5
From Conventional Approaches to Sol-gel Chemistry and Strategies for the Design of 3D Additive Manufactured Scaffolds for Craniofacial Tissue Engineering
Introduction
Over the past two decades, great interest has been focused on craniofacial tissue engineering (Langer and Vacanti 1993; Hollister 2005; Thrivikraman et al. 2017). In particular, a significant contribution was clearly due to the research and development in the field of bone augmentation, thus leading to tissue engineering as an alternative treatment option in dentistry and medicine (Bertassoni and Coelho 2015; Thrivikraman et al. 2017).
In this context, biomaterials play an important role for tissue regeneration as it is widely recognized how their properties may be suitably tailored by varying the composition and, in many cases, the architecture, in order to regulate the cell microenvironment during the tissue formation process, also controlling the rate of regeneration (Bertassoni and Coelho 2015; Thrivikraman et al. 2017).
Generally, biomaterials are used as scaffold systems which should allow cell migration, proliferation and differentiation, thus promoting new tissue formation.
With regard to craniofacial bone regeneration and augmentation, a wide range of organic and inorganic biomaterials have been considered. As an example, bioceramics such as calcium phosphate (CaP) have been used to develop ‘inorganic’ scaffolds, whereas synthetic and natural biopolymers have been taken into account to manufacture ‘organic’ scaffolds.
The great effort to mimic the organic-inorganic composition and the structure of the natural bone, in which crystallites of a natural bioceramic (hydroxyapatite) reinforce the fibrils of a natural organic polymer (collagen), has led research towards the design of strong and durable biomaterials (Thrivikraman et al. 2017).
In the current chapter, an overview of conventional and recent developments in the field of craniofacial bone regeneration and augmentation will be first reported. Bioceramics, bioglasses, biopolymers and biocomposites will be described together with their peculiar features. Furthermore, starting from experimental and theoretical analysis performed on the material, a special focus will be devoted to the potential combination of sol-gel chemistry and CAD-based approach to design additively manufactured scaffolds for craniofacial tissue engineering.
Materials: Bioceramics, Bioglasses, Biopolymers and Biocomposites
Most candidate materials for bone regeneration are represented by bioceramics such as calcium phosphates (CaP), calcium carbonates, calcium sulfates, bioactive glasses and composites consisting of biodegradable polymers and bioactive inorganic materials (Gerhardt and Boccaccini 2010; Thrivikraman et al. 2017).
The ability of CaP bioceramics to stimulate bone growth is well documented as in the 1920s an effort to improve bone formation was made using an aqueous slurry of ‘triple calcium phosphate’ (Albee and Morrison 1920). In the late 90s, CaP materials started to receive great attention in the field of bone tissue engineering, as a consequence of their ability to rapidly promote bone formation (Langer and Vacanti 1993; Thrivikraman et al. 2017).
Many reasons have been frequently reported to justify their interesting properties: in terms of composition, the similarities to hydroxyapatite, which is the main constituent of the bone; osteoprogenitor cells are able to process and resorb them; the possibility to trigger the intracellular signaling of osteogenesis through the presence of inorganic phosphates and soluble calcium (Thrivikraman et al. 2017) derived from byproducts of CaP crystal dissolution (Thrivikraman et al. 2017).
Many commercial CaP materials are currently available for bone regeneration, generally including one or more phases of CaP in different mineral phases, processing conditions and crystal structures. Experimental studies have also demonstrated that the specific material phase which constitutes the CaP materials strongly determines the efficacy of the osteogenesis process. A key factor which regulates osteoinduction is represented by the solubility of the CaP mineral phase (Kamakura et al. 2002; Thrivikraman et al. 2017).
The synthesis of materials with a composition similar to the mineral phase of the natural bone was a starting point towards the design of scaffolds consisting of CaP and possessing high mechanical properties (Galea et al. 2008; Thrivikraman et al. 2017). Thus, sintered hydroxyapatite (HA) or sintered 13-tricalcium phosphate (13-TCP) were initially obtained by CaP graft sintering.
Anyway, scaffolds fabricated by the sintering process have low injectability and are generally too brittle for load bearing applications. In addition, the process of scaffold remodeling and osteoclast-driven biodegradation is hindered by their low solubility.
In fabricating novel CaP scaffolds for bone regeneration, a wide range of possibilities was offered by the development of bone cements consisting of CaP phases which may be formed at room temperature (i.e., calcium deficient HA, octacalcium phosphate, monetite and brushite) (Munting et al. 1993; Steffen et al. 2001; Thrivikraman et al. 2017).
Interestingly, such materials with less crystalline phases possess a much lower solubility rates and may transform into a more stable HA phase upon implantation (Suzuki et al. 1991; Constantz et al. 1998; Bohner et al. 2003; Thrivikraman et al. 2017), also showing better biological performances if compared to sintered CaP (Thrivikraman et al. 2017).
From a historical point of view, CaP materials have been processed in different forms, including particulates, solid and porous blocks, and this led to a limitation for their clinical use in dentistry, if compared to injectable materials with enhanced handling characteristics and less invasive features.
Furthermore, in comparison to soft and moldable sponges and polymers, it is often difficult to strictly control the shape and architecture for particulate materials. Thus, their applications are largely limited.
Although many efforts have been made to properly understand the mechanisms related to CaP-induced bone formation, pre-clinical results have shown interesting results (Thrivikraman et al. 2017).
In this context, it was demonstrated that similar results were obtained when using an osteoinductive TCP ceramic, an autograft scaffold and scaffolds loaded with growth factors such as rhBMP-2 or recombinant human BMP (Yuan et al. 2010; Thrivikraman et al. 2017). Moreover, the use of CaP materials results in very promising craniofacial bone regeneration, taking into consideration the interesting results obtained from clinical trials on commercially available products.
However, many analyses performed on HA, TCP and biphasic calcium phosphate have generally shown how the presence of a more soluble phase may improve bone formation, even if the ability to induce bone formation has already been displayed by several compositions and phases of CaP (Habibovic et al. 2005; Yuan et al. 2006; Thrivikraman et al. 2017). Grain size, specific surface area and microporosity of CaP are features playing a key role in osteoinduction (Hulbert et al. 1970; Klawitter and Hulbert 1971; Klein et al. 1985; Thrivikraman et al. 2017).
Methodologies involving the combination of CaP materials with pre-fabricated blood vessels and capillaries, as well as a vascularized collagen-13-tricalcium phosphate graft, would represent novel strategies for the development of advanced scaffolds (Kang et al. 2013; 2015; Thrivikraman et al. 2017).
Bio-inorganic substitution of CaP materials and bioglasses represent further interesting approaches. It is well known that the mineral phase of the natural bone is characterized by many trace elements, and a cationic substitution with Sr of Mg on CaP-based scaffolds may alter the biological and mechanical performances, as they modify the physiochemical properties of CaP (i.e., microstructure, crystallinity, solubility) (Tarafder et al. 2013; Thrivikraman et al. 2017). A study in an animal model reported the effect of SrO and MgO doping ß-TCP scaffolds resulting in an increase of the early bone formation in the doped structures, in comparison to the undoped ones (Tarafder et al. 2013; Thrivikraman et al. 2017).
Like the CaP materials, bioactive glass and glass-ceramics have also been widely investigated and proposed as potential candidates for bone regeneration (Kaur et al. 2014; Thrivikraman et al. 2017). The ability of bioglasses to induce the formation of a hydroxycarbonate apatite layer, when exposed to body fluids, has been discussed in the literature (Gerhardt and Boccaccini 2010; Thrivikraman et al. 2017). Even though bioactive glasses and glass ceramics are commercially available in particulate form, their use for load-bearing devices is strongly limited by their low strength and low fracture toughness.
Taking into account the potential to promote the osteogenic cell response, bioactive glasses are generally employed as fillers or coatings for polymer-based scaffolds. BonAlive® (BonAlive Biomaterials Ltda), Perioglass® (NovaBone), GlassBone® (Noraker) and Vitoss® (Stryker) are examples of current materials for bone regeneration available for clinical applications. However, despite the interesting results obtained from these kinds of materials in comparison to the typical CaP sintered ceramics, studies are still ongoing to assess their long term clinical performance and their efficiency for craniofacial applications (Thrivikraman et al. 2017).
With regard to biopolymers, gels and hydrogels have been studied for cell and growth factor delivery. The attention on natural polymers (i.e., gelatin, collagen, silk, chitosan) has been focused with the aim to mimic the chemical composition, structure and biochemical properties of the organic matrix of the natural bone, considering their low immunogenic properties, as well as their ability to stimulate cell response and function, and to support tissue remodeling (Russo et al. 2013; Thrivikraman et al. 2017).
In the field of tissue regeneration, collagen represents an immediate choice because of its presence in the extracellular matrix (ECM) of vertebrates as the most abundant protein.
The low mechanical properties of collagen limit its applications in the case of bone regeneration. In addition, it cannot be mass produced and risks of allergic reactions and infections may be related to the use of animal tissues, even if the use of recombinant collagen may reduce these drawbacks (Lynn et al. 2004; Adachi et al. 2006; Thrivikraman et al. 2017). Alginate, chitosan and agarose are natural polysaccharides which can be considered to develop polymeric scaffolds. The presence of positively charged amino groups enables the interactions with DNA, proteins, lipids and cell membranes.
In the case of chitosan, cytokines and growth factors may be stimulated by its cationic nature which favors the interactions with proteoglycans and glycosaminoglycans, playing an important role in the tissue regeneration process (Costa-Pinto et al. 2011; Thrivikraman et al. 2017). Silk fibroin has also provided interesting results in terms of cell proliferation and in vivo bone formation (Meechaisue et al. 2007; Zhang et al. 2010; Uebersax et al. 2013; Thrivikraman et al. 2017). The low mechanical strength obviously limits its applications in the biomedical field (Thrivikraman et al. 2017).
The Demineralized Bone Matrix (DBM) is a further kind of bone substitute which has been clinically studied (Sawkins et al. 2013; Kolk et al. 2012; Thrivikraman et al. 2017).
It mainly consists of type I collagen (about 90%) with several growth factors (i.e., osteopontin, bone sialoprotein, IGF1, BMPs, as well as some inorganic phosphates, calcium-based particles and cellular debris (Gaskin et al. 2012; Holt and Grainger 2012; Thrivikraman et al. 2017).
Even if different DBM products are available in the market, they show osteoinductive and osteoconductive properties, but differ from each other in terms of sterilization methods, process conditions, donor specifications (Kinney et al. 2010; Thrivikraman et al. 2017). As for the osteoinductive ability, different DBM products generally provide different results. Surgical handling also affects the efficacy and, for this reason, DBM is often mixed with binders (i.e., hyaluronan, glycerol, bovine collagen with sodium alginate, gelatin, calcium sulfate, lecithin, carboxymethyl cellulose).
DBM may be used as a bone graft extender as it possesses low mechanical strength. It has also been considered better than standard autografts due to its ability to revascularize and locally induce the release of agents and growth factors promoting bone formation.
Regarding synthetic polymers, polylactic acid (PLA), polyglycolide (PGA), poly(lactide-co-glycolide) (PLGA), poly(E-caprolactone) (PCL), polyhydroxyalkanoates (PHAs) and (propylene fumarate) (PPF). Such polymers have been developed to tailor the mechanical properties of the scaffolds by varying the degrees of cross-linking and concentration or by means of copolymerization strategies (Thrivikraman et al. 2017).
In particular, over the past years a great deal of attention has been focused on PCL, which is a biodegradable aliphatic polyester (Gloria et al. 2010; 2012; 2019; Thrivikraman et al. 2017).
Generally, neat synthetic polymers also do not properly satisfy the mechanical requirements for bone tissue engineering due to their flexibility and weakness. Thus, polymer-based ‘biocomposites’, consisting of biopolymers reinforced with inorganic fillers, have been considered an intriguing alternative for hard-tissue engineering.
If compared to neat polymers, polymer-based composites should possess enhanced mechanical properties and better structural integrity and flexibility than brittle ceramics. Porous composite scaffolds with improved bioactivity and tailored resorption rate were obtained by properly combining polymers and inorganic fillers, showing a combination of the best features of their constituents, and, in many cases, further properties which the single constituents generally do not show (Gloria et al. 2010).
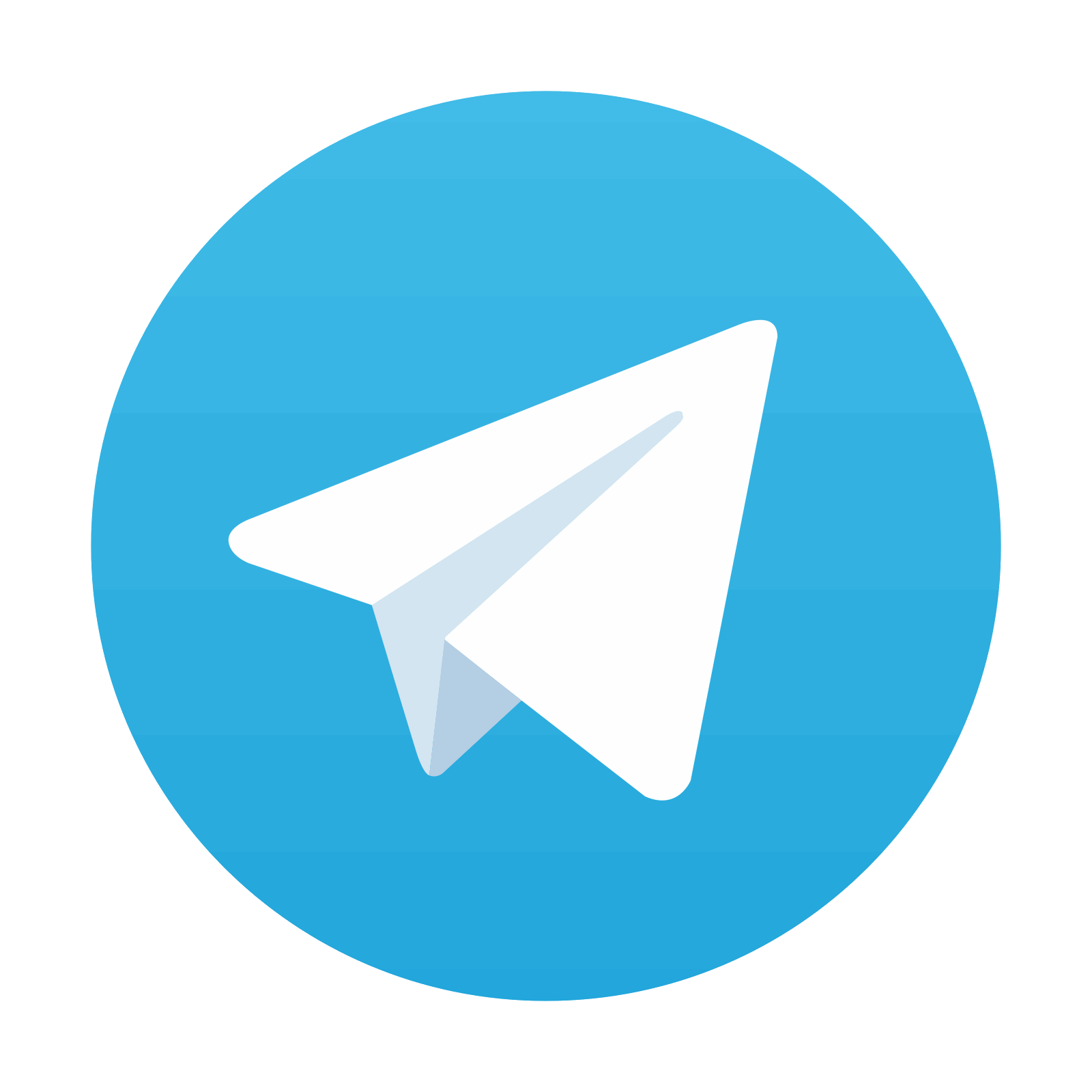
Stay updated, free dental videos. Join our Telegram channel

VIDEdental - Online dental courses
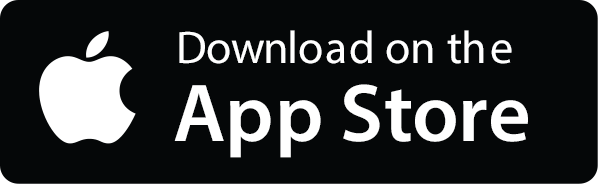
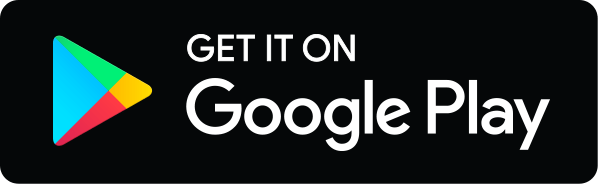