8
Biomimetic Approaches for the Design and Development of Multifunctional Bioresorbable Layered Scaffolds for Dental Regeneration
Introduction
The inability of most tissues and organs in adult humans to regenerate after damage, has been a serious problem that affects doctors, dentists and, of course, patients. Among them, the tooth is a complex organ made of highly mineralized tissues (alveolar bone, cement, dentin, enamel) and non-mineralized periodontal ligament (PDL), which connects the alveolar bone to the cement and guarantees the functionality and stability of the tooth. Dental diseases are extremely widespread all over the world, such as periodontitis, pulpitis and others that require a reconstruction of the dental apparatus. Biocompatible prosthetic devices have provided options in many cases helping millions of people, but the body’s reaction to these devices is far from ideal. Complications including thrombosis, infections, ongoing inflammatory reactions, excessive fibrosis, impaired functions, mobilization and extrusion, are problematic for patients and expensive for the healthcare system. In dentistry, titanium and bioceramics implants or materials such as stainless steel, silicone rubber and poly methacrylate are widely used for the reconstruction of dental apparatus. However, the failure of these devices due to healing problems, infection and overload problems is well recognized Nowadays, the accepted standard for defining success in dental implants, has become a phenomenon called osseointegration, associated to a concept of years of reliable function rather than device life. The challenge for osseointegration is the stimulation of the right response in the surrounding tissue, which is strictly related to the material and the implant chemistry and features.
In the last 50 years, innovative technologies and materials inspired by nature have been designed, among them biomaterials, that play an exciting role in the field of regenerative medicine where biomimetic has now become a driving concept. Biomimetic in this field means examining the nature, its models, systems, processes and elements to take inspiration and emulate them to solve technological problems for human health. In fact, the close reproduction of the physical-chemical, morphological and mechanical characteristics of the targeted tissues provides biomaterials with the ability to exchange information with cells and trigger the tissue regeneration cascade (Preti et al. 2019).
In particular, new synthetic methods that allow the controlled growth of crystals and the multiscale organized structures are attracting increasing attention. In this way, nature is studied not only to develop new and biomimetic materials, but also to imitate the natural processes and design innovative methods of syntheses. An example of a highly biomimetic process investigated to develop bone-like biomaterials is biomineralization, a natural assembly process that has been successfully reproduced in the laboratory to induce a heterogeneous nucleation of inorganic nanocrystals on an organic matrix, to produce hybrid scaffolds with compositional, morphological and structural characteristics similar to natural mineralized tissues. This process is the basis of bones, shells and exoskeletons generation and allows the design of bio-hybrid materials with unique properties that cannot be obtained from conventional approaches (Campodoai et al. 2018).
As in bone, the event active in the formation of the different dental tissues involves collagen and proteoglycans as macromolecular assembled matrix, mineralized with variable ratio of hydroxyapatite (HA) nanocrystals and organized into complex fibrous constructs with graded morphologies and structures. Being able to develop novel biomimetic scaffolds providing suitable chemical and mechanical cues allows to promote the exchanges of effective information with the endogenous cells and trigger tissue regeneration, avoiding the use of inert and non-biomimetic prostheses often associated with problems related to inflammations and infections reactions (Sprio et al. 2018).
Biomimesis and Biomineralization: From Nature to the Laboratory
The word biomimetics was first used in the late 1950s when it was coined by the biophysics Otto Schmitt who used it to describe the transfer of ideas and analogues from biology to technology. More precisely, he studied and described the formation, the structure and the function of biological substances and materials and the biological mechanisms (as protein synthesis or photosynthesis) especially for the purpose of synthesizing similar products by artificial processes which mimic natural ones (Ramalingam et al. 2013; Hwang et al. 2015).
Some effective examples of biomimetics are, actually, very well-known everyday usage items like, for example, Velcro®. Velcro® is considered the first practical application of a biomimetic principle. In the early 1940s, the Swiss inventor George de Mestral went for a walk with his dog and on returning home, he noticed that his dog coat and his pants were covered with cockleburs. His curiosity led him to study the burrs under a microscope, discovering their natural hook-like shape. This was to become the basis for a unique, two-sided fastener, one side with stiff ‘hooks’ like the burrs and the other side with the soft ‘loops’ like the fabric of his pants. The result was Velcro® (1959) brand hook and loop fasteners, named for the French words ‘velour’ and ‘crochet’ (Saunders 2015).
Biomimetics means, to look towards nature for ideas that may be adapted and adopted for solving problems or create new solutions. One of the natural processes to which biomimetic takes inspiration is without any doubt biomineralization, a very peculiar, natural, as well as transversal phenomenon, of interest to many different living organisms from diatoms to humans, passed by plants. It refers to the processes by which organisms form minerals starting from simple compounds and are defined biogenic minerals or biominerals. It is, therefore, by definition, a true multidisciplinary field that spans from both the inorganic and the organic world. But, why should living organisms form minerals? The main functions of mineralized tissues can many, among them protection, motion, cutting, grinding, optical, magnetic and gravity sensing as well as storage (Talham 2002; Weiner 2003).
Among biogenic minerals approximately 80% are crystalline and 20% are amorphous. Calcium minerals account for about 50% of biogenic minerals and the most widespread are carbonates, phosphates, silicates and iron oxides. About 60% of the known biogenic minerals types contain hydroxyl groups and/or bound water molecules and the abundance of hydrated biominerals is not accidental. Hydrated phases are favoured over anhydrous counterparts by significantly lowering energetic barriers to nucleation and growth from aqueous solution (Rao and Cölfen 2016; Crichton 2019). Organisms are metabolic misers, thus, they use the Ostwald-Lussac rule to their advantage by favouring the precipitation of the lowest energy phases (Coombs 1984).
Biomineralized materials do not contain just mineral components but both inorganic and organic ones finely organized and chemically interacting thus to create a new hybrid material endowed with new smart properties and are used to build exo- as well as endoskeletons. Most invertebrates use chitin based structures, that are largely organic while vertebrates, in their bones and teeth, use biomineral constructions made of both organic and inorganic components consisting of calcium phosphate nano-crystals growth on collagen. The inorganic component confers mechanical resistance without which large land-living animals could not exist, but it also brings brittleness, therefore, an organic matrix is required to provide elasticity and tensile strength to the final hybrid material. Moreover, the organic matrix is also responsible for the control of nucleation and growth of crystals of the inorganic component. Biogenic minerals are mainly characterized by mild conditions of temperature and pressure synthesis, unusual morphology and unusual mechanical properties. Typically, the organic matrix is laid down first and the inorganic reinforcing phase grows within this organic matrix template that confers important and unique feature to the crystals (Weiner 2003; Chen et al. 2019).
One of the major characteristics of biomineralization strategies is, the use of the bottom up approaches, in which the material is built starting at the atomic and molecular scale, leading to the formation of nanostructured building blocks, which in turn organize into complex hierarchical structures. This take the name of biomineral tectonics, an integrated process of construction that extends across many length scales and results in higher ordered structures. Although these tissues are made of relatively weak components at ambient conditions, their hierarchical structural organization and intimate interactions between different elements lead to superior mechanical properties (Talham 2002; Beniash 2011).
Biomineralization is one of the natural processes that has been successfully reproduced in the laboratory, with the induction of the inorganic phase heterogeneous nucleation onto the organic matrix through fine mechanisms, controlled and driven by the organic matrix itself (Fig. 8.1). The chemical interaction between the elected organic matrix and the grown-into-it inorganic phases allow to obtain hybrid materials with unique features that are more than just a pro sum of the single parts, exactly as it happens in nature. Moreover, a proper doping with inorganic ions [e.g., Mg+, Sr+, CO32- , Fe2+/3+] makes it possible to adapt the composite material properties, like biodegradation, cell adhesion and growth, rather than magnetism, for the final purpose (Campodoni et al. 2016; Sprio et al. 2018; Tampieri et al. 2008).
In the early 2000s a nature-inspired fabrication process to obtain 3D scaffolds strongly mimicking hard human tissues was successfully drawn up. The developed process takes advantage of the complex chemical-physical, topological and ultrastructural information stored into the collagen molecule to induce hierarchical self-assembly and biological-like low-crystalline hydroxyapatite nucleation, with the help of foreign ions incorporation into the apatite lattice.
In details, to in-laboratory reproducing the biomineralization process performing a neutralization reaction where the acid solution containing the PO43- was mixed with collagen gel and then dropped into a Ca2+ ions-containing alkaline solution. The pH of the suspension was then decreased until it reached the neutral value to trigger two different mechanisms at a time: meeting the isoelectric point of collagen so that it starts to assemble in fibers and then into a 3D network and starting the mineral nucleation in correspondence of the carboxylic groups exposed by collagen. The presence in the basic suspension of foreign ions that can be incorporated in the apatite lattice during its formation helps in obtaining a more amorphous and natural-like mineral phase and therefore a highly biomimetic hybrid material. The possibility for the apatite lattice to host many different isovalent and heterovalent ions allow to synthesize tailor-substituted apatite for different applications, an example is the MgHA/Coll biomimetic and fully bioresorbable hybrid material described by Tampieri et al. for the regeneration of the subchondral bone in a multi-layer scaffold designed for osteochondral regeneration (Fig. 8.1) (Tampieri et al. 2008).

Figure 8.1. (A) Overview on the hierarchical organization of natural bone tissue. (B) Schematic representation of the biomineralization process developed in lab-scale through a neutralization acid-base reaction (1). Mg-doped hydroxyapatite nanocrystals (MgHA) were grown on collagen fibers (Coll) by to obtain a hybrid biomaterial (MgHA/Coll) mimetic of the bone mineralized matrix (2). In detail the collagen fibers, investigated with ESEM, before (3’) and after (3”) the biomineralization process.
Besides collagen, many other polymers have been exploited as possible matrices for biomineralization; among them gelatin, chitosan, alginate and nano-cellulose (Campodoni et al. 2016; Panseri et al. 2016) Thanks to their chemical structure, all of the above-mentioned biopolymers are able to undertake the biomineralization process with interesting different final results in terms of mechanical and physical properties, biological activity and biodegradation kinetic that can be exploited to achieve disparate regenerative goals.
The possibility to in-laboratory the polymer assembly directly and the inorganic phase nucleation and growing on it, paves the way for obtaining better customized hybrid mineralized gels possible to be further processed in 3D scaffolds or carriers depending on the therapeutic need (Campodoni et al. 2018).
Biomaterials for Dental Regeneration
After several years of research, the evolution of biomaterials for tissue regeneration has seen a quick upgrade of fundamental concepts which biomaterials have to respect. At the beginning of the biomaterials research, the first requirement was non-toxicity, which meant avoiding to stimulate undesired reactions and the death of the surrounding tissue, in that period bio-inert products were developed.
Nowadays, new emerging concepts have been taking hold in this field such as bioactivity, bioresorbability and biomimicry that allow to obtain materials capable of stimulating specific responses from the biological system (Tampieri et al. 2003; Bakopoulou et al. 2016). Firstly, a bioresorbable material is designed to stimulate regenerative reactions inducing the formation of a new tissue and to gradually degrade in safe by-products during the new tissue formation until complete material substitution. Secondly, bioactivity allows to stimulate a specific biological response producing a bond between the material and tissue; two different responses can be stimulated depending on the material which can be osteoconductive or osteoinductive (Kokubo et al. 2003; Panseri et al. 2014). An osteoconductive material is able to be colonized by the host tissue cells and promote the bone tissue formation thanks to the permissive morphology, e.g., pore dimensions and interconnection however, it is not able to induce a cell differentiation. This capability is instead typical of an osteoinductive material, which is able to recruit undifferentiated cells and stimulate them to evolve in osteoblastic cells promoting bone formation. This family of materials is the ideal solution only if they are endowed with the required chemical stability, degradation kinetics and mechanical strength. Together with features described above, the new generation of materials are generally defined as ‘smart’ because they can intrinsically and actively promote the capability of tissue to heal and self-repair by responding to internal and external environment stimuli, thus taking part in the regeneration of the damaged tissue (Tampieri et al. 2005; Iafisco et al. 2014; Mohammed and Gomaa 2016). To achieve this interaction, a smart biomaterial may also have biomimetic abilities, meaning the ability to mimic the function of extracellular matrix to stimulate cellular invasion, attachment and proliferation. This means that well-designed biomaterials are able to recruit resident stem cells and orchestrate their behaviors and functions to promote tissue regeneration, exploiting the intrinsic regenerative potential of endogenous tissues.
Biomimetic properties have been obtained by technological adaptation of the macro, micro and nanostrncture, by trying to replicate the hierarchical organization of natural tissues. Taking inspiration from nature it is possible to develop better materials in terms of chemical composition, 3D organization and mechanical performances, which lead to a better interaction with the cell and to improve their responses, for the achievement of vascularization and tissue ingrowth (Panseri et al. 2012; Sandri et al. 2016; Krishnakumar et al. 2018).
The concept mimicking the natural tissues to develop a bioresorbable material is largely applied in several areas such as orthopaedic and dental fields. In the orthopaedic field, several previous studies carried out by Tampieri et al. showed substantial mimicry of the composition and multi-scale structure of target native tissues enhancing regenerative ability to develop different kind of materials such as porous 3D scaffolds, injectable self-hardening bone cements, 3D hybrid scaffold for osteochondral and bone regenerations, biomimetic scaffolds obtained by biomorphic transformation for the regeneration of load-bearing segmental bones (Tampieri et al. 2008; 2019; Dapporto et al. 2016; Sprio et al. 2016). The same approaches were translated to the dental field, for which several biomaterials mimicking structure and composition of various tissues have been developed for the regeneration of different parts of the tooth; the next paragraphs will better describe examples of biomaterials for periodontium and dentin regeneration (Panseri et al. 2016; Sprio et al. 2018).
Mimicry of the Natural Gradient of the Periodontal Region
The tooth is a complex organ combining hard and soft tissues such as enamel, dentin, cementum and vascularized dental pulp; the latter is the central part of the tooth filled with soft connective tissue in close connection with dentin. It contains blood vessels and nerves that enter the tooth from a hole at the apex of the root and along the border between the dentin and the pulp are odontoblasts, which initiate the formation of dentin. Periodontium includes tissues surrounding and supporting the tooth such as cementum, periodontal ligament (PDL) and the alveolar bone (Fig. 8.2B) (Maeda et al. 2014). It is well known that stem cells, scaffolds and signal molecules are requisites for tissue engineering, especially, with regard to scaffolds, in addition to the chemistry of the material, it is important to mimic the ‘scale’ of these tissues. The periodontal ligament, in fact, has a thickness of about 20 µm, and the cementum of about 10 µm, each layer has a unique morphology and chemistry fundamental for its role and recognized by cells. A good mimesis of natural tissue reproducing the biophysical properties such as surface tomography, internal microstructure, scale and highly interconnected porous structure together with a good elasticity are key points to promote cell adhesion, migration, proliferation and differentiation (Lee et al. 2005; 2010; Dangaria et al. 2011).

Figure 8.2. (A) SEM images showing at the micro scale the multi-layer feature of a scaffold made of three biomaterials mimetic of cementum, periodontal ligament and alveolar bone. (B) Details of the complex structure of natural periodontal tissue that inspired the fabrication of the three-layer biomimetic periodontal scaffold.
Several materials are used for tissue regeneration classifiable in synthetic, natural, bioceramic and composite materials. Among synthetic materials available for periodontal regeneration are largely investigated poly-lactic acid (PLA), poly-glicolic acid (PLGA) polycaprolactone (PCL), all these materials have been developed with a suitable porous structure ideal for cell engraftment and proliferation (Galler et al. 2011; Kawase et al. 2011; Ba Linh et al. 2013; Bölgen et al. 2014). Galler et al. developed a polyethylene glycol blended with fibrin and dental stem cell from the human PDL and pulp displaying a good in vivo cell growth and differentiation due to a suitable 3D fabrication (Galler et al. 2011). Among natural materials, several of those are exploited for dental application such as gelatin, chitosan, collagen, fibrin and hyaluronic acid due to their favorable properties such as biodegradability, biocompatibility and bioresorbability. These features together to the possibility to generate 3D porous structures through well-known fabrication technologies allow to promote cell adhesion and proliferation, important for a suitable tissue regeneration (Panos et al. 2008; Wolf et al. 2013; Jimbo et al. 2014; Sugawara and Sato 2014). Furthermore, bioceramic materials for the fabrication of cement and bone replacement devices were also studied, including hydroxyapatite (HA) and ß-tricalcium phosphate (13-TCP), both calcium phosphates are widely used in the field of dentistry, orthopedic and plastic surgery (Xia et al. 2011; Kasaj et al. 2012; Lee et al. 2012; Suto et al. 2013).
Finally, composites of bioactive ceramic and polymers, in particular biomimetic biodegradable scaffolds are currently being developed by taking advantage of each component to increase mechanical stability and improve tissue interaction (Takechi et al. 2012; Tsuzuki et al. 2012; Maeda et al. 2014). The combination of HA and collagen has frequently been used to create biomimetic scaffolds in regenerative medicine because they greatly resemble natural tissues especially if they are synthesized by biomineralization process (Tampieri et al. 2003; Krishnakumar et al. 2018).
A novel strategy to develop scaffolds for periodontal regeneration mimicking the natural periodontal region well is to create a multi-layer construct with different region-specific pore/ channel sizes and material composition (Lee et al. 2014; Sprio et al. 2018). In particular, Sprio et al. developed a device that mimics the entire periodontium through three different layers, each of which mimics a specific tissue and is responsible for a well-defined function: PDL, cementum, alveolar bone. This construct has a biomimetic composition and a gradient structure able to instruct specialized cellular components of the periodontium to participate in the regenerative process (Fig. 8.2A) (Sprio et al. 2018). Alveolar bone-like layer is composed of mineralized collagen with Fe-doped HA (FeHA/Coll) highlighting chemic-physical features of natural tissue together with superparamagnetic properties able to stimulate the cell to reproduce and differentiate under weak magnetic fields (Silvia Panseri et al. 2012; Tampieri et al. 2012; 2014). The presence of Fe-ions, responsible for the generation of the magnetic properties, do not affect the biocompatibility and the degradability of the hybrid material, instead their effectiveness in accelerating tissue regeneration was reported (Glazer et al. 1997; Bañobre-López et al. 2011; Assiotis et al. 2012; Panseri et al. 2012). PDL-like layer is composed of a highly porous collagen membrane (Coll) in order to allow a high vascularization of cementum metabolically relating to nutrients diffused by PDL. Collagen is an excellent candidate because it is highly biomimetic and biocompatible, but its degradability is very fast, for this reason biocompatible chemical cross-linking agents, such as 1,4-butanediol diglycidyl ether (BDDGE), are selected to improve its permanence in vivo (Zeeman et al. 2000). Finally, a cementum-like layer is a very thin layer processed by electrospinning a composite blend of nano-sized FeHA with cellulose acetate (FeHA+CA). The technique chosen allows to obtain a mat composed of non-woven micrometric fibers very close to natural cementum, furthermore, the presence of nano-sized hydroxyapatite confers to the layer a high biomimicry creating a structure very similar to bone, but with a disordered and less porous structure, suitable for anchoring the tooth to the alveolus. The introduction of FeHA confers to the biomaterial a magnetic property, normally not present in nature, but is assessed to be useful for improving the regeneration capability of the biomaterial by the activation of cell processes in hard tissue regeneration.
Layers were assembled all together before freeze-drying because the presence of water improves the layers’ integration minimizing their delamination. In conclusion a three-layers device was developed by changing for each layer, the composition, degree of mineralization, morphology and fabrication technology in order to mimic the different tissues of periodontal region and obtaining a biocompatible and bioactive construct with instructive abilities for cells due to its multi-scale structural features (Fig. 8.2A) (Sprio et al. 2018).
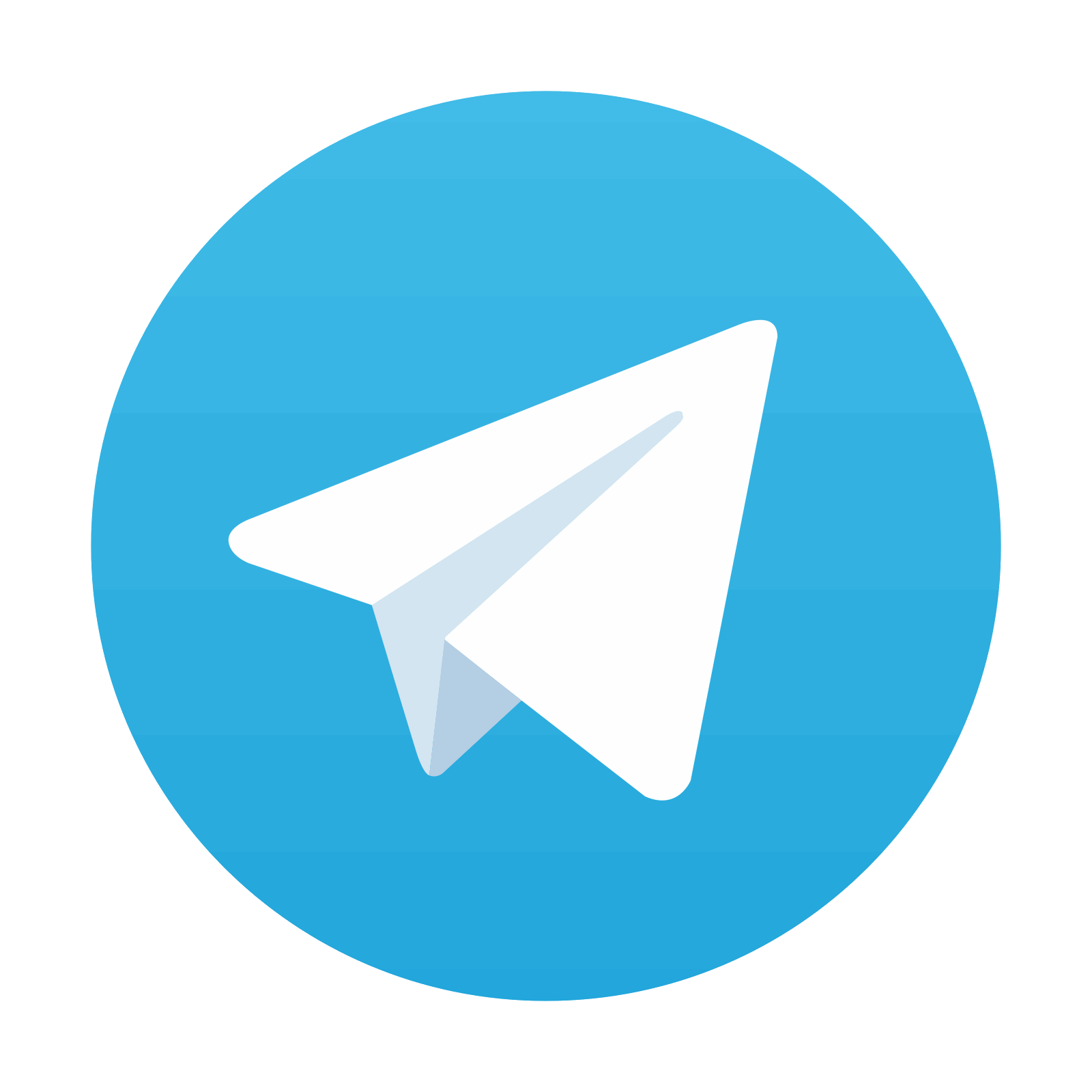
Stay updated, free dental videos. Join our Telegram channel

VIDEdental - Online dental courses
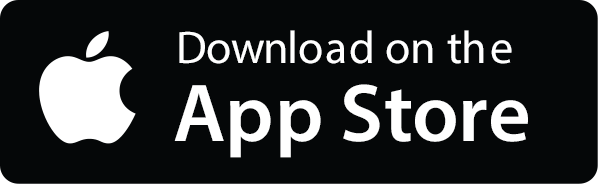
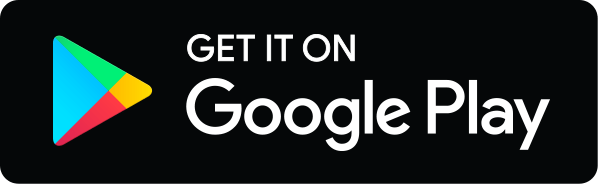