4
Calcium Phosphate and Bioactive Glasses
Introduction
The focus of biomaterials has been evolving in the last 50 years, leading to the design of bioactive materials. Bioactive material is defined as a material that has the potential effect on or inducing a response from living tissues, organisms or cells (Hench 1991; Salinas and Vallet-Regi 2007; Hench and Polak 2002).
Bone defects are among the most common diseases in clinical orthopedics and are mainly caused by infections, defects, tumors and congenital diseases. Generally, these defects need bone grafts since they cannot heal by themselves, and inappropriate treatment can lead to death or invalidity. Researchers have, therefore, tried to find novel materials for bone repair and substitution. Ceramic based materials are important sources of biomaterials for the biomedical field. The ceramics intended to be in contact with living tissues are called bioceramics (Hench 1991; Salinas and Vallet-Regi 2007). These types of bioceramics have been used to repair and replace diseased and damaged parts of musculoskeletal systems. Its biocompatibility varies from ceramic oxides, which are bioinerts in the body, to the other end of biodegradable bioactive materials that allow the stimulation of specific cellular responses at the molecular level like any other biomaterial. Bioceramics can be manufactured in porous or dense, amorphous or crystalline forms and applied as coatings, cements, scaffolds and nanoparticles (Yilmaz et al. 2019). The most widely used biodegradable bioactive ceramics include calcium phosphates (CaPs), they are the main constituents of bones, teeth and tendons of mammals, giving these organs hardness and stability (Supovd 2015). Biomineralization can be described as a phenomenon in which a mineral is integrated as a functional and often structural part of living organisms, often in direct and close contact to a matrix forming protein or carbohydrate structure. Among biominerals found in nature, the most common are calcium carbonate-based biominerals like aragonite (nacre) and calcite (mussels, exoskeletons of crayfish, etc.), CaPs (in vertebrate bone and teeth) and silicates (plants, sea sponges) but much rarer natural minerals also exist (Habraken et al. 2016). They can be produced in large quantities, against relatively low cost, are stable and therefore available off-the-shelf. Recent knowledge from the field of biomineralization has clearly indicated that the architecture of natural composites such as bone and nacre is responsible for their high tensile and toughness properties. Also, various authors have proposed innovative methods to produce architecturally complex structures with a high level of control and have shown that outstanding properties can be achieved (Habraken et al. 2016).
The medical needs of an increasingly aging population have prompted a great deal of research in search of new biomaterials for the manufacture of products for many uses. These biomaterials are used to regenerate and repair living tissues damaged by disease or trauma. For specific clinical applications, mainly in orthopedics and dentistry, such as drug delivery agents, biocomposites filler, as coatings in metals and in bone implants, bioceramics play a key role (Aparicio 2014).
Calcium Phosphate
Calcium phosphate (CaP) compounds have been investigated as bone repair materials since 1920. However, they were of little use in clinical applications until the 1970s, when CaP materials were used as bone substitutes in the form of porous blocks and granules (Vallet-Regi and Salinas 2009). The clinical potential of CaP materials increased further in the early 1980s when LeGeros postulated the concept of calcium phosphate-based cements, and which was concretized by Brown and Chow in 1983 when publishing the first study on a bone cement that was set at a physiological temperature and meant the possibility of obtaining HA in monolithic form (Szurkowska and Kolmas 2017). The cement was obtained by mixing two salts of calcium phosphate, specifically a basic phase teracdlcico phosphate and an acid phase, dicalcium phosphate dihydrated with an aqueous solution, as a result a paste was set that set at room temperature (O’Neill et al. 2017; Brown 1987).
Cement setting is the result of a process of dissolving the reagents and precipitation of a new phase, which takes place at room or body temperature. The entanglement between the precipitated crystals is responsible for the setting and hardening of the cement. CaP cements are produced by two types of setting reaction. The first occurs with an acid-base reaction; a relatively acidic calcium phosphate phase reacts with a relatively alkaline phase producing a neutral calcium phosphate phase. The second setting reaction occurs when the initial calcium phosphate and the final product of the setting reaction share the same Ca/P molar ratio. CaP cements have a microporous structure after setting, with quite high porosities that can vary from 30 to 50%, depending on various processing factors such as the powder-liquid ratio used (Brown 1987; Regi et al. 2000). In addition, CaP cements exhibit unique bioactive and osteoconductive properties that make them a viable alternative for bone regeneration, have excellent handling properties (i.e., injectability, moldability and cohesion). This gives them the additional advantage of being molded at irregularly shaped defect sites (O’Neill et al. 2017; Kucko et al. 2019). Compared with grafts of spongy bone or other bone substitutes, calcium phosphate has a much higher compressive strength (4 to 10 times greater than spongy bone once hardened) (Hak 2007). Calcium phosphate cements have been used clinically as bone grafts in dental and orthopedic applications (Dorozhkin 2015). In general, the calcium phosphate powder component is kneaded with setting liquid to produce a paste-like fluid, which is injected or filled by hand to cover bone defects with complicated shapes. Therefore, the use of CaP cements makes minimally invasive operations possible (Unuma and Matsushima 2013).
Some clinical studies have reported the use of CaP cement in fractures of the distal radius; in a prospective study 110 patients over 50 years with fracture of the distal radius were randomized to form two groups; a group with conventional immobilization treatment and another group with CaP cement treatment where patients treated with calcium phosphate, 82% had a satisfactory result, compared with a 56% result in the conventional immobilization group (Sanchez-Sotelo et al. 2000). Another small prospective randomized study compared calcium phosphate cement (Bone Source) with an autograft in the treatment of fractures with metaphyseal defects. Twenty-nine patients with 30 defects were evaluated for reduction radiographic loss and clinical outcome at 6 months follow-up. The majority of patients had sustained fracture of the tibial plateau or distal radius. Joint reduction was maintained in 83% of patients treated with calcium phosphate cement (Bone-Source), compared with 67% of those treated with autograft (Dickson et al. 2002). Despite the progress made towards the manufacture of CaP cements that possesses a variety of surface and chemical characteristics, the influence ofmaterial properties in the organization of cellular events such as adhesion and differentiation is still poorly understood (Samavedi et al. 2013).
Table 4.1. Summary of the uses of calcium phosphate.
Ceramic | Applications | Reference |
Calcium Phosphate |
○ Bone gaff to restore bone defects caused by periodontal disease ○ Scaffolding for bone or dentin regeneration ○ Orthodontic cement ○ Coating material for the surface of dental implants |
(Samavedi et al. 2013) |
Calcium Sulfate
The hemihydrated form of calcium sulfate is more commonly known by orthopedic surgeons as plaster of Paris (Fig. 4.1), a material used for splinting and plastering (Hak 2007). It has long been used to immobilize fracture areas, and has also been widely used as dental impression material (Tang et al. 2010).
Calcium sulfate has been used since 1892 to fill bone defects and to act as a bone graft substitute. During the last 75 years, several authors reported variability on its use (Table 4.2), although in general with good results as a graft of bone defects, without complications attributed to calcium sulfate. Thefirst results were variable, probably due to the inconsistent crystalline structure, purity and quality of calcium sulfate (Kelly et al. 2001). Some histological studies on the use of calcium sulfate in animals have shown that the trabecular bone obtained in surgically created bone defects is qualitatively similar to that observed after using an autogenous bone graft (Turner et al. 2001). Currently calcium sulfate is available in individual pellets or as a powder that can be mixed in solution to form an injectable paste or molded to form the desired shapes; its compressive strength is similar to that of a spongy bone (Pietrzak and Ronk 2000). Of the available bone graft substitutes, calcium sulfate is the one that reabsorbs more rapidly (4-6 weeks) (Hak 2007). Several studies have reported the use of calcium sulfate; During the 50s and 60s, Peltier et al. described data on their clinical experience in the use of calcium sulfate to fill bone defects (Peltier et al. 1957); in the 70s Peltier and Jones reported the results of 26 patients who had been treated by unicameral bone cysts with calcium sulfate with a long-term follow-up (1 to 20 years), where 92.3% of the patients had a successful cure of the defect with bone formation in the cyst, without complication or need for additional surgery (Peltier et al. 1978). In the 1980s, Coetzee reported that 110 patients treated with calcium sulfate mainly for bone defects in the skull and facial bones, where he concluded that calcium sulfate was an excellent substitute for bone grafting (Coetzee 1980). By the 2000s Watson reported promising results on the use of injectable calcium sulfate in five patients with tibial plateau fracture and in three patients with tibial pylon fracture (Watson 2004). On the other hand, Moed et al. reported the use of calcium sulfate implanted during acetabular fracture surgery in 32 patients, whereby monitoring CT scans they showed good bone growth (> 90%) in 22 of the 31 patients (Moed et al. 2003).

Figure 4.1. Calcium sulfate. The different morphology of the dust particles, in general irregular prisms of 50-80 pm, is observed by SEM micrograph. In addition, a composition of 48% 0, 27% Ca and 14% S, the main components of Ca504, was identified by EDS analysis, and traces of typical plaster contaminants were found, such as 9% Si, 1% Na and 0.3% Mg. Own source.
Table 4.2. Some clinical uses of calcium sulfate.
Ceramic | Application | Reference |
Calcium Sulfate |
○Fracture zone immobilizer ○Dental impression material ○Bone substitute |
(Balhaddad et al. 2019) |
Tricalcium Phosphate
It has been known for more than 250 years that calcium phosphates are a component of the human body. Therefore, it was logical to assume that this material was thought of as a natural substitute for bone. Within calcium phosphates, one of the most used for this purpose is tricalcium phosphate (TCP). The first reported case of a tricalcium phosphate used as a graft dates back to 1920 by Albee who reported that a TCP graft promoted the formation of new bone tissue (Moed et al. 2003). Tricalcium phosphate is a bioceramics of formula Ca, (PO4)2, which is divided into two main groups, the form a and 13, both with the same Ca/P ratio of 1.5 but with different crystallographic, monoclinic and rhombohedral structures, respectively (Samavedi et al. 2013; Barrere et al. 2006; Jeong et al. 2019). This structure gives them different properties, since it depends on their stability, solubility, mechanical resistance and even their biological properties, and therefore their final applicability. The a form is generally obtained by two methods, the first is by means of a thermal transformation in which the temperature rises above 1125°C of some precursor with a Ca/P ratio of 1.5, such as hydroxyapatite deficient in calcium, amorphous calcium phosphate or the β-TCP form. The second method of obtaining it is by the so-called solid state reaction, in which precursors are mixed and the temperature is raised in the same way. Some authors recognize an α’ form that occurs only at temperatures greater than 1430°C and returns to the a form when cooled below its transition temperature, and therefore lacks clinical interest (Carrodeguas and De Aza 2011). In general, the α-TCP form is more soluble and less stable, and also has less mechanical resistance than the β-TCP form, so it is not used as a bone graft, but rather as cement, which can be accompanied by a polymer that functions as a vehicle for α-TCP particles. Colpo and collaborators developed an α-TCP cement using an acrylamide-based polymer as a vehicle, which was also used to release drugs in a controlled manner (Colpo et al. 2018). An and collaborators tested an α-TCP cement together with carboxymethylcellulose and hyaluronic acid, achieving values of up to 10 MPa, which shows that polymers also help improve their mechanical properties (An et al. 2016). The α-TCP cements set by a reaction in which three α-TCP molecules incorporate hydrogen from water to form an acidic phosphate, also adding a hydroxyl group to the resulting molecule (Gildenhaar et al. 2012).
The β form can be obtained by various methods, the most common is by calcination at a temperature of 900 to 1100°C, it is more stable at room temperature and has been reported to have osteoconductive and osteoinductive properties, it has also been shown to stimulate the differentiation to preosteoblastic cells, therefore, is mainly used as a bone graft. Guillaume proposes that β-TCP can be used to fill in different types of bone defects in maxillofacial surgery such as: post extraction alveoli, apicectomy defects or prior to the placement of an implant to increase bone volume in different areas, etc. (Guillaume 2017).
When β-TCP is in the presence of water (physiological fluids) at 37°C, a reaction occurs in which hydroxyapatite is formed with the release of calcium and acid phosphates to the surrounding environment, which lowers the pH of the area, and increases its reabsorption, it is important to note that both the a and 13 forms have different dissolution kinetics at different pH, the a form being more soluble, as mentioned earlier (Carrodeguas and De Aza 2011; Ratner et al. 2013).
The different dissolution and absorption rates are important for these types of materials, and depend on their physicochemical properties, their porosity, their defects in the crystallographic network, and the processes to which they are subjected, for example, if they are sintered. It is well known that hydroxyapatite is not completely reabsorbed, hindering the formation of new bone tissue. In general, β-TCP is reabsorbed for 6 to 9 months, which makes it an ideal candidate for use as scaffolding (Guillaume 2017). While α-TCP is dissolved by the physiological medium, in the 13 form, in addition to the physiological medium, osteoclasts appear to be involved, since, when these are experimentally inhibited, the remodeling process becomes slower (Tanaka et al. 2017).
However, the process by which graft remodeling occurs is more complex than it might seem at first instance, although β-TCP has an acceptable solubility rate, it can release excess calcium ions that prevent osteoclast activity (Yamada et al. 1997), and it is important to remember that for efficient bone remodeling, a coordinated mechanism between osteoclasts and osteoblasts is needed.
Through a sintering process the degree of graft porosity can be controlled, it has been shown that porosities less than 100 lam influence the rate of reabsorption of the material, facilitating the apposition of osteoblasts on the material (Chan et al. 2015), in addition, a porosity insufficiency does not allow the formation of blood vessels, however, excessive porosity considerably decreases the mechanical strength of the material.
Although both forms of TCP are reabsorbed faster than hydroxyapatite, they lack some of its advantages, which is why it has been proven to form composite ceramics between these materials, giving rise to the so-called Biphasic Calcium Phosphate (BCP), with the intention to control the rate of graft resorption, and take advantage of each of its elements (Samavedi et al. 2013). Chan and colleagues conducted a study to compare commercial hydroxyapatite against a BCP formed by α-TCP and hydroxyapatite, and demonstrated that the BCP had better osteoinductivity in vitro (Chan et al. 2015). One of the most innovative applications of tricalcium phosphates is in stereolithography, which is an additive-type 3-D printing technique based on a powder-liquid system, in the dental area it is used in prosthetics and maxillofacial surgery to develop models of study, however, it has been proposed that by this 3-D printing technique grafts based on calcium phosphates can be made with the advantage that they will fill the defect perfectly. For example, klammert and collaborators performed a mixture of 45% α-TCP and 55%β-TCP (Klammert et al. 2010). Khalyfa et al., tested a combination of tetra calcium phosphate and tricalcium phosphate to perform models on a stereolithograph, showing good results in cell proliferation (Khalyfa et al. 2007).
Table 4.3. Clinical applications of TCP.
Ceramic | Application | Reference |
α-TCP | Bone cement | (Carrodeguas and De Aza 2011) |
α-TCP | Bone cement | (Colpo et al. 2018) |
β-TCP | Bone graft | (Gildenhaar et al. 2012) |
BCP | Bone graft | (Yamada et al. 1997) |
α-TCP and [3-TCP | Graft in Stereolithograph | (Chan et al. 2015) |
β-TCP | Graft in Stereolithograph | (Klammert et al. 2010) |
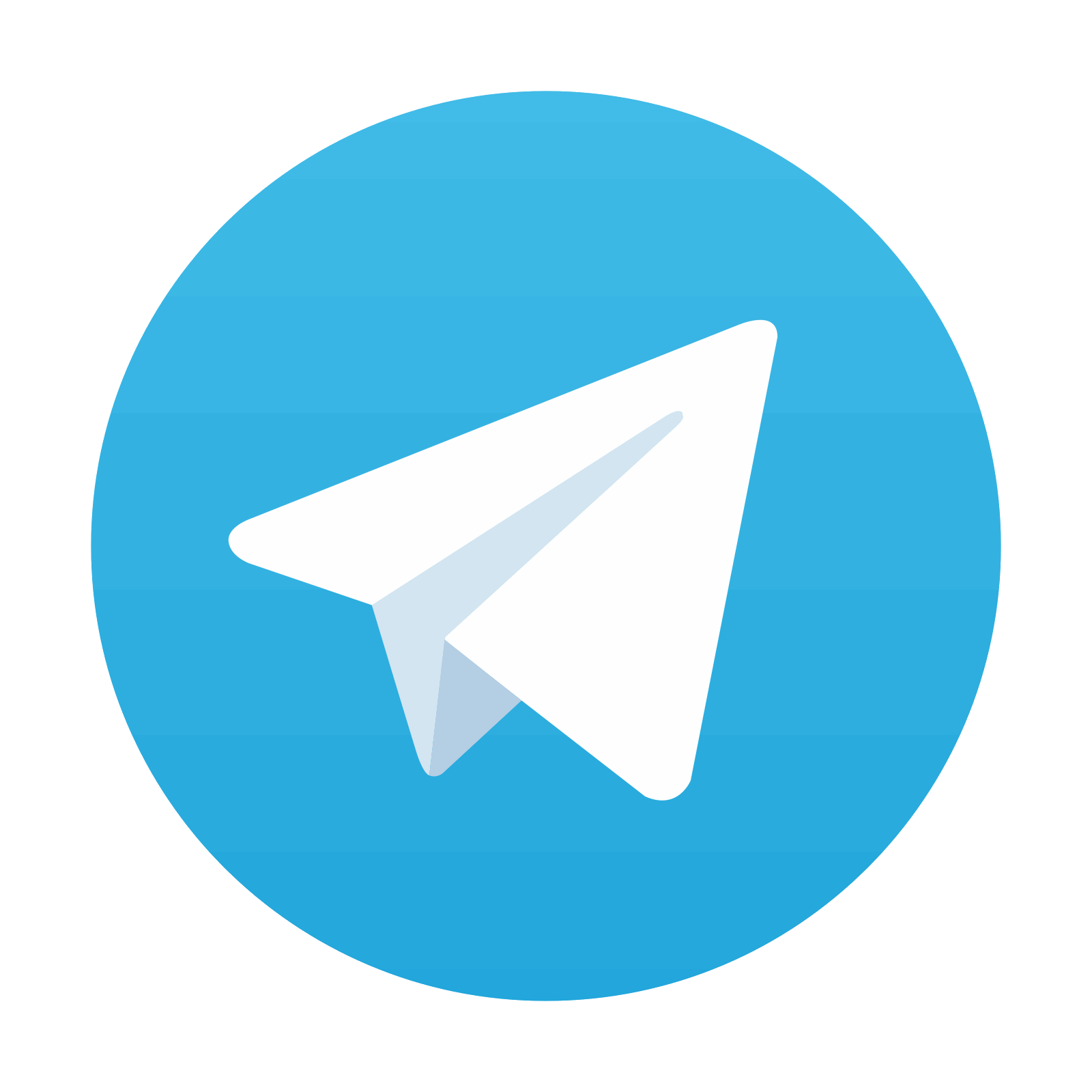
Stay updated, free dental videos. Join our Telegram channel

VIDEdental - Online dental courses
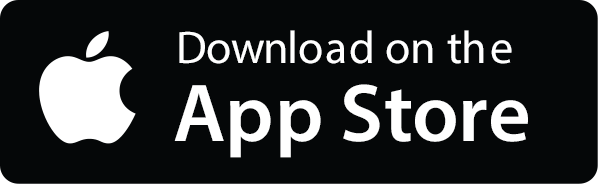
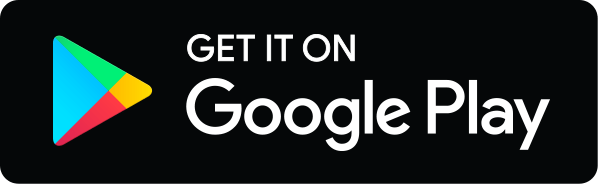