Composition (wt%)
45S5 Bioglass (NovaBone, PerioGlas)
S53P4 (AbminDent1)
A-W glass-ceramic (Cerabone)
Na2O
24.5
23
0
CaO
24.5
20
44.7
CaF2
0
0
0.5
MgO
0
0
4.6
P2O5
6
4
16.2
SiO2
45
53
34
Phases
Glass
Glass
Apatite beta-wollastonite glass
Class of bioactivity
A
B
B
The glass implants bonded to the living bone (rat femora) within 6 weeks and could not be removed from their implant site [1–3]. This discovery led to the development of a new class of biomaterials, called bioactive materials, for use in implants or prostheses and repair or replacement of bones, joints, and teeth. Table 3.2 summarizes the chronology of the development of bioactive glasses as a second generation of biomaterials. The seminal paper describes the composition of the glass and the evidence of bonding to bone by use of transmission electron microscopy (TEM) that reveals the bonded interface as a layer of growing Ca-P-based bone mineral that has interdigitated with collagen fibrils generated by osteoblasts growing at the interface [1]. An acellular in vitro model of soaking the glass samples in a 37 °C calcium phosphate-rich solution showed by X-ray diffraction (XRD) the steps of growth of a biologically active hydroxyapatite crystal phase on the surface of the glass that mimics the XRD pattern of the HA phase in living bone. Details of the bone-bonded interface are described in additional papers in 1971–1972 [1, 2].
Table 3.2
Chronology of science and clinical product development of 45S5 Bioglass
Discovery of bone bonding to 45S5 Bioglass at the University of Florida
|
|
---|---|
1971
|
|
1972
|
Bonding of Bioglass bone segments and coated femoral stems in monkeys
|
1975
|
Bioglass-coated alumina bone bonding to sheep hip implants (in Germany)
|
1975
|
Bioglass dental implants bonded in baboon jaws
|
1977
|
Bonding of Bioglass implant in guinea pig middle ear
|
1977
|
Patent applications filed for Bioglass coatings on metals and alumina ceramics
|
1981
|
Discovery of soft connective tissue bonding to 45S5 Bioglass
|
1981
|
Toxicology and biocompatibility studies (20 in vitro and in vivo) published to establish safety for FDA clearance of Bioglass products
|
1985
|
First medical product (Bioglass Ossicular Reconstruction Prosthesis) (MEP) cleared by FDA via the 510 (k) process
|
1987
|
Discovery of osteoproduction (osteostimulation) in use of Bioglass particulate in repair of periodontal defects
|
1988
|
Bioglass endosseous ridge maintenance implant (ERMI) cleared by FDA via the 510 (k) process
|
1991
|
Development of sol-gel processing method for making bioactive gel-glasses extending the bioactive compositional range of bioactivity
|
1993
|
Bioglass particulate for use in bone grafting to restore bone loss from periodontal disease in infrabony defects (PerioGlas) cleared by FDA via the 510 (k) process
|
1995
|
PerioGlas obtained CE Mark in Europe
|
1996
|
Use of PerioGlas for bone grafts in tooth extraction sites and alveolar ridge augmentation cleared by FDA via the 510 (k) process
|
1999
|
European use of 45S5 particulate for orthopedic bone grafting (NovaBone)
|
2000
|
FDA clearance for use of NovaBone in general orthopedic bone grafting in non-load-bearing sites
|
2000
|
Quantitative comparison of rate of trabecular bone formation in the presence of Bioglass granules versus synthetic HA and A/W glass-ceramic
|
2000
|
Analysis of use of 45S5 Bioglass ionic dissolution products to control osteoblast cell cycles
|
2001
|
Gene expression profiling of 45S5 Bioglass ionic dissolution products to enhance osteogenesis
|
2004
|
FDA clearance of 45S5 particulate for use in dentinal hypersensitivity treatment (NovaMin)
|
2005
|
Development of variety of dental maintenance products (NovaMin)
|
2009
|
Anniversary of one million doses of NovaBone bone graft product and one million tubes of NovaMin toothpaste
|
2011
|
Acquisition of NovaMin technology by GlaxoSmithKline and world launch of Sensodyne Repair and Protect toothpaste for prevention of dentinal hypersensitivity and gingivitis
|
3.2.1 Compositions of Bioactive Glasses
Bioactive materials, including bioactive glasses [1–3] and glass-ceramics [4–6], are special compositions made typically from the Na2O-CaO-MgO-P2O5-SiO2 system (Table 3.1). All of the compositions in Table 3.1 form a mechanically strong bond with bone within weeks or months. Details are described in references [7–15]. The rate of bone bonding depends upon composition of the material. Glass compositions with lower contents of SiO2 (<52 % by weight) have the fastest rates of bone bonding and also bond to soft tissues [16]. Compositions such as 45S5 Bioglass with high rates of bioactivity produce rapid regeneration of trabecular bone with an amount, architecture, and biomechanical quality of bone that match that originally present in the site [14, 17–19]. The rapid regeneration of bone is due to a combination of processes called osteostimulation and osteoconduction [16–19]. Large differences in rates of in vivo bone regeneration and extent of bone repair indicate that there are two classes of bioactive materials (Table 3.1) [16–19].
3.2.2 Classes of Bioactivity
Class A bioactivity leads to osteoconduction, growth of new bone along the bioactive interface, and osteostimulation, growth of new bone throughout the defect to regenerate the architecture of bone natural to the site, as a consequence of rapid reactions on the bioactive glass surface [16–19]. The surface reactions involve dissolution of critical concentrations of soluble Si and Ca ions that give rise to both intracellular and extracellular responses at the interface of the glass with its physiological environment. The intracellular and extracellular response of osteoprogenitor cells results in rapid formation of osteoid bridges between particles, followed by mineralization to produce mature bone structures. This process is termed osteostimulation or osteoproduction. Rates of osteostimulation of various types of bioactive particulates have been quantified by Oonishi et al. that provide the fundamental in vivo comparisons of Class A versus Class B bioactive materials [14–19].
3.2.2.1 Characterization of Bioactivity Reaction Stages
New surface and interfacial analyses techniques were developed to understand the mechanisms and kinetics of bioactive reactions in vitro and in vivo [1, 13, 20–23]. These methods showed that there is a sequence of 11 reaction stages that occur at the surface of a Class A bioactive glass. Figure 3.1 indicates in the log time axis that the first five stages of surface reactions occur very rapidly and go to completion within 24 h for the bioactive glasses with highest levels of Class A bioactivity, e.g., 45S5 Bioglass. The effect of the surface reactions is rapid release of soluble ionic species from the glass into the interfacial solution. A high surface area composed of hydrated silica and polycrystalline hydroxyl carbonate apatite (HCA) as a bilayer is formed on the glass surface within hours (Stages 1–5) [23]. The reaction layers enhance adsorption and desorption of growth factors (Stage 6) and decrease the length of time macrophages prepare the implant site for tissue repair (Stage 7).
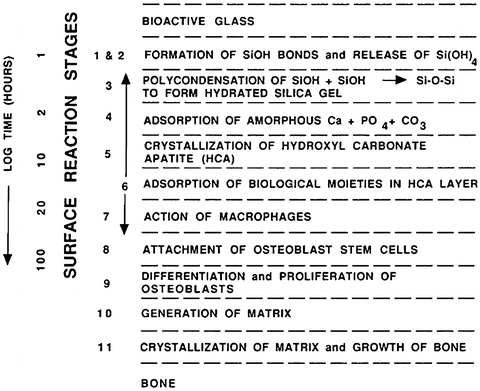
Fig. 3.1
Sequence and kinetics of interfacial reactions between bones and a Class A bioactive glass or glass-ceramic (Reprinted with permission from Hench [19], American Ceramic Society)
Attachment of stem cells (Stage 8) and synchronized proliferation and differentiation of the progenitor cells (Stage 9) rapidly occur on the surface of Class A bioactive materials [10, 14–16, 24]. Several weeks or months are required for similar cellular events to occur on the surface of Class B bioactive materials. Differentiation of progenitor cells into a mature osteoblast phenotype does not occur on bio-inert materials and is rare on Class B bioactive materials because of the lack of ionic stimuli. In contrast, osteoprogenitor cells colonize the surface of Class A bioactive materials within 24–48 h and begin production of various growth factors which stimulate cell division, mitosis, and production of extracellular matrix proteins (Stage 10). Mineralization of the matrix follows soon thereafter, and mature osteocytes, encased in a collagen-HCA matrix, are the final product by 6–12 days in vitro and in vivo (Stage 11) [14–16, 24–30].
3.3 Genetic Control of Bone Regeneration by Bioactive Glasses
The original discovery of bioactive glasses and emphasis of research were on the mechanisms of interfacial bonding of bone to bioactive glasses [1–3, 7–22]. The seminal step to shift thinking from bioactive bonding to bone regeneration was the reporting by June Wilson et al. for the first time that new bone had colonized the surface of an array of 45S5 Bioglass particulate placed in a surgically created site in the jaw of monkeys that mimicked the loss of bone from periodontal disease [31]. New bone formed around the particles and created a regenerated architecture of bone that bridged the bioactive glass particles (Fig. 3.2). The new phenomenon was designated as osteoproduction, now generally called osteostimulation. The concept of osteostimulation was quantified by Oonishi et al. a few years later [14, 32]. The second key finding to develop a genetic basis for bone regeneration was the finding of Xynos et al. that it was not only the glass but it was the ionic dissolution products released from 45S5 Bioglass that influenced and controlled the cell cycle of osteogenic precursor cells and ultimately controlled the differentiated cell population [26, 27]. Cells that were not capable of achieving a fully differentiated phenotype characteristic of mature osteocytes were eliminated from the in vitro cultures by programmed cell death, apoptosis. The shift in cell population towards mature osteoblasts occurred rapidly, in hours, and led to mineralized bone nodules in culture, without addition of organic bone growth factors, such as bone morphogenetic proteins (BMP). Another key finding from the work of Xynos et al. was that the effective ionic dissolution products released at slow rates from 45S5 Bioglass were biologically active soluble Si and Ca ions. Both ions are necessary for osteostimulation. Osteostimulation occurs only when the ions are present at a particular ratio of ions and at a particular concentration range of 15–30 ppm Si and 60–90 ppm Ca.
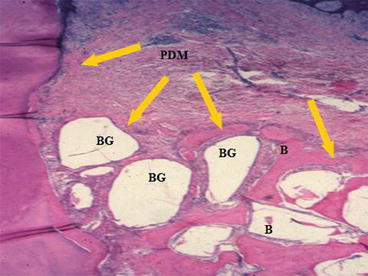
Fig. 3.2
Bioactive glass BG particulate used to enhance bone [B] formation around the tooth [T] and thereby restores its function following treatment of periodontal, gum, disease in a patas monkey periodontal defect model. Note the mineralized collagen that surrounds the bioactive glass particles forming a regenerated bone structure. Also note the periodontal ligament labeled PDM that is stable above the regenerated bone. (Magnification 20x). (Photo courtesy of Dr. June Wilson)
These findings provide an understanding of the clinical success of use of 45S5 Bioglass particles, NovaBone and PerioGlas, in a wide range of dental and orthopedic applications in use for nearly 20 years, as discussed in [17–19]. When used as a synthetic bone graft, the bioactive glass particles dissolve slowly and release the critical concentrations of Si and Ca over many weeks, as needed for progressive regeneration of bone to fill the defect. The initial effect is proliferation of osteogenic precursor cells at the periphery of the particles. These cells undergo mitosis and lead to an expanded population of mature osteoblasts that generate extracellular matrix proteins, especially Type I collagen that mineralizes to form regenerated bone (Fig. 3.3).
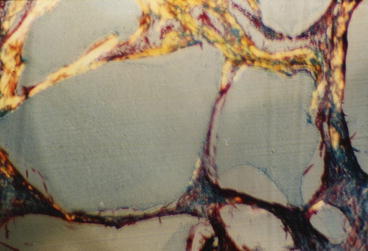
Fig. 3.3
Higher magnification of collagen fibrils that have been formed and adherent to bioactive glass particles in a regenerated periodontal defect model equivalent to that shown in Fig. 3.2. (Magnification 100x). (Photo courtesy of June Wilson)
Oonishi et al. used a critical size defect model in a rabbit femoral condyle model to quantify the histological sequence of osteostimulation by 45S5 Bioglass particulate [14, 32]. The studies show that there is both more rapid bone formation in the presence of the osteostimulation particles and regeneration of a more highly mineralized quality of bone in the defect, in comparison with synthetic hydroxyapatite (HA) particles or bioactive A/W glass-ceramic particles. The rate of bone regeneration in the Oonishi model is related to the rate of release of the soluble Si and Ca ions from the particulates tested. A rapid rate of release, as depicted in Fig. 3.1 Stages 1–5, is necessary for rapid bone regeneration and optimal fill of the defect by regenerated trabecular bone.
The third critical step in developing a genetic basis for tissue regeneration was the discovery that critical concentrations of ionic dissolution products (soluble Si and Ca ions) activate or upregulate seven families of genes in osteogenic precursor cells [27–30]. The genes encode transcription of numerous proteins that control the cell cycle, proliferation, and ultimately the differentiation of the cells towards the mature osteoblastic phenotype. The seminal paper leading to identification of the genetic response to bioactive dissolution products was published by Xynos et al. in 2001 resulting from a collaboration at Imperial College London of biomaterials investigators (the author’s group) and the cell and molecular biology groups of Professor Dame Julia Polak [27].
3.3.1 Confirmation of Genetic Control of Bone Regeneration
Numerous studies have confirmed the results of the early Xynos et al. research and extended the generality to include several types of precursor cells and differing sources of ionic stimuli. Gene array analyses of five different in vitro models using five different sources of inorganic ions provide the experimental evidence for a genetic theory of osteogenic stimulation [26–30, 33–36]. The cell and organ culture models are summarized in Table 3.3. Sources of the ionic stimuli are given in Table 3.4.
Cell and organ culture
|
|
---|---|
Model # 1
|
Primary human osteoblasts (pHOBs)
|
Model # 2
|
Fetal human osteoblasts (fHOBs)
|
Model # 3
|
Murine embryonic stem cells (mES)
|
Model # 4
|
Human embryonic stem cells (hES)
|
Model # 5
|
Murine fetal long bones (metatarsals) (mFLBs)
|
Source of ionic dissolution products
|
|
---|---|
Source A
|
45S5 bioactive glass culture discs
|
Source B
|
4555 bioactive glass particulate (NovaBone)
|
Source C
|
58S bioactive gel-glass
|
Source D
|
70/30 bioactive gel-glass 3D porous tissue engineering scaffolds
|
Source E
|
Ionic dissolution products of B, C, D.
|
In Table 3.4 the composition of the melt-derived 45S5 bioactive glass culture discs (A) and particulate (B) was 45 % (by weight) SiO2, 24.5 % CaO, 24.5 % Na20, and 6 % P2O5 [1]. Samples of (A) were obtained from US Biomaterials Corp., Alachua, FL, from a certified batch. Commercial powders of (B) with a particle size of 90–710 pm were obtained from NovaBone Products, Alachua, FL. The 58S sol-gel-derived particulate (C) composition (58 % SiO2, 36 % CaO, 6 % P205) and the 70/30 sol-gel sample (D) composition (70 % SiO2, 30 % CaO) were made by the Dept. of Materials, Imperial College London [27, 37]. Sample (E) and the ionic dissolution products of (B), (C), and (D) were obtained by immersing particulates of (B), (C), and (D) in simulated body fluid solution at 37 °C for various times to achieve concentrations of 15–30 ppm of soluble Si ions and 60–90 ppm of soluble Ca ions [38–40].
A study of dose dependence of ionic dissolution products showed this range of concentrations led to enhanced proliferation of osteoblasts [33–41]. Human primary osteoblasts (Table 3.3 Model #1) were obtained from excised femoral heads of total hip arthroplasty patients aged 50–70 years [24]. The first cell cycle and gene array experiments compared samples (A) with Thermanox plastic controls; the 2nd experiment compared ionic dissolution products of (B) with Thermanox controls; and experiment 3 used PCR methods to confirm effects of the ionic dissolution products of (B) on expression of specific genes from osteoblasts obtained from excised femoral heads of five individual patients [26]. Student’s t tests were used to determine statistical significance of the results. The 4th and 5th experiments tested the effects of sample (E) on fHOBS and hES cells. The 6th and 7th experiments confirmed the findings of experiments 1–5 by comparing dosage effects of samples A and E on murine fetal metatarsals grown for 4 days in organ culture post-day 14 gestation [28] and growth of primary hOBs within three-dimensional scaffolds (Source D) [24].
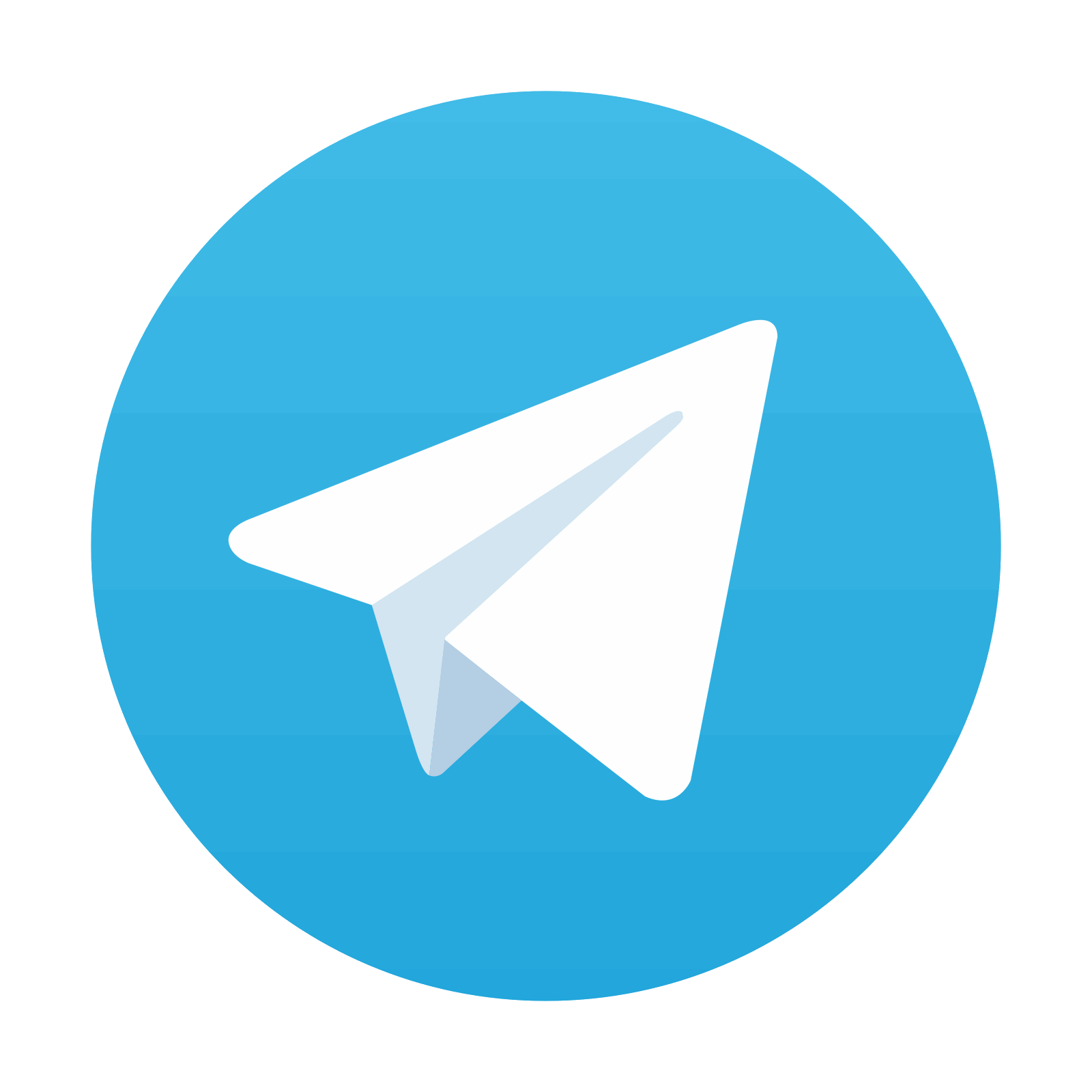
Stay updated, free dental videos. Join our Telegram channel

VIDEdental - Online dental courses
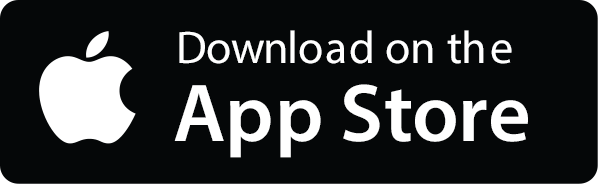
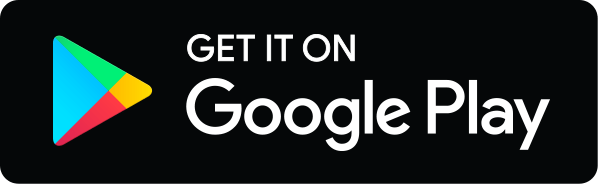