Species
Advantages
Limitations
Mice
Ease of genetic manipulation
Lack of intracortical remodeling
Small size for surgical manipulation
Rats
Most widely used model of OVX-induced bone loss
Lack of intracortical remodeling
Large animals (dogs, pigs, sheep)
Intracortical remodeling
Expensive
Large size
Limited/variable response to OVX
Biopsies possible
Nonhuman primates
Intracortical remodeling
Expensive
OVX-induced bone loss
Limited access for many labs
Large size
Biopsies possible
Large Animal Models of Osteonecrosis of the Jaw
The first reports of oral bone necrosis associated with bisphosphonate treatment came from an experiment in our laboratory that was focused on investigating how bisphosphonates affect bone mechanical properties [13, 14]. The design of these experiments was such that skeletally mature female beagle dogs were treated with daily oral alendronate at a dose consistent with that used for treatment of postmenopausal osteoporosis (on a mg/kg basis) or a dose 5× higher (approximating the dose used in Paget’s disease). An age-matched control treated with oral saline was also part of the experiment. Groups of dogs were treated for 1, 2, or 3 years in duration. Although the effects of alendronate on the oral skeleton were not part of original analysis plan, the mandibles were saved “just in case” at the recommendation of a collaborator.
Following several years of clinical ONJ reports, we began to investigate the utility of our stored tissue to understand ONJ. Historical precedence existed for studying bone necrosis histologically. Using basic fuchsin staining, which our lab was quite familiar with based on our interest in microdamage, regions of necrotic bone could be histologically identified by an absence of stain penetration [15]. Using this method, we showed that animals treated with oral alendronate for three years had a significantly higher incidence of matrix necrosis within the mandible compared to vehicle-treated controls [7]. Our sampling method, which examined four different regions of bone throughout the mandible, revealed no regions of nonviable matrix in vehicle animals while 30 % of alendronate-treated animals had at least one necrotic region. Necrotic regions, which represented areas lacking viable lacuna-canalicular networks (Fig. 15.1), were fundamentally different from what was being seen clinically with ONJ – exposed bone in the oral cavity. Follow-up analyses of animals that had been treated for 1 year with these same doses of oral alendronate showed a similar pattern of matrix necrosis with alendronate-treated animals, but not vehicle controls, having regions of nonviable bone in the mandible matrix [16]. While the matrix necrosis did not match the clinical manifestation of ONJ, it was hypothesized that these necrotic regions could be the early stages of bone death that would eventually manifest into exposed bone if combined with other factors. These studies also documented, for the first time, that daily oral alendronate at clinically meaningful doses significantly suppressed intracortical remodeling within the mandible cortex. This was important as suppression of remodeling within the oral cortical bone, which normally occurs at a high rate, was hypothesized to be a key factor in the etiology of ONJ but had not been previously shown to occur in a model with intracortical remodeling.
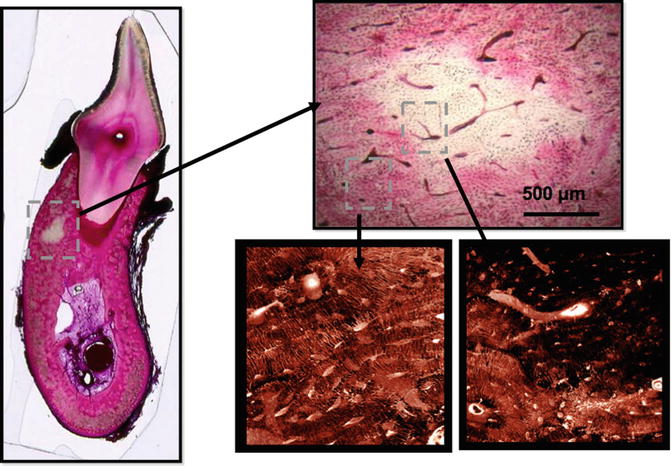
Fig. 15.1
Bone matrix necrosis associated with bisphosphonate treatment in beagle dogs. Following 1 or 3 years of treatment, basic fuchsin staining of samples revealed regions of nonviable bone that were notably absent in control animals. Regions of nonviable bone appear white in fuchsin-stained bone due to the absence of penetration of the stain into regions that lack patent canaliculi. These can be observed using either bright-field or confocal microscopy (Adapted from Allen and Burr [7])
Subsequent to these experiments, our group designed a series of studies to further investigate the utility of the beagle dog as a model for ONJ. As the majority of the emerging ONJ reports were in patients treated with bisphosphonate doses consistent with those in cancer patients (intravenous zoledronic acid), we asked the question of whether there was a difference in matrix necrosis between IV zoledronic acid and oral alendronate. In short-term experiments (3 and 6 months), we showed that while IV zoledronic acid produced a more rapid and profound suppression of remodeling in the mandible compared to oral alendronate, neither agent produced regions of bone matrix necrosis (assessed using basic fuchsin staining) [17]. The working hypothesis that emerged from this study was that the matrix necrosis necessitated longer than 6 months to manifest even in the face of potent remodeling suppression such as occurs with intravenous dosing regimens for cancer treatment.
Two additional experiments aimed at understanding the interaction between bisphosphonates and dental extraction. Early clinical reports suggested (and subsequent reports have strongly confirmed) that dental intervention precipitated exposed bone in patients at a significantly higher rate than occurred spontaneously. In an acute experiment (3 months in duration), we observed one case of exposed bone in animals treated with IV zoledronic acid and then subjected to dental extraction [18]. This exposed bone matched several of the clinical criteria for ONJ including the production of a sequestrum (Fig. 15.2). Our follow-up experiment, where animals were treated for 7 months with IV zoledronic acid prior to dental extraction (9 months’ total experimental duration), failed to produce exposed bone in any animal, even in those animals in which zoledronic acid was combined with dexamethasone (a common cofactor in clinical ONJ) [19]. Despite the lack of exposed bone, high-resolution CT revealed two animals with high-dose zoledronic acid had significantly compromised extraction socket healing. Furthermore, the majority of animals treated with bisphosphonates, but not those treated with vehicle, had periosteal reaction (new bone formation on the periosteal surface) associated with the extraction site. Similar to our earlier studies, these extraction studies revealed that IV zoledronic acid potently suppressed (and in some cases completely abolished) cortical bone remodeling in the mandible.
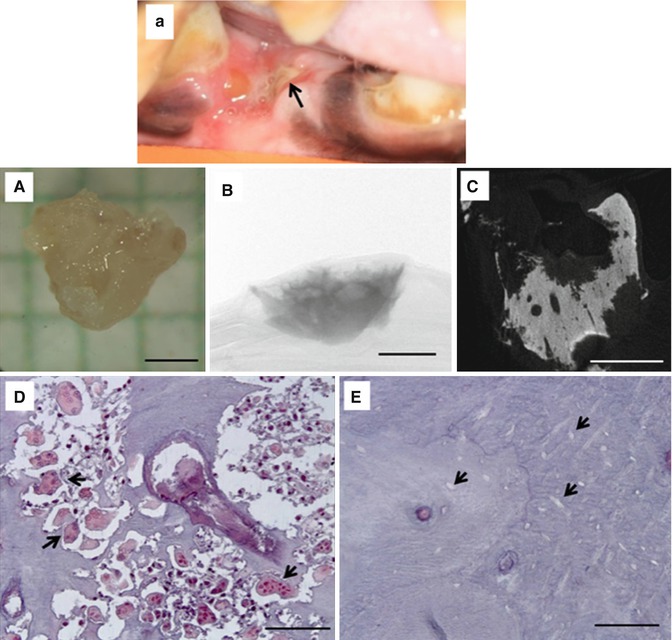
Fig. 15.2
Exposed bone in a zoledronate-treated beagle dog following dental extraction (a). The exposed bone eventually formed a sequestrum. (A) Photograph; (B) two-dimensional micro-CT image through the center of the sequestrum showing highly scalloped surfaces; (C) two-dimensional micro-CT projection image of the entire specimen; (D) photomicrograph of histological section through the sequestrum stained with tartrate-resistant acid phosphatase (TRAP) to visualize osteoclasts (select, but not all, osteoclasts identified with black arrowheads); (E) photomicrograph of histological section through the sequestrum stained with TRAP showing empty osteocyte lacunae (select, but not all, empty osteocyte lacunae identified with black arrowheads). Scale bars = 1 mm for (A–C) and 100 μm for (D, E) (Reproduced with permission from Allen et al. [18])
A handful of other experiments have investigated the interaction between bisphosphonates and the oral skeleton in dogs, although not always focused on producing ONJ. A small-scale experiment was conducted to look at extraction healing in the presence of zoledronic acid with or without bone morphogenic protein treatment [20]. These results showed significant bisphosphonate effects on remodeling and healing but made no measures of necrotic bone. In a similar way, studies examining extraction and/or implant healing in animals treated with zoledronic acid failed to produced exposed bone, although remodeling suppression and matrix necrosis (assessed using lactate dehydrogenase histochemistry) were both noted in treated animals [21, 22]. Collectively, these studies in a dog model produce a consistent picture of potent suppression of intracortical remodeling and negatively affect osseous healing. However, the model does not produce a robust manifestation of exposed bone.
The other large animal species investigated as a model of ONJ is the swine with two reports showing manifestation of exposed bone. Skeletally mature male Gottingen mini pigs were treated with either weekly zoledronic acid or vehicle for 16 weeks with multiple dental extractions (6 in total) at week 6 [23]. All bisphosphonate-treated animals developed exposed bone at three or more of the extraction sockets, while the vehicle animals all had epithelial coverage within 2 weeks (Fig. 15.3). This study conducted a robust analysis of several factors related to necrosis – effectively showing visual, histological, and CT-based evidence of ONJ. This represents the most thoroughly documented evidence of clinically consistent ONJ in a large animal model. A second study, using a different strain of swine (Wuzhishan mini pigs), also documented the development of exposed bone when zoledronic acid treatment was superimposed with extraction [24]. Again, control animals showed complete healing at the time point assessed. Neither of these studies measured intracortical bone turnover rates nor commented on the amount of osteonal bone within the mandible tissue of mini pigs.
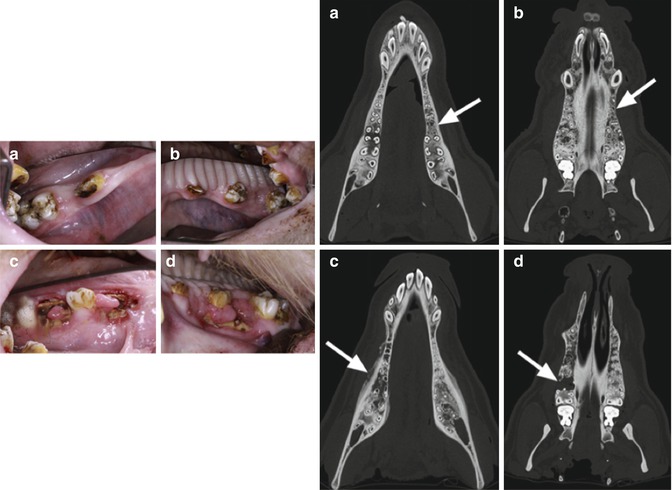
Fig. 15.3
Exposed bone and disrupted healing post-extraction in a mini pig model. Intraoral views of the animals 10 weeks after tooth extractions with uneventful wound healing in the control group (a: mandible; b: maxilla). In contrast, all animals in the bisphosphonate group showed impaired healing and exposed bone (c: mandible; d: maxilla). Computed tomography images (axial views) of the lower and upper jaw of animals in the control group (a, b) as well as of the bisphosphonate group (c, d). The extraction sites are indicated by white arrows. Bony healing and no periosteal reaction were observed in the control animals (a, b). In contrast, the bisphosphonate group exhibited no bone remodeling of extraction sockets, and typical radiological hallmarks of a bisphosphonate-related osteonecrosis were observed (cortical erosion, periosteal calcification, hypo- and hyperdensities) (c, d) (Reproduced with permission from Pautke et al. [23])
Although rabbits are not necessarily a “large animal,” they are often grouped in this category for skeletal research because like other larger animals (and different from rodents), they undergo intracortical remodeling. There are no published reports focused on ONJ in rabbits, although the rabbit is a common model for studying dental perturbations such as distraction osteogenesis. A potentially interesting aspect of rabbits is that although they do undergo intracortical remodeling, qualitative analysis revealed it was not common in the mandible [16].
The underlying mechanism driving the disconnect between the two large animal models, canine and swine, with respect to producing exposed bone following bisphosphonate/dental extraction is not clear. Doses of bisphosphonates were roughly comparable between the studies in these two models, as was the time post-extraction that the animals were assessed. Yet, out of confusion arises opportunity. The disparate results in these two large animal models set the stage for potentially useful comparative studies, examining either bone/mucosal-specific factors or bacterial profiles in the oral cavity that differ between mini pigs and dogs. Such factors that exist in swine but not dogs may provide insight into key factors involved in the manifestation of exposed bone in the setting of bisphosphonates.
Rodent Models of Osteonecrosis of the Jaw
In 1981, Gotcher and Jee produced, in retrospect, the first animal model of ONJ [25]. They treated young, rapidly growing rats with high doses of clodronate for up to 18 weeks and observed bone protruding into the oral cavity that upon histological analysis was found to be void of viable cells and considered devitalized. These studies utilized the rice rat, a model known for developing periodontal disease. Interestingly, the exposed bone occurred in the absence of any dental intervention. Because the animals were young and received high doses of drug, the results were, at the time, considered to have limited clinical relevance.
Nearly three decades later, the first animal model to emerge following the clinical description of ONJ was published. Sonis and colleagues designed their study specifically to try and produce changes in the oral cavity consistent with the clinical and radiographic criteria of ONJ [8]. Three-month-old rats were treated with a combination of zoledronic acid and dexamethasone and then subjected to extraction of three molars in the mandible or maxilla. The results were striking (Fig. 15.4), with many treated animals developing mucosal ulceration at the extraction sites, and those treated with both drugs had persistent ulcerations even 28 days postsurgery. Despite the oral examination showing clear lack of healing in treated animals, most of the other analyses (radiographic, histological) were qualitative. Yet this study is considered one of the most of important preclinical contributions to the field of ONJ as it provided a foundation for future work in animals.
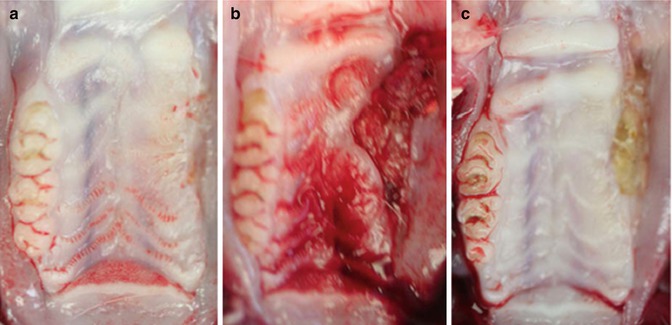
Fig. 15.4
Exposed bone following dental extraction in the first rat model of ONJ. Representative photographs of gross clinical appearance of maxillary ridges in animals with intact epithelium (a, day 28 following extraction) or ulcerated mucosa overlying necrotic bone at days 14 (b) and 28 (c) following extraction. Ulcerative areas were characterized by rolled mucosa, lack of drainage, and, by day 28 post-extraction, central areas of yellow/gray necrosis (Reproduced with permission from Sonis et al. [8])
Since the work of Sonis and colleagues, over three dozen publications have described how bisphosphonates, with or without dental perturbation or coadministration of other drugs, lead to the manifestation (or lack) of ONJ in rodents (Table 15.2). The majority of these have utilized the rat as a model although both rats and mice have been shown to develop exposed bone and related oral manifestations. The remainder of this section will highlight some generalizations and key rodent papers, rather than detailing specific strengths/weaknesses about each study. Those interested in specifics are encouraged to use Table 15.2 as a guide to work through the relevant literature.
Table 15.2
ONJ studies in rodents
First author (year)
|
Species
|
Bisphosphonate
|
Dental insult
|
Exposed bone
|
---|---|---|---|---|
Sonis (2009)A
|
Rat
|
Zoledronate (SC)
|
All molars from one maxilla
|
Yes
|
Hikita (2009)B
|
Rat
|
Alendronate injections in oral cavity
|
Second molar from one maxilla
|
No
|
Biasotto (2010)C
|
Rat
|
Zoledronate (IV)
|
One upper molar plus an additional 4 mm defect
|
Yes
|
Hokugo (2010)D
|
Rat
|
Zoledronate (IV)
|
All molars from one maxilla
|
Yes
|
Lopez-Jornet (2010)E
|
Rat
|
Pamidronate (IP)
|
All molars from one maxilla
|
Yes
|
Maahs (2010)F
|
Rat
|
Alendronate (oral) or zoledronate (IP)
|
All molars from one maxilla
|
Yes
|
Senel (2010)G
|
Rat
|
Zoledronate (IP) or pamidronate (IP)
|
None
|
Yes
|
Aguirre (2010)H
|
Rat
|
Alendronate (SC)
|
Mandibular first molar
|
No
|
Xiong (2010)I
|
Rat
|
Alendronate (SC)
|
Periapical lesion at the mandibular first molar
|
No
|
Jee (2010)J
|
Rat
|
Alendronate (SC)
|
First molar from one maxilla
|
No
|
Kobayashi (2010)K
|
Mouse
|
Zoledronate (SC)
|
First molar from one maxilla
|
No
|
Yamashita (2010)L
|
Rat
|
Zoledronate (SC)
|
Denuded palatal mucosa between the first molar and great palatine canal
|
No
|
Kikuiri (2010)M
|
Mouse
|
Zoledronate (IV)
|
First molar from one maxilla
|
Yes
|
Bi (2010)N
|
Mouse
|
Zoledronate (IP)
|
First molar from one maxilla
|
No
|
Aghaloo (2011)O
|
Rat
|
Zoledronate (IP)
|
Ligature-induced periodontal disease
|
Yes
|
Lopez-Jornet (2011)P
|
Rat
|
Pamidronate (IP)
|
All molars from one maxilla or mandible
|
Yes
|
Marino (2012)Q
|
Rat
|
Zoledronate (IV)
|
Mandibular first molar
|
Yes
|
Aguirre (2012)R
|
Rat
|
Alendronate (SC) or zoledronate (IV)
|
Diet-induced periodontitis
|
Yes
|
Abtahi (2012)S
|
Rat
|
Alendroante
|
First molar from one maxilla
|
Yes
|
Said (2012)T
|
Rat
|
Etidroante (SC)
|
None
|
No
|
Zhao (2012)U
|
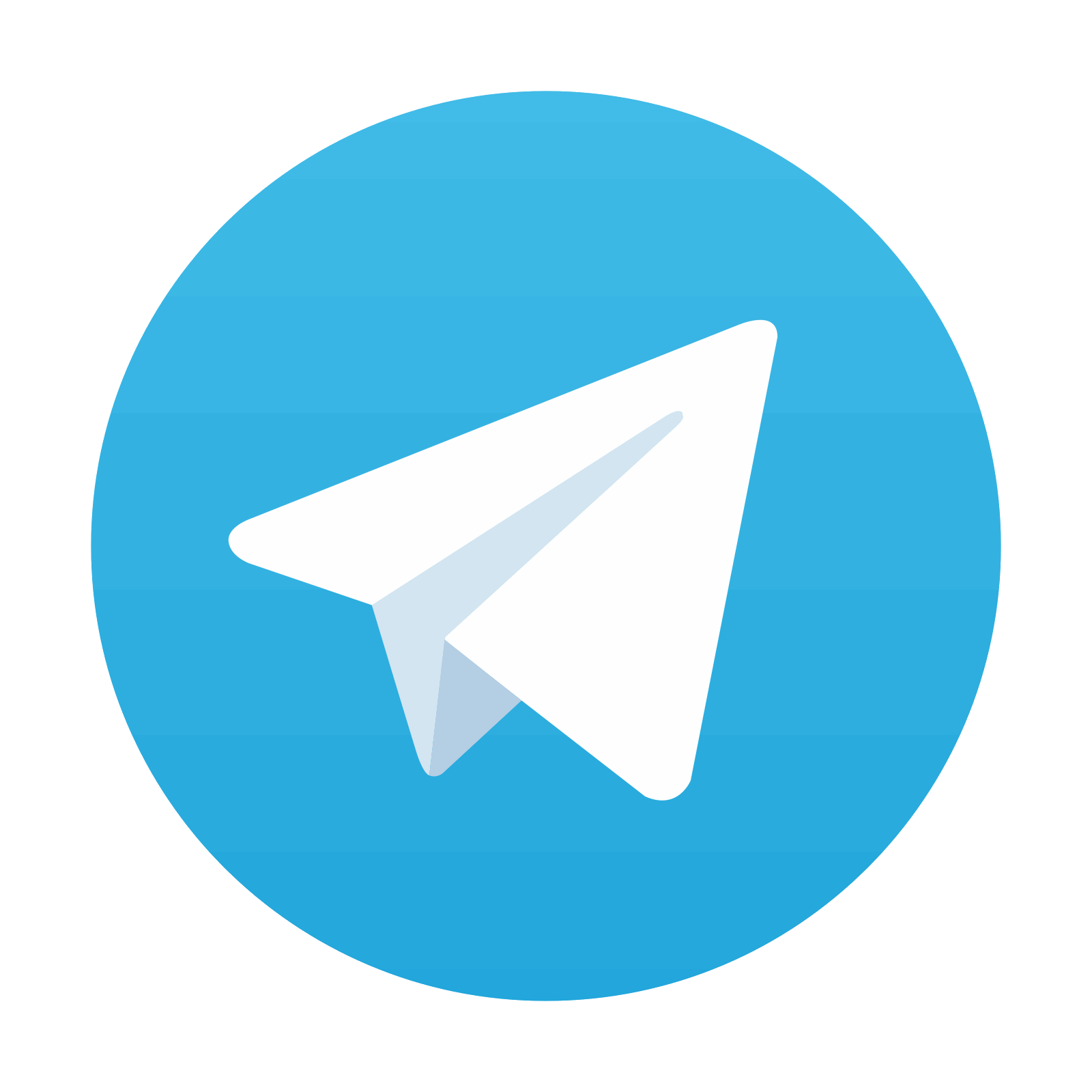
Stay updated, free dental videos. Join our Telegram channel

VIDEdental - Online dental courses
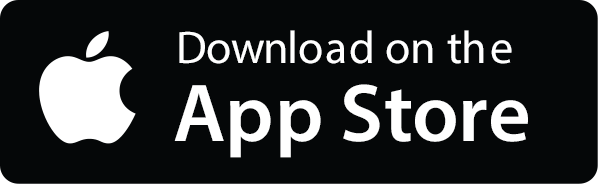
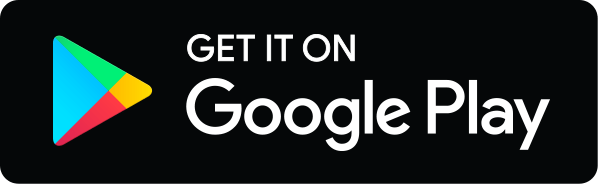