999). The adjunctive use of phosphoric acid preconditioning has been suggested as a means to improve bonding of self-etching primers to sound dentin with thick smear layers (Tay et al. 2000b; Eick et al. 1997; Gwinnett et al. 1996). Ground dentin surfaces are often produced clinically and have been shown to improve bond strength (Coli et al. 1999).
10.4.3 Problems in Bonding to Sclerotic Dentin
Regardless of the use of a total-etch or a self-etching technique, bonding to pathologically altered substrates such as sclerotic dentin from noncarious cervical lesions generally leads to compromised bonding (Yoshiyama et al. 1996b; Prati et al. 1999; Kwong et al. 2002; Daculsi et al. 1979). Reduced bonding efficacy was attributed to a combination of factors that included the obliteration of dentinal tubules with sclerotic casts, the presence of an acid-resistant hypermineralized layer, and the presence of bacteria on the lesion surface. The presence of the hypermineralized layer, bacteria, and tubular mineral casts in sclerotic dentin is analogous to the presence of the smear layer and smear plugs in sound dentin, being potential diffusion barriers for primer and resin infiltration. The concern that a self-etching primer may not etch through the superficial layers on sound dentin may likewise be applicable to sclerotic dentin. Phosphoric acid preconditioning prior to the application of a self-etching primer may thus be a useful technique when bonding to sclerotic dentin (Fig. 10.12a) (Kwong et al. 2002).
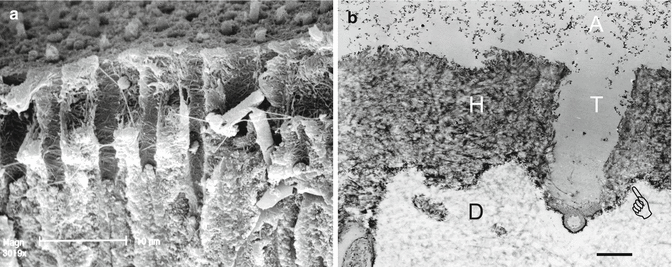
Fig. 10.12
(a) Sclerotic dentinal tubules that were filled with mineralized casts which block resin infiltration, after etching with 37 % phosphoric acid to solubilize those casts, opening the tubules for resin tag formation. (b) Combined action of 40 % phosphoric acid to demineralize hypermineralized dentin more deeply than can a self-etching adhesive, followed by a water rinse and application of a self-etching adhesive, creates well-primed thick hybrid layers in sclerotic dentin. The smear layer was completely removed. The hybrid layer (H) was about 4 μm thick. Pointer: base of hybrid layer. A: filled adhesive containing nanofillers (Modified from Tay and Pashley (2004a))
10.4.4 Obstacles in Bonding to Acid-Etched, Sound Dentin
Adhesive strategies that rely mostly on micromechanical retention are hampered by obstacles that jeopardize infiltration of resin into dental tissues (Hashimoto et al. 2002). In abraded sound dentin, the smear layer is effectively removed in adhesive systems that utilize a separate acid-conditioning and rinsing step. In order to achieve optimal resin infiltration, acid-etched demineralized dentin must be suspended in water to prevent collapsing of the interfibrillar spaces. This is effectively accomplished using the wet-bonding technique. Interfacial strength is dependent upon the ability of resins to engage the deepest extent of demineralized intertubular dentin (Wang and Spencer 2002, 2003; Vasiliadis et al. 1983; Vargas et al. 1997; Inai et al. 1998). The collagen matrix may thus be viewed as a diffusion barrier or obstacle for resin infiltration in acid-etched dentin.
10.4.5 Obstacles in Bonding to Acid-Etched Sclerotic Dentin
Unlike sound dentin, application of the same adhesive strategy to sclerotic dentin results in substantial variation in both hybrid layer and resin tag morphology. Potential obstacles of resin infiltration into uninstrumented natural lesions include the hypermineralized surface layer, an additional partially mineralized surface bacterial layer, and intratubular mineral casts which are comparatively more acid resistant (Gwinnett and Jendresen 1978; Van Meerbeek et al. 1994; Kwong et al. 2000). As these inclusions vary considerably along the occlusal, gingival, and the deepest part of a wedge-shaped lesion, variation in the ultrastructure of the resin-sclerotic dentin interfaces are possible in these different regions. While it is reasonable to assume that the extent of tubular occlusion (Fig. 10.12a) would vary according to the severity of dentin sclerosis (Tay et al. 2002), both the superficial bacterial and hypermineralized layers are found to vary from site to site, being thicker along the deepest part of the wedge-shaped lesions (Kwong et al. 2002). The surface hypermineralized layer was usually thinner in gingival and occlusal surfaces than in the apical or deepest part of the wedge-shaped defects and was often partially or completely dissolved when phosphoric acid was applied to sclerotic dentin. As a result, the thickness of the hybrid layers in the gingival and occlusal sites was similar to those seen in acid-etched sound dentin and remained fairly consistent at about 5 μm (Fig. 10.12b). Bacteria, if present, tended to be tenaciously attached to the dentin surfaces and in the dentinal tubules and were retained even after rinsing.
Thicker diffusion barriers are found within the deepest part of wedge-shaped lesions, which hamper the penetration of acids through the underlying intact sclerotic dentin. As a result, alterations in hybrid layer morphology and thickness are seen in these regions. One example is shown in Fig. 10.13a. The surface hypermineralized layer from the deepest part of a wedge-shaped lesion was about 3 μm thick and was trapped within the resin-sclerotic dentin interface. The phosphoric acid apparently etched through the hypermineralized layer and created a hybrid layer of about 2 μm thick within the underlying intact sclerotic dentin. Rhombohedral whitlockite crystallites from the sclerotic casts may also be identified within dentinal tubules in the underlying sclerotic dentin. Figure 10.13b shows a specimen where the hypermineralized surface layer was destroyed. Bacteria remain on the surface and within tubules within the hybrid layer (Hd).
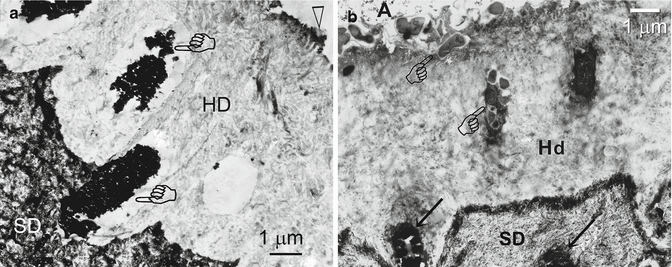
Fig. 10.13
(a) Application of the etch-and-rinse adhesive One-Step (Bisco) to 37 % phosphoric acid-etched sclerotic dentin (SD). This stained undemineralized section was taken from the gingival surface of a wedge-shaped lesion. Remnants of a very thin, electron-dense, hypermineralized layer (arrowhead) could be seen on the surface of the hybrid layer. Some tubules remain occluded with sclerotic casts (pointers) that became surrounded by resin tags (pointers) (Modified from Tay and Pashley (2004a)). (b) Stained, undemineralized TEM section showing application of a filled adhesive to the occlusal surface of a phosphoric acid-etched, sclerotic lesion. Bacteria (pointers) were trapped in the hybrid layer (Hd) surface and in the tubules. Other tubules were filled with sclerotic casts (arrows) (Modified from Tay and Pashley (2004a))
Figure 10.14 is a stained, demineralized TEM micrograph taken from the deepest part of another acid-etched, wedge-shaped natural sclerotic lesion. Within the 50 μm wide region of the micrograph, the thickness of the hybrid layer in sclerotic dentin changed abruptly from 2 μm to 5 μm. This aspect of uneven etching may also be seen in areas in which the acid etched laterally within the subsurface sclerotic dentin, producing lateral hybrid layer extensions which are separated from areas above that are not infiltrated with resin. This feature is distinct from incompletely resin-infiltrated hybrid layers that are observed in air-dried, acid-etched sound dentin. These “eccentric” hybrid layers in abnormal dentin substrates have never been observed in bonded sound dentin.
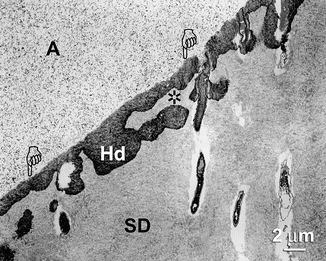
Fig. 10.14
Undemineralized section taken from the deepest part of a wedge-shaped natural sclerotic lesion that was etched with 40 % phosphoric acid and bonded using self-etching primer/adhesive. Hd: hybridized hypermineralized layer that varied in thickness from 2 μm (pointers) to 5 μm (Hd). A adhesive containing nanofillers. A lateral extension of the hybrid layer could be seen below an area that was not infiltrated with adhesive resin (asterisk). A adhesive, SD sclerotic dentin (Reprinted from Tay and Pashley 2004b, with permission from Elsevier)
Unlike sound dentin in which the morphology of the hybrid layer remains fairly consistent as long as a wet-bonding technique is used, extensive variations are found in different specimens or locations within cervical sclerotic lesions. Figure 10.15 is a demineralized, stained TEM micrograph taken from the deepest part of a natural lesion. Whereas a thin hypermineralized layer and the presence of bacteria did not prevent the penetration of acid or resin into the underlying sclerotic dentin, the thickness of the hybrid layer was greatly reduced in the presence of a thick hypermineralized layer. These thick hypermineralized layers probably serve as diffuse barriers and prevent the penetration of both phosphoric acid and adhesive monomers. Reduced hybrid layer thickness may have no correlation with reduced regional microtensile bond strength in natural sclerotic dentin (Yoshiyama et al. 1996b; Kwong et al. 2002; Daculsi et al. 1979). However, the presence of thin hybrid layers should clearly be differentiated from the total absence of hybrid layer formation in the bonding substrate. Under such circumstances, the adhesive will be bonding directly to the diffusion barriers that impede acid etching. The resultant bond strength will depend on the strength of the attachment of such obstacles to the underlying sclerotic dentin. It is remarkable that these morphological fluctuations are continuous and vary within a very small region of a lesion that is covered by these micrographs. Such extreme variations in hybrid layer morphology are probably responsible for the large standard deviations in microtensile bond strength measurements of bonding to sclerotic dentin. It is likely that these segregated areas of deficient hybrid layer formation act as weak links, or stress raisers, that contribute to the initiation of adhesive failures in bonded sclerotic dentin.
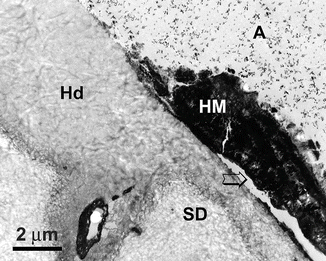
Fig. 10.15
Undemineralized TEM micrograph from the deepest part of a phosphoric acid-etched, natural sclerotic lesion. The hybrid layer (Hd) in intact sclerotic dentin (SD) was 5 μm thick when there was no overlying hypermineralized layer. This thickness was reduced to 2 μm thick beneath the hypermineralized layer (HM). The separation of the hypermineralized layer (arrow) was an artifact produced during specimen processing. A filled adhesive, SD sclerotic dentin (Reprinted from Tay and Pashley 2004b, with permission from Elsevier)
10.4.6 Obstacles to Bonding in Sound Dentin Treated with a Self-Etching Primer Alone
The use of two-step and single-step self-etching adhesives represents an alternative means to acquire micromechanical retention in dentin. They are attractive in that they may be used on dry dentin and, after mixing, require only one primer application, which is subsequently air-dried rather than rinsed. The latest single-step self-etching adhesives further incorporate all the resin monomers, photoinitiator, and tertiary amine accelerator into a single bottle and eliminate an additional mixing step. Despite the physical appearance of thin hybrid layers and short, hybridized smear plugs in a two-step self-etching adhesive (Fig. 10.16), high initial bond strength have been reported. This suggests that there is no correlation between hybrid layer thickness and bond strength as long as a uniform demineralization front is created in sound intertubular dentin (Ogata et al. 2002; Wang and Spencer 2002; Pashley et al. 1988, 1992).
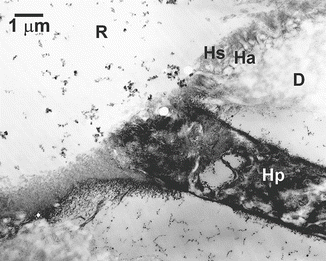
Fig. 10.16
Demineralized TEM micrograph of the application of a self-etching primer/adhesive to sound normal dentin (artificial wedge-shaped lesion). To achieve effective bonding, a self-etching primer must not only create a hybridized smear layer (Hs) but also an authentic hybrid layer in the underlying intact sound dentin (Ha). The dentinal tubule was sealed by a hybridized smear plug (Hp). R adhesive resin, D sound dentin
There was concern, in normal dentin, that thick and rough smear layers may interfere with diffusion of self-etching primers into the underlying intact dentin. This may be due to the physical presence of thick smear layers as a diffusion barrier, or their ability to buffer the acidic monomers, making the pH too high to demineralize the underlying intertubular dentin. Recent studies showed that mildly aggressive self-etching adhesives penetrated through smear layers up to 3–4 μm thick and still retained sufficient acidity to demineralize the underlying intertubular dentin to a depth of 0.4–0.5 μm (Yu et al. 1990). This suggests that either the buffering capacity of the smear layer is weak or the smear layer does not impose much of a physical barrier to the primer compared with the underlying mineralized dentin matrix. The looseness of the surface portion of the smear layer and/or the presence of diffusion channels between its constituents may facilitate diffusion of the self-etching primer through the smear layer (Ferrari et al. 1996). Disaggregation of the smear layer into globular subunits (Gwinnett 1993) further provides micro-channels for diffusion of the self-etching adhesives. These micro-channels, in theory, should be more permeable to resin monomers than the interfibrillar spaces (ca. 20 nm) of demineralized intertubular dentin.
10.4.7 Obstacles in Bonding to Self-Etching Primer Treated Sclerotic Dentin
The smear layers in sound, cut dentin do not impose much of a restriction to the bonding of contemporary self-etching adhesives because of their loose organization. Unless they are instrumented, shiny sclerotic cervical lesions are free of smear layers. In lieu of smear layers, other diffusion barriers in shiny cervical sclerotic lesions include the much denser surface hypermineralized layer, as well as the more loosely arranged, partially mineralized bacterial biofilms. Some of the hypermineralized layers in sclerotic dentin are so thick that they restrict the penetration of strong inorganic acids such as phosphoric acid that usually etches 5 μm or beyond into sound dentin. Being less acidic in nature, mild self-etching primers only etch 0.5 μm into sound dentin that is covered with smear layers. As sclerotic dentin is highly variable in its ultrastructure, different morphologic expressions of the resin-dentin interfaces can be anticipated when a mild self-etching primer is applied to this abnormal bonding substrate. This may be classified into three categories, depending on the thickness and continuity of the surface hypermineralized layer at different locations of the wedge-shaped lesion.
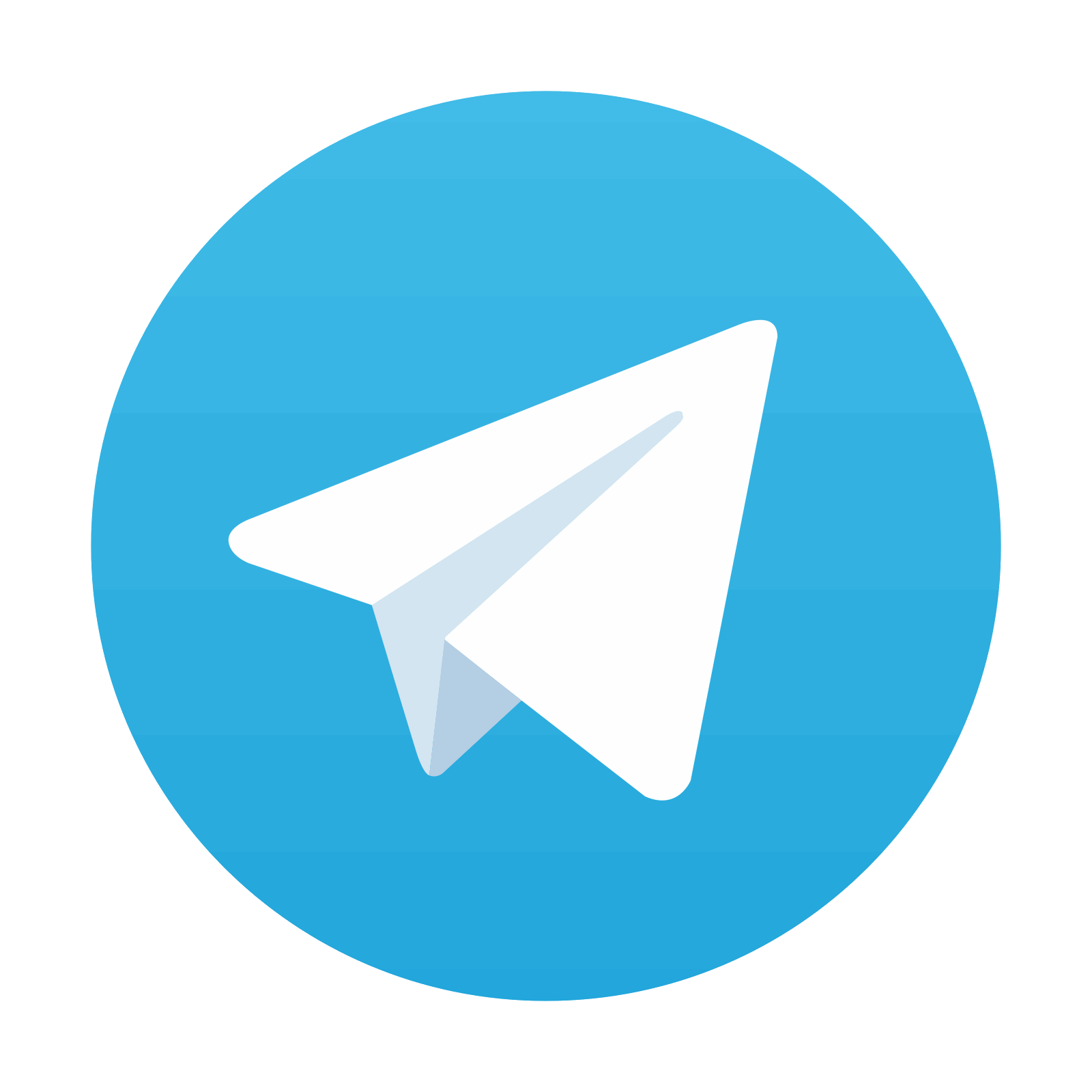
Stay updated, free dental videos. Join our Telegram channel

VIDEdental - Online dental courses
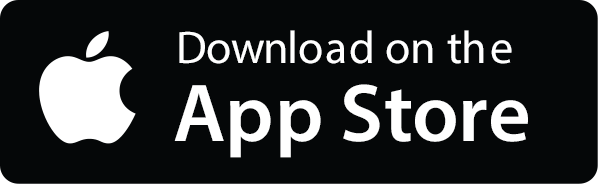
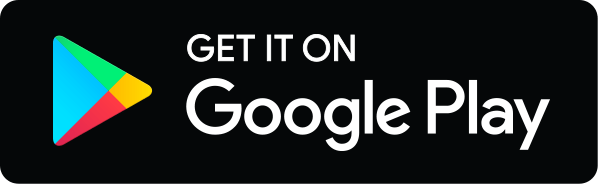