Fig. 3.1
Biomechanical considerations in zygomatic implant application
The use of tilted or angled implants particularly in the posterior region to treat atrophic maxilla patients showed an increase with short and medium term clinical success [25, 26]. Several clinical follow-up studies stated that the survival rates of angled implants placed in posterior maxilla were from 92.8 to 98.8 % in combination with the use of axial implants under delay loading [26, 27]. The application of zygomatic implant has a similar concept with the use of distal implant in particular inclination to restore and rehabilitate the prosthetic functions. The rationale behind the inclination of distal implant is based on biomechanical viewpoint, where the cantilever length can be reduced and the polygonal area for prosthetic support will be increased.
Other than that, longer implants can be used to increase the surface area contact with bone tissues for a better osseointegration. The anchorage of implant to bone with more than one cortical plate will increase the initial implant stability [26, 28]. There was an experimental study done by Geremia et al. and the results showed that a better general biomechanical behaviour of tilted distal implant was observed when compared with straight implant for the same cantilever extension [25]. The installation of posterior implant in distal direction parallel to the anterior sinus wall is required to avoid the penetration of implant body through sinus mucosa and to provide better implant retentions. The zygoma was claimed to absorb most of the posterior loads while the conventional dental implants in the premaxillary region responsible to the anterior loads [26]. This could therefore reduce the adverse masticatory loading and cantilever effects on the zygomatic implant body.
3.4.1 Previous Biomechanical Study of Zygomatic Implants
Ujigawa et al. conducted a study on zygomatic implants to measure the stress distribution within craniofacial structures and superstructure itself through 3D finite element method [29]. The study had compared the configuration of zygomatic implants with and without connected implants to support the superstructure. The outcome of study showed that most of stresses were sustained by the zygomatic bone, at the middle part of the implant body and at the connection of implant-abutment for the case without connected implants. For the case with connected implants, there were less stresses concentrated within the alveolar bone around the head of zygomatic implant. The occlusal loading was primarily sustained by the zygoma and the stresses were dispersed into temporal and frontal processes.
The evaluation of zygomatic implants in the unilateral maxillary defect restoration was done by Wu et al. in 2008. The aim of the study was to investigate the biomechanical criteria and functional design of the unilateral maxillary reconstruction method using the microsurgery bone graft and zygomatic implant [30]. The results found that the highest stress magnitude was generated at the neck of implant on the zygomatic bone whilst the rest of peri-implant bone was observed to sustain lower stress values. The study also concluded that the role of zygomatic implant is important as it will transmit the occlusal forces substituting the zygomatic process brace [30].
The role of zygomatic implants in the treatment of maxillectomy patients was continued to be investigated by Miyamoto et al. in 2010 [31]. In the study, the maxillary prosthesis with different positions and numbers of standard dental implants were examined through 3D FEA. Maxillary prosthesis was designed with two zygomatic implants placed on the affected side and two to three standard implants in the maxillary alveolar bone on the unaffected side. The result of analysis demonstrated that zygomatic implants provided suitable stress dispersal to the zygomatic and craniofacial bone on the affected side.
3.5 Finite Element Analysis in Dentistry
Various methods or analyses exist and can be applied to investigate the biomechanical criteria in the field of dentistry like the mechanical stress and strain distribution within implants and bones. Photoelastic model studies, strain gauge analysis and FEA are among the methods that are broadly available to be conducted on [1, 32]. In the photoelastic model study, the results obtained are recorded photographically using a high-resolution camera in the field of a circular polariscope [33]. The values of stress and its locations will be evaluated visually and subjectively. One of the disadvantages of photoelastic study is the normal stresses within the material simulating the bone cannot be detected. Furthermore, the isotropic photoelastic material simulates the orthotropic material (bone).
The stress distribution within bone can also be evaluated using the strain gauge analysis. The strain gauge analysis involves the use of strain transducers that placed on the prosthesis or overdenture surfaces connected to the implant bodies [22]. This analysis can measure the relative forces on restoration and simulates more complex structure than in the photoelastic study. Moreover, the additional experimental information such as stress types and strain qualification can also be found through this analysis. If a study gives a high focus on system geometry and mechanical properties of biologic tissues, a 2D or 3D FEA is the best option to be chosen. However, the 2D FEA is not preferable because of invalidity of models representation [34, 35]. As instance, the 2D FEA fails to distinguish between cortical and cancellous bone. Therefore, the results from 2D FEA cannot be used extensively as a prediction tool because they show a significant difference to represent the realistic situation.
In FEA, researchers can predict the behaviour or pattern of stress distribution at bone-implant interface as well as around the apical part of implant body in cancellous bone [14]. Moreover, through FEA, the complicated geometry of bones or implants can be modelled and solved, in which cannot be done by analytical solution. A complex mechanical problem can be solved into several collections of much smaller and simpler domains or elements by using FEA. The shape function is used to explain and interpolate the field variables. Finite element analysis that is also known as a prediction tool of the models tested, which the approximated results obtained to the basic problem are determined based on variational principles. In other words, the whole body or entire domain solution provided by FEA is based on the combination of solution functions for each finite element. Therefore, since a dental implant-bone system consists of several components that are extremely complex geometrically, FEA is believed can provide as an appropriate solution to the models tested [36–39]. There is a necessity to divide the whole domain into smaller elements by having a meshing process for the execution of analysis in the finite element method. Besides the mesh, elements and nodes creation, the other processes like boundary conditions determination is referred to as “discretization” of the problem domain [14].
Finite element analysis was introduced since 1960s to solve the structural problems in aerospace industry; however, it has been expanded in use in other fields like heat transfer, electromagnet, mass transport and fluid flow. The first application of FEA in implant dentistry started in 1976 by Weinstein et al. [14, 40]. Ever since, FEA was widely applied in that field.
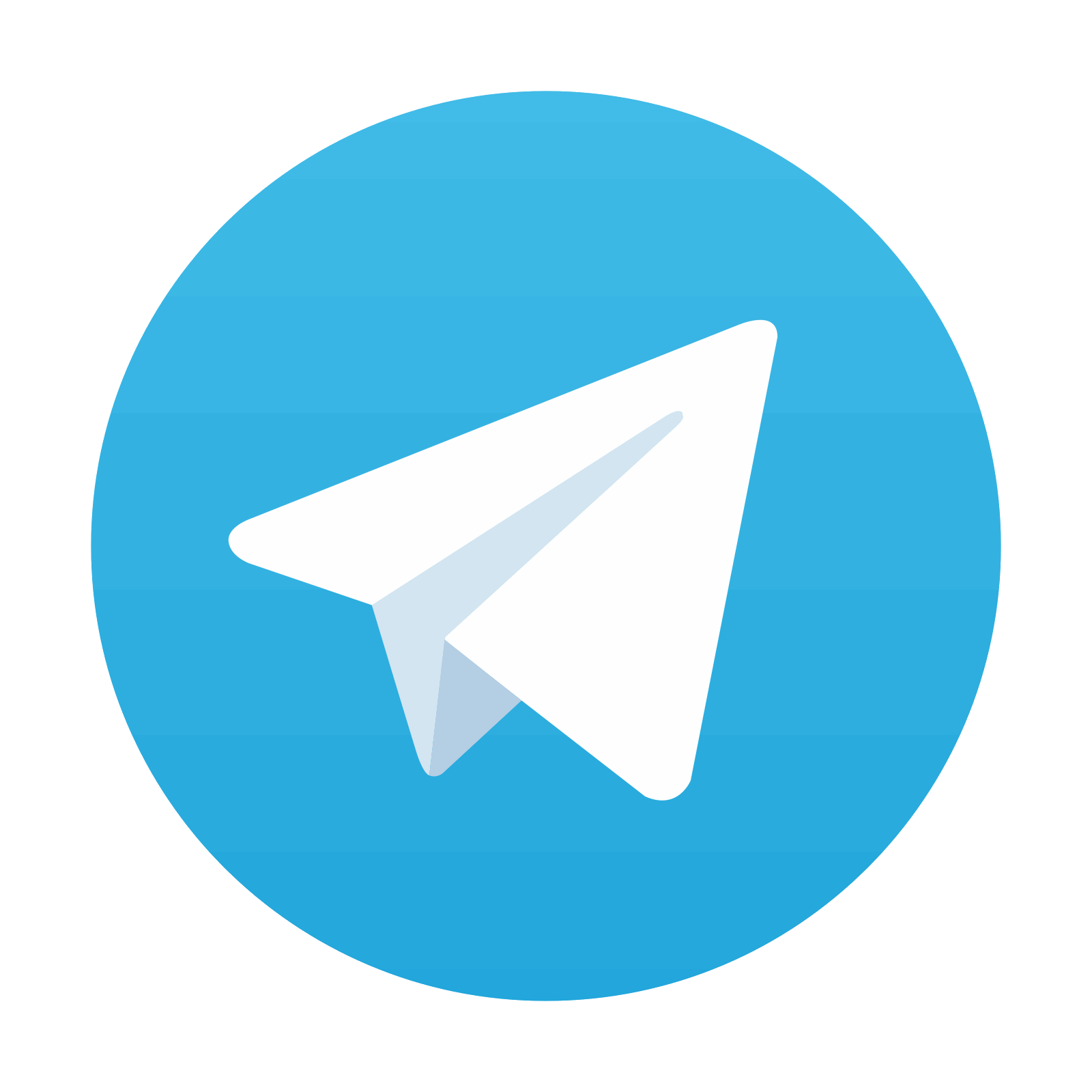
Stay updated, free dental videos. Join our Telegram channel

VIDEdental - Online dental courses
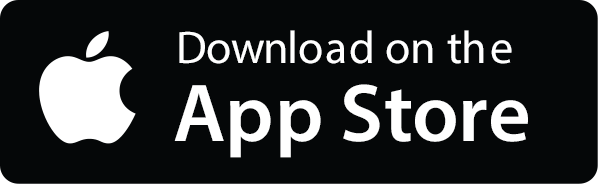
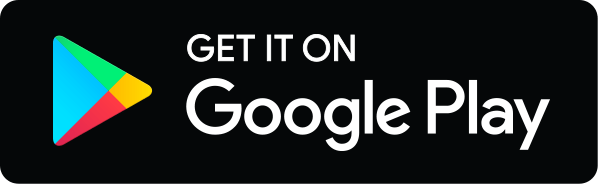