Fig. 2.1
Principal virulence factors assisting in the survival of pathogenic Candida
Adhesion and Colonization
Prior to any signs of overt infection, Candida species must first undergo adhesion and colonization to either host cells or an abiotic substrate. After the initial adhesion, colonization is established, which subsequently may lead to a diseased state (Kumamoto 2002). Several factors have been suggested to influence Candida adhesion, primarily adhesion proteins (Mayer et al., 2013). The adhesive proteins of C. albicans have been intensely studied and the agglutinin-like sequence (ALS) proteins have been identified as the key players. There are eight ALS proteins known, (ALS 1-7 and ALS9) and of these ALS3 has been isolated as the most important due to its vast upregulation during infection and ability to bind cadherins on host cells and induce endocytosis of the pathogen (Murciano et al., 2012; Phan et al., 2007) (Fig. 2.2). Another essential protein is Hwp1, Hyphal-associated GPI-linked protein; this adhesin induces a covalent bond between hyphae and the host cell as Hwp1 is the substrate for transglutaminases. The evidence for the leading role these proteins play comes from studies using knock out (KO) mouse models, which demonstrated reduced infection in models of systemic candidiasis (Sundstrom et al., 2002; Phan et al., 2007). As for C. albicans invasive abilities, two invasins are known, Ssa1, a member of the HSP70 family, and the previously mentioned Als3, both bind to E-cadherin on host cells and consequently induce endocytosis (Sun et al., 2010; Phan et al., 2007). C. glabrata has lower adherence capacity to gingival cells when compared to C. albicans and C. tropicalis, and its adhesive properties are under the control of the epithelial adhesion (EPA) family of genes. The C. glabrata genome contains several EPA genes, though EPA1 has been shown to play a significant role in adhesion as only EPA1 KO strains have shown reduced adherence (De Las Penas et al., 2003; Cormack et al., 1999). Of note, C. glabrata adhere to dentures at a twofold greater rate than C. albicans, suggesting that this species have a stronger affinity for binding to prosthetics materials; thus, a possible explanation for the increase in C. glabrata infection may simply be due to increased use of denture prosthesis, catheters, and ventilation tubes (Li et al., 2007). In relation to C. parapsilosis adhesion during infection, relatively few studies have been undertaken; however, five ALS genes and six predicted glycophosphatidylinositol-anchored proteins 30 (Pga30) have been identified (Butler et al., 2009). As for C. tropicalis, it is known to adhere well to human cells and abiotic surfaces and three ALS genes have been recognized thus far. If and how these genes contribute to adhesion is yet to be investigated (Hoyer et al., 2001). Therefore, the knowledge gained from these studies helps to piece together the infection process, giving a better understanding of how subsequent colonization and biofilm formation occurs.
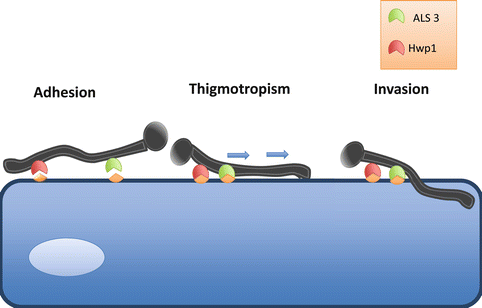
Fig. 2.2
Key stages in adhesion and tissue penetration. Candida adheres to epithelial cells via interactions of specific adhesin proteins, such as ALS3 and HWP1, to cellular cadherins. Once adhered, thigmotropism occurs, in which directional hyphal growth leads the hyphae toward weakened areas of the cell. Release of hydrolytic enzymes then further facilitates invasion of the epithelial cell and liberation of nutrients
The attachment of fungal cells is closely followed by cell division, proliferation, and the development of a biofilm (Kumamoto 2002). The capacity of some Candida species to form biofilms is classed as a virulence factor; a biofilm is defined as a complex structured microbial community enclosed in an extracellular matrix (ECM) and it is now believed that the majority of microorganisms utilize this form of growth. The encased structure of the mature biofilm provides protection by preventing the penetration of host immune factors and antifungals into the ECM when compared to planktonic cells (Ramage et al., 2009). C. albicans, C. glabrata, C. parapsilosis, and C. tropicalis all have the ability to form biofilms and have been associated with higher levels of morbidity than that of non-biofilm forming Candida species (Kumamoto 2002). Strong evidence suggests the hyphal production of Candida species is necessary to form the stable 3D architecture characteristic of mature biofilms (Ganguly and Mitchell 2011). Nonetheless, the need for hyphae to form a biofilm remains controversial as C. glabrata is unable to form hyphae, yet remains the second most commonly isolated Candida species (Li et al., 2007). Furthermore, there is a lack of experimental evidence for C. glabrata biofilms, despite the increasing number of clinical isolates. Of note, C. glabrata is rarely isolated on its own, as it is generally found with other Candida species, primarily C. albicans (Coco et al., 2008). In cases of severely inflamed denture stomatitis, C. glabrata and C. albicans were co-isolated in 80 % of cases. Therefore, it has been suggested that the C. albicans biofilm supports the growth of C. glabrata and acts as a scaffold to maintain structural integrity (El-Azizi et al., 2004; Ramage et al., 2009).
Gene expression is under the control of transcription factors, and these control the upregulation or downregulation of their target genes. Six transcription factors have been identified as the core regulators involved in biofilm formation in C. albicans, Egf1, Bcr1, Brg1, Rob1, Ndt80, and Tec1 (Nobile et al., 2012). All of these resulted in defective biofilm formation in vitro and in two in vivo animal models when these genes were deleted (Nobile et al., 2012). The ECM composition of C. albicans consists of carbohydrates, mainly β-1,3 glucan, proteins, phosphorus, and hexoamines. Positive regulators of β-1,3 glucan such as Glucoamylases (Gca1 and Gca2), glucan transferases (Bgl2 and Phr1), and exo-glucanase Xog1 play an integral role in protecting the fungal cells as the biofilm becomes more susceptible to antifungals when they are absent (Taff et al., 2012). As for NCAC species, their ECM matrix composition has been understudied; however, it is known that C. tropicalis main ECM component is hexosamine and therefore has a lower protein and carbohydrate concentration when compared to other NCAC species (Silva et al., 2009a). Once a mature biofilm is fully established, yeast cells can then disseminate out to other areas, subsequently leading to the spread of infection (Fig. 2.3). Therefore, the formation of a biofilm is a fundamental mechanism exerted by Candida species, which aids their success as a pathogen by providing a protective niche for these fungi to grow, proliferate, and subsequently disperse whilst defending against potentially devastating assaults from the immune system.
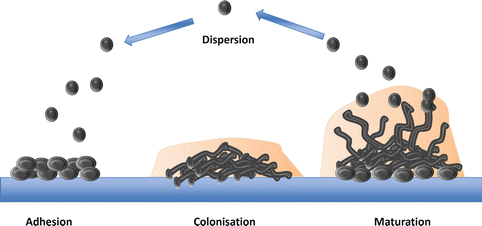
Fig. 2.3
Developmental phases of biofilm formation. The attachment of fungal cells is closely followed by cell division and proliferation, thus establishing colonization. The production of hyphal growth and ECM leads to mature stable biofilm architecture. The mature biofilm then disperses yeast cells, subsequently leading to the formation of a new colonies and further biofilm development
Although the role of hyphae within biofilms remains controversial, particularly with C. glabrata, overwhelming evidence indicates that it plays an integral role of forming the stable 3D architecture.
Filamentation
The genus Candida is a group that can grow as several distinct morphologies. The one morphological form in common is the rounded yeast form, although the texture of the colonies differs depending on the species (Larone 2002b). Hyphae are formed as a germ-tube projection from the original yeast daughter cell, forming branches, which are divided by septa into separate fungal units. Pseudohyphae can also form by budding from the original yeast cell, but which fail to detach and thus extend outward into true hyphae (Silva et al., 2012). Those Candida species that can grow as hyphae or pseudohyphae are considered as being more virulent (Jacobsen et al., 2012). This is attributed to its ability to penetrate into mammalian cells more easily than in the yeast form, particularly epithelial cells, which act as a primary barrier for innate immunity. Figure 2.4 illustrates the different morphological forms of Candida species.
It is thought that the more effective infiltration of epithelial cells by the filamentous morphologies is due to the pressure generated by the hyphal tip (Gow et al., 2002). The mechanics behind the generation of tip pressure remain little understood; however, it is evident from studies on plant fungal pathogens, that the cell must be sufficiently adhered to the surface to generate the pressure (Brand 2012). This gives explanation as to why genes responsible for adhesion are rapidly upregulated during morphogenesis. The tip is also the site of enzyme secretion and other degrading substances to weaken the cell wall (Hube and Naglik 2001). It has been suggested that the penetrative nature of Candida is intrinsic and that these organisms have been programmed to infiltrate any surface they contact. Hyphae are able to penetrate into silicone material that biofilms are grown on, even despite the absence of any biological interactions with the surface (Leonhard et al., 2010). The directionality of hyphal tip growth is responsive to the surrounding environment, allowing the tip to direct its way around obstructions or toward essential nutrients (Gow et al., 1994). Additionally, thigmotropism (directional hyphal growth) can occur due to contact sensing with the cell surface and can lead the hyphae to weakened areas of the cell wall (Hube and Naglik 2001; Gow et al., 1994). Studies on thigmotropism in human pathogenic fungi are limited; however, characteristic thigmotropic behavior has been demonstrated in C. albicans and C. dubliniensis (Watts et al., 1998; Chen et al., 2011). Control over directionality is important in the initial stages of tissue invasion as a C. albicans mutant of the rsr1 gene, which is involved in hyphal directional growth, saw growth become erratic and its invasive abilities decreased by 50 % (Brand et al., 2008). However, once the initial layer of cells has been invaded, control over directional growth is no longer required to cause cell damage (Wachtler et al., 2011). Furthermore, filamentous growth has been suggested as a mechanism for avoiding phagocytosis, with in vitro experiments demonstrating hyphal outgrowth within macrophages leading to puncturing and killing of these cells, though this has yet to be proven in vivo (Cutler 1991).
C. albicans being the most widely studied is known to be truly polymorphic taking on yeast, hyphae, and pseudohyphae forms; this is also true for C. dubliniensis (Silva et al., 2012). C. glabrata however can only grow as yeast, yet remains to be the second or third most commonly isolated species in candidiasis after C. albicans; this is likely a result of the strong antifungal resistance of this species (Li et al., 2007). On the other hand, C. parapsilosis fails to generate hyphae but can produce pseudohyphae known as “giant cells” due to their large curved appearance (Trofa et al., 2008). As for C. tropicalis, it is found as blastoconidia, pseudohyphae, and may also be found as true hyphae (Larone 2002a; Okawa and Goto 2006). Candida morphology is heavily influenced by the environment; for example, pH controls C. albicans morphology as pH (<6) grows as yeast and pH (>7) will induce hyphal growth and temperatures of 37 °C and CO2 also promote filamentous growth (Odds 1988; Sudbery 2011). Quorum sensing, a form of microbial communication, can sense cell densities and which in turn influences cell morphology, the primary quorum sensing molecules in C. albicans are farnesol and tyrosol (Albuquerque and Casadevall 2012). As for other NCAC species, there is relatively little evidence on the effect of morphology on the pathogenesis of these species; nonetheless, it is known that C. tropicalis can only invade oral epithelium in hyphal formations, only certain strains of C. parapsilosis can form hyphae and thus filamentous form was not a requirement to invade oral epithelium (Silva et al., 2009b; Albuquerque and Casadevall 2012; Silva et al., 2011). Perhaps the most prominent evidence for the importance of hyphae as a major virulence factor comes from mutants of C. albicans lacking the capacity to form hyphae as they exhibit lower ability to invade cells when compared to wild-type strains (Jayatilake et al., 2006). Thus the combination of hyphal growth, accompanied by thigmotropism, is a successful mechanism of infection. However, invasion is further optimized via the release of hydrolytic enzymes from the hyphal tip, which acts to weaken the cell membrane.
Hydrolytic Enzymes
Damage and penetration of the host epithelium is assisted by the secretion of hydrolytic enzymes in addition to the tip pressure generated by hyphae and pseudohyphae (Wachtler et al., 2012). Several groups of hydrolases are secreted by Candida species, proteases, phospholipases, and lipases (Mayer et al., 2013). Secreted aspartyl proteinases (SAP) function by disrupting the host membrane, allowing for pathogenic invasion. Ten SAP proteins have been identified, with some having a more prominent role in pathogenicity than others. SAP1-8 are secreted, whereas SAP9-10 remain bound to the fungal membrane (Naglik et al., 2003; Albrecht et al., 2006). It is well documented that SAP expression is significantly upregulated in C. albicans isolates from diseased individuals when compared to healthy controls; furthermore, biofilm formation has also been shown to positively correlate with SAP expression (Naglik et al., 2003). SAP1-3 have been shown to be responsible for the destruction of host epithelium in vitro, (Schaller et al., 1999), whereas SAP5-6 have been linked to hyphal formation and invasive candidal infection, with SAP5 in particular being associated with the early aggressive stage of biofilm formation (Hube et al., 1994; Ramage et al., 2012a). SAP8 is now emerging as a prominent player in candidal infection where it has been shown to be highly upregulated in mature biofilms, yet more evidence is required before a true role for SAP8 can be established (Ramage et al., 2012a). As for the role of SAP in NCAC species, proteinase secretion has been identified in C. glabrata, but the class of proteinase was not specified (Chakrabarti et al., 1991). On the other hand, three SAP genes have been identified in C. parapsilosis, but they have remained relatively unexplored (Merkerova et al., 2006). C. parapsilosis SAP expression appears to be both strain and environment dependant, as it exhibits stronger invasive abilities in skin and vaginal isolates in comparison to strains isolated from oral epithelium (Cassone et al., 1995; Dagdeviren et al., 2005). C. tropicalis possesses four SAP genes, named SAPT1-4, with only SAPT1 being well characterized thus far (Zaugg et al., 2001). SAPs secreted from invasive C. tropicalis isolates have been found on the fungal cell surface of those penetrating mucosal cells (Borg and Ruchel 1990). However, the role of SAP in invasion remains controversial as recent evidence from a mouse model of oral candidiasis suggests SAP1-6 are not essential causes for infection (Lermann and Morschhauser 2008; Correia et al. 2010). Nonetheless, the vast upregulation of these genes in diseased individuals when compared to healthy indicates they are playing a role in candidal pathogenicity (Hube et al., 1994; Naglik et al., 2008).
Phospholipase production is found in many Candida species, C. albicans produce several classes, which are separated into four groups, A-D, yet only certain members of class B are secreted extracellularly (PLB1-5), and play a role in virulence (Niewerth and Korting 2001; Mavor et al., 2005). Phospholipases hydrolyze phospholipids into fatty acids and thus cause damage to the host cell membrane and potentially exposing possible adhesion sites (Ghannoum 2000). Evidence for phospholipase secretion in NCAC species has been controversial with some studies reporting phospholipase activity in certain strains, whilst others detected no activity in the same strains (Ghannoum 2000; Kantarcioglu and Yucel 2002). C. tropicalis like C. albicans exhibits high phospholipase production but it is strain specific (Galan-Ladero et al., 2010; Negri et al., 2010), and as for C. glabrata, no studies have been conducted in reference to the presence of phospholipases.
Lipases are involved in the disruption of the cell membrane via the hydrolysis of triacylglycerols (Silva et al., 2012). Ten genes encoding lipases can be found in C. albicans LIP1-10, thus far only LIP8 has been proven to have a role in pathogenicity, as a LIP8 mutant showed attenuated virulence in a mouse infection model (Hube et al., 2000; Gacser et al., 2007). C. parapsilosis produced a less complicated biofilm when the lipase encoding genes CpLIP1/CpLIP2 were deleted and when a lipase inhibitor was applied; significantly less damage was done to the reconstituted human epithelium (Neugnot et al., 2002). Gene sequences closely related to those in C. albicans have been detected in C. tropicalis, but not C. glabrata (Fu et al., 1997), yet no studies have been conducted to investigate their role in virulence. The evidence for the role of lipases in candidal infection is promising, yet several genes still require exploration before these proteins can be said to be a major virulence factor for Candida species.
Stress Response Proteins
Stressful conditions such as starvation within living organisms can induce the heat shock response. Heat shock proteins (HSP) are released to prevent protein unfolding and aggregation, which if not prevented can lead to cell death. They act as chaperones by stabilizing essential proteins, thereby maintaining their form and function (Burnie et al., 2006). To date, six HSPs have been discovered within C. albicans: HSP90, HSP60, HSP104, HSP78, and two members of the HSP70 family; Ssa1 and Ssa2 (Mayer et al., 2013). HSP90 is found in all eukaryotes and many of its target protein function as cell-signalling components; however, in C. albicans, impaired HSP90 function leads to a temperature-dependant morphological switch from yeast to hyphae (Shapiro et al., 2012). This suggests that HSP90 is having a protective effect by preventing the transition to a more pathogenic morphology. Conversely, compromise of HSP90 induces resistance to azoles and echinocandins, transforming these usually fungistatic class of drugs into fungicidal (Singh et al., 2009; Cowen et al., 2009). Thus, HSP90 has a role in both protection and virulence. However, recent findings in which the depletion of HSP90 in C. albicans reduces virulence in a mouse model of systemic fungal infection suggests it is more implicated in pathogenicity (Shapiro et al., 2009). HSP60 is a mitochondrial HSP, of which the function is currently unknown but has been associated with a role in the regulation of elevated temperatures (Leach et al., 2011). As for HSP104 and HSP78, they are involved in biofilm formation and the response to phagocytosis by macrophages, respectively (Lorenz et al., 2004; Fiori et al., 2012). The two HSP70 members Ssa1 and Ssa2 are expressed on the surface of both yeast and hyphal forms (Li et al., 2006). Mutants of Ssa2 have indicated that this protein is dispensable for virulence; Ssa1 on the other hand acts as an invasin by binding surface proteins and directly infecting the oral epithelium, in a similar fashion as Als3 (Li et al., 2003, Sun et al., 2010).
Additional responses to environmental stress deployed by C. albicans include defence against reactive oxygen species (ROS) and reactive nitrogen species (RNS) released by phagocytes. Superoxide dimutases Sod1, Sod5, and catalase Cta1 protect against ROS; meanwhile, flavohemoglobin-related protein Yhb1 defends against RNS; deletion of any of these genes attenuates virulence in mouse models of systemic candidiasis (Brown 2012; Wysong et al., 1998; Hwang et al., 2002; Martchenko et al., 2004; Hromatka et al., 2005).
Antifungal Resistance
The classic treatment for oral candidiasis is antifungal therapy, but finding an effective antifungal is becoming increasingly problematic due to resistance. Resistance develops due to prolonged usage of antifungal drugs, which can have serious consequences, particularly in immunocompromised individuals, and is therefore viewed as an important virulence factor. Resistance can be an innate mechanism but is more commonly acquired by continued exposure to an antifungal where the target organism was previously susceptible.
Several classes of antifungal drugs are currently in use for treatment of candidiasis and are separated into groups depending on their molecular targets. Azoles interfere with membrane component ergosterol, and they target its biosynthesis pathway by inhibiting the enzyme lanosterol demethylase, causing loss of membrane fluidity and function and thus halting cell growth (Lewis et al., 2012). The low toxicity of these agents has led to its overuse in treating fungal infections, which has subsequently resulted in resistance (Pfaller and Diekema 2007). Mutations in the ergosterol biosynthesis pathway as well as the upregulation of efflux pumps are associated with azole resistance (Lupetti et al., 2002; Lewis et al., 2012), with around 20 % of C. glabrata strains developing resistance during therapy (Pfaller and Diekema 2007). Fluconazole and itraconozole are active against most Candida species with itraconozole showing activity against fluconazole-resistant strains (Pfaller and Diekema 2007; Pfaller et al., 2005). Voriconazole on the other hand acts on most Candida species, even those resistant C. albicans and C. glabrata strains; only C. tropicalis is less susceptible (Pfaller and Diekema 2007). Polyenes such as Amphoterecin B (AmpB) are highly fungicidal and directly target the ergosterol membrane components, forming pores, which destabilize the membrane, causing leakage of cellular contents (Lewis et al., 2012). AmpB has the broadest spectrum of antifungal activity and is therefore used to treat chronic fungal infections (Silva et al., 2012). Resistance to polyenes is rare, although in cases that do occur, it is generally a result of mutations in key members of the ergosterol biosynthesis pathway, which reduce the amount of ergosterol in the membrane. Mutations of enzyme sterol delta 5,6-denaturase (ERG3), which contribute to lowering antifungal susceptibility, are thought to be the primary cause (Chau et al., 2005). The most recent addition to the antifungal family are echinocandins, and these agents work by inhibiting β-1,3-D-glucan synthase, an enzyme essential for the synthesis of the key cell wall component β-1,3-D-glucan (Fig. 2.4) (Denning 2003). The echinocandins are active against C. albicans, C. glabrata, C. tropicalis but higher MIC values are required against C. parapsilosis (Pfaller et al., 2005). As this group of agents are relatively new, their use has not been widespread enough to determine if resistance against these antifungals will arise.
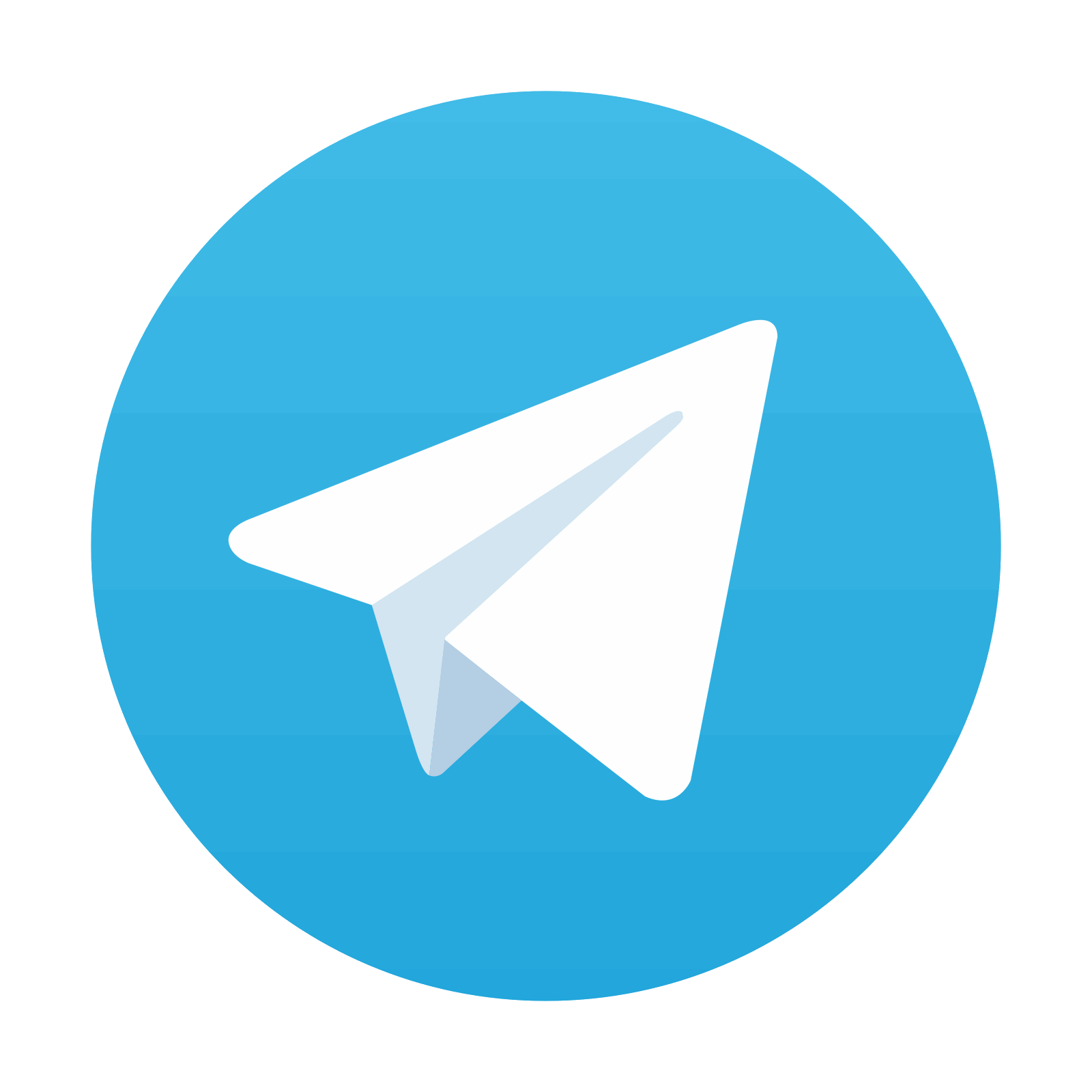
Stay updated, free dental videos. Join our Telegram channel

VIDEdental - Online dental courses
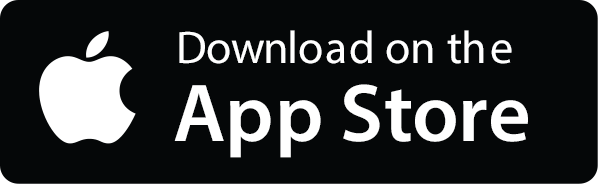
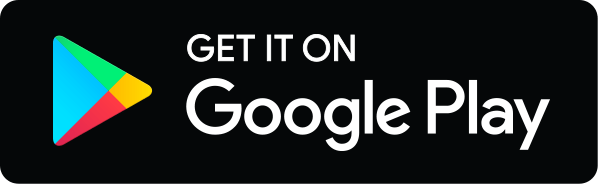