7
Age‐related Differences in the Brain–Stomatognathic Axis
7.1 Age‐related Differences in Brain Mechanisms
7.1.1 Introduction
Age‐related difference in brain function and structure has been widely investigated in recent years. Several neuroimaging findings, such as the age‐related decline in the total volume of grey matter and expansion of the volume of cerebrospinal fluid (CSF) and the age‐related difference in the intrinsic connectivity, have been consistently reported as the key brain features of older adults. However, the association between age‐related brain features and oral functions in older adults has remained unclear. In this chapter, we start with an introduction to the structural and functional features related to healthy aging. Subsequently, clinical implications of the age‐related brain mechanisms are outlined, and the methodological considerations of the research on the aging brain are discussed.
7.1.2 Age‐related Differences in Brain Structure
There exist great individual differences in the morphological features of the brain (see Chapter 2), such as the volume of cortical/subcortical regions and the thickness of cortical regions. In the following sections, we refer to an ‘age‐related difference’ as the difference in the brain features between different age groups. Such a difference can be observed from a cross‐sectional dataset by identifying the association between subjects’ age and brain features. In contrast, we refer to an ‘age‐related change’ as the difference of brain features between different ages in the same cohort of subjects. From the point of research design, the age‐related changes should be identified by comparing the brain features between different time points via longitudinal research. As shown in the following discussion, clarifying the research design of age‐related studies is pivotal for properly interpreting the results.
7.1.2.1 Cortical Morphology
Cumulating evidence has suggested that as age increases, the global morphology of grey matter showed a significant reduction in multiple aspects, including the volume, surface area and cortical thickness (Lemaitre et al. 2012; Storsve et al. 2014; Thambisetty et al. 2010). Early findings show a significant reduction in grey matter volume (GMV) (mean ± standard deviation) in the older group (50–86 years, 626.1 ± 84.3 ml) compared to the younger group (20–49 years, 717.8 ± 96.8 ml). In contrast, the difference in the total intracranial volume, which consists of grey matter, white matter and CSF volumes, was not statistically significant between older and younger groups (Ge et al. 2002). In adults between 18 and 87 years old, there is an annual reduction in the total cortical GMV at the rate of 1.89 cm3/year, in the global average thickness at the rate of 0.004 mm/year and in the total surface area at the rate of 3.68 cm2/year (Lemaitre et al. 2012). There also exists a strong association between different morphometric features. For example, an accelerating reduction in the volume was positively correlated with an accelerating reduction in the cortical thickness. In contrast, more reduction in the cortical thickness may be associated with less reduction in the surface area in occipital‐parietal regions (Storsve et al. 2014). Together, the findings suggest that changes in brain structure at the whole‐brain scale may occur as age increases. The changes in the overall architecture of the brain may be associated with some systemic factors related to aging. For example, individual differences in GMV were associated with the degree of physical activity and cardiorespiratory fitness (Erickson et al. 2014).
Notably, the reduction of morphological features at the whole‐brain scale does not mean a homogenous reduction in all brain regions. Region‐wise, some brain regions show more age‐related reduction than others (Lemaitre et al. 2012; Pfefferbaum et al. 2013; Storsve et al. 2014; Thambisetty et al. 2010). For example, based on 216 healthy volunteers without a history of neurological or psychiatric disorders, Lemaitre et al. (2012) found that the age‐related differences in the morphological features are not equal between different brain regions. The prefrontal cortex (PFC), including the superior frontal gyrus (SFG), the middle frontal gyrus (MFG) and the frontal pole, showed the greatest reduction in volume than the other regions, and the SFG also showed the greatest reduction in the cortical thickness (Lemaitre et al. 2012). Longitudinal studies also revealed age‐related atrophy in GMV and cortical thinning in various regions (Taki et al. 2013; Thambisetty et al. 2010). A longitudinal study based on 207 healthy adults aged between 23 and 87 years revealed a substantial regional difference in the annual changes in the thickness and surface area. For example, as age increases, the temporal lobe showed an accelerating reduction, while the PFC showed a decelerating reduction (Storsve et al. 2014). Consistently, a longitudinal study (over a one‐ to eight‐year interval) on adults aged from 20 to 85 years revealed different patterns of age‐related reduction in the regional brain volume. The age‐related reduction of the frontal regions and the hippocampus fits a cubic model of regression, while the reduction of the primary somatosensory area (i.e. the precentral and postcentral cortices) fits a linear model of regression (Pfefferbaum et al. 2013). These findings suggest that the age‐related effect on structural features varies between different brain regions.
7.1.2.2 White Matter Morphometrics
Consistent with the changes in the cortical grey matter, cross‐sectional research has revealed an age‐related difference in the morphometrics of white matter, including a difference in the total white matter volume (WMV) (mean ± standard deviation) between the younger group (20–49 years, 499.9 ± 73.7 ml) and the older group (50–86 years, 445.3 ± 56.0 ml) (Ge et al. 2002). Longitudinal research revealed an annual decrease in fractional anisotropy (FA) and an increase in diffusivity (Sexton et al. 2014). The changes also showed a region‐specific pattern, with the frontal and parietal regions showing a greater degree of reduction (Sexton et al. 2014). In middle‐aged adults, a significant WM contraction in frontal, temporal and cerebellar regions can be identified in a longitudinal study over four years (Ly et al. 2014). The FA of white matter regions (including the entorhinal area, genu and splenium) estimated at the baseline is associated with the rate of atrophy of other tracts, including the superior longitudinal fasciculus and anterior corona radiata (Ly et al. 2014). Notably, the changes can also be identified in very old subjects. For example, in the very old subjects aged between 81 and 103 years, a decrease in mean FA and an increase in mean diffusivity (MD) were identified for all tracts in longitudinal research with a period of 2.3 years (Lövdén et al. 2014). Notably, the changes were associated with the decreased performance of a perceptual speed task, suggesting an association between alterations of white matter and age‐related changes in sensorimotor functions (Lövdén et al. 2014).
7.1.2.3 Structural Connectivity
In addition to the regional changes in morphological features, aging is also associated with brain connectivity. Diffusion tensor imaging (DTI), a widely used method for diffusion magnetic resonance imaging (dMRI), can be used to estimate the number of potential trajectories between brain regions, indexed as the strength of structural connectivity (see Chapter 2). An age‐related difference in structural connectivity has been identified, based on 47 healthy adults aged 18–82 years (Zimmermann et al. 2016). The research further identified the association between age and the coupling between structural connectivity and intrinsic functional connectivity. A recent dMRI study based on a large sample of elderly people (73–76 years), with a follow‐up of three years, revealed a decreased FA and an increased MD in multiple tracts (Alloza et al. 2018), which is similar to the previous findings (Lövdén et al. 2014). Furthermore, the study investigated the change in the whole‐brain architecture of structural connectivity using a graph‐based method (see Section 2.4). The authors found a significant decline in the mean edge weight and strength of structural connectivity. However, changes in the global efficiency and the clustering coefficient were not statistically significant (Alloza et al. 2018). In general, both findings from cross‐sectional and longitudinal research suggest that aging may be associated with a decreased WMV and FA. Moreover, the alteration in structural connectivity may occur not only in a specific region but also in the whole‐brain architecture of structural connectivity. Additionally, the structural covariance of grey matter (see Chapter 2), such as the correlation of the cortical thickness between different regions across individuals, was used to estimate the inter‐regional association. A recent study based on 257 healthy adults aged 20–87 years reported that structural covariance of grey matter declined as age increased, signifying a ‘disintegration’ of grey matter network (Koini et al. 2018). Consistently, the findings revealed that in normal aging, the structural changes may not happen in an isolated region.
7.1.2.4 Cerebellum
In addition to the cerebral cortex, structural features of the cerebellum have also been widely investigated in recent years. Based on 210 older people (mean age = 70.7 years), the estimated size of bilateral cerebellar grey and white matter was approximately 94 and 31 ml, respectively (Koppelmans et al. 2015). Age‐related changes in brain features of the cerebellum were reported in a recent longitudinal study based on cognitively normal adults for a period of 3.1 years. At the baseline session, the average (±standard deviation) cerebellar volume adjusted for intracranial volume is approximately 123 (±10) cm3 (Tabatabaei‐Jafari et al. 2017). The study reported an annual shrinkage rate of 0.36% for the cerebellar volume (Tabatabaei‐Jafari et al. 2017). It should be noted that the cerebellum is a functionally and structurally inhomogeneous structure. Recent neuroimaging findings suggest that the anterior and posterior lobes of the cerebellum may participate in different mental functions, collaborating with different cortical regions (Stoodley and Schmahmann 2010). A longitudinal study reveals a significant positive correlation between the age and the annual rate of change in the posterior lobe of the cerebellum, but a negative correlation in the anterior lobe of the cerebellum (Taki et al. 2013). Therefore, the association between aging and brain features may vary between different subregions of the cerebellum.
7.1.2.5 Cerebrospinal Fluid
One of the most pronounced age‐related changes of the morphometric features is the increased volume of CSF, particularly in one’s late life. For example, the age group over 60 years old showed a rapid expansion of CSF volume, especially in men (Pfefferbaum et al. 2013). CSF expansion occurs in both the lateral and the third ventricle, with the lateral ventricle showing more pronounced age‐related changes (Pfefferbaum et al. 2013).
7.1.3 Age‐related Differences in Intrinsic Functional Connectivity
In the following discussion, we outline the age‐related differences associated with functional connectivity of the brain, primarily focusing on the intrinsic functional connectivity of resting‐state functional magnetic resonance imaging (fMRI).
7.1.3.1 Inter‐regional Resting‐stated Functional Connectivity
Compared to the findings of structural features, the association between aging and intrinsic functional connectivity is less clear‐cut. Alterations in the default mode network (DMN), the salience network and the central executive network were associated with aging (Sala‐Llonch et al. 2015). The DMN has been widely investigated in recent years (Geerligs et al. 2015; Huang et al. 2015; Song et al. 2014; Tomasi and Volkow 2012). In resting‐state functional magnetic resonance imaging (rs‐fMRI), increased functional connectivity of the DMN is consistently identified in subjects of all age groups compared to task‐based fMRI. The functional connectivity of the DMN may play a key in the processing of episodic memory and self‐referential information (Raichle 2015) when subjects are in a relaxed state, not disturbed by environmental stimuli. In contrast, in task‐based fMRI, the DMN shows a decreased functional connectivity compared to rs‐fMRI. The decreased in DMN connectivity is associated with increased functional connectivity in the salience and central executive networks when subjects are engaged with a task that demands more attention and cognitive resources (Raichle 2015). Notably, the DMN consists of several subnetworks that may subserve different mental functions. A study based on a larger sample (N = 430) of healthy older people (51–85 years) revealed that the ventral subnetwork of the DMN (consisting of the precuneus, the posterior cingulate cortex and the hippocampus), but not the anterior or the posterior subnetwork, showed a significant age‐related reduction (Huang et al. 2015). Notably, as age increases, the association between the DMN and other intrinsic functional connectivity networks may vary. For example, the association between the posterior DMN and the auditory networks was found negatively correlated with age. In contrast, the association between the DMN and the somatosensory network shows no significant correlation (Huang et al. 2015). In contrast, the age‐related differences in the sensorimotor network were less consistent in the literature. While some researchers did not report a significant age‐related difference in the sensorimotor network (Geerligs et al. 2015), others reported stronger connectivity in the sensorimotor network in older vs. younger subjects (Song et al. 2014) and a positive correlation between age and functional connectivity density of the network (Tomasi and Volkow 2012). Wu et al. (2007) reported that within the sensorimotor network, only the right cingulate motor area and the left premotor cortex (PMC) revealed a decreased resting‐state functional connectivity in the older group compared to the younger group. The diversity of results may be interpreted according to methodological variations in experimental design and analysis (Gargouri et al. 2018).
7.1.3.2 Whole‐brain Functional Connectome
The investigation on the whole‐brain functional connectome, which is largely based on graph‐based methods, has gradually revealed a consistent pattern of ‘decreased segregation’ of the functional connectome in older people. In a study based on 210 healthy adults, Chan et al. (2014) reported that as age increased, the connectivity within an individual functional module of the brain decreased; in contrast, the connectivity between different systems increased (Chan et al. 2014). In other words, in the older group compared to the younger group, the whole‐brain connectome showed a reduction in the degree of segregation. Moreover, the degree of decreased segregation varied between brain systems (networks): the association systems showed a pronounced decrease in segregation, particularly in the oldest age group (Chan et al. 2014). Consistently, the degree of modularity, which signifies the segregation of communities of nodes, was significantly lower in the older group compared to the younger group (Cao et al. 2014; Geerligs et al. 2015; Song et al. 2014). The decrease in modularity was associated with a decrease in local efficiency (Cao et al. 2014, Geerligs et al. 2015, Song et al. 2014), but the global efficiency of whole‐brain connectome remained similar between the age groups (Geerligs et al. 2015). Such age‐related differences in the whole‐brain functional connectome may reflect a substantial association between aging and information transmission between brain regions (Sala‐Llonch et al. 2015). On the one hand, the decreased segregation, as demonstrated by a lower degree of modularity of weaker intra‐network connectivity, may signify a loss of selectivity in recruiting neural substrates (King et al. 2018). On the other hand, the corresponding increase in inter‐network connectivity may signify the compensation of function, i.e. to maintain the function by recruiting a more extensive network (Cox et al. 2015). In sum, the findings suggest that as age increases, a global change in the functional architecture of the brain may occur. Moreover, the alterations in the overall connectome do not imply a homogenous change in all networks. Decreased connectivity is consistently found in the DMN. However, the age‐related alterations may be less clear‐cut for other networks, such as the somatosensory network (Sala‐Llonch et al. 2015).
7.1.4 Clinical Implications
Neurodegenerative disorders, including Alzheimer’s disease (AzD) and Parkinson’s disease (PD), have become the major challenge in geriatric healthcare, and aging is one of the major risk factors of the diseases (Hou et al. 2019). An earlier neuroimaging meta‐analysis based on 429 patients of amnestic mild cognitive impairment (MCI) revealed that grey matter atrophy at the left hippocampus and parahippocampus. Notably, the reduction of GMV of these regions, as a neuroimaging marker, was predictive of the conversion from amnestic MCI to AzD (Ferreira et al. 2011). A recent study found that patients with AzD, but not MCI, showed a larger rate of atrophy of the cerebellar volume compared to the cognitively normal participants. The findings suggest the cerebellar atrophy may be associated with the progression of the late phase of cognitive impairment (Tabatabaei‐Jafari et al. 2017). The neuroimaging markers derived from structural brain features also help to predict individual sensorimotor performance. For example, in older people, cerebellar WMV was found associated with hand‐tapping speed and manual dexterity (Koppelmans et al. 2015). Finally, the difference in functional features, such as intrinsic functional connectivity, also reflects clinical conditions. As noted previously, the DMN has been widely investigated for its association with aging. Patients with AzD showed decreased activity in the posterior cingulate and hippocampus during a resting state compared to healthy controls (Greicius et al. 2004). In general, the findings suggest that in older people, brain features derived from structural or functional neuroimaging may provide important clues about the progression of neurodegenerative disorders.
7.1.5 Methodological Considerations
It should be noted that methodological variations may lead to different results between studies. In terms of structural features, the variations in pre‐processing steps, including the methods of brain segmentation and normalization, may affect the estimation of morphological features (Callaert et al. 2014; Peelle et al. 2012). For example, the methods for adjusting for the global effects from the individual differences in a specific tissue class (i.e. the total GMV) may have a substantial influence on the results of brain morphometry (Peelle et al. 2012). The age‐related differences in GMV were also influenced by the methods of segmentation and normalization, and the method‐specific effect was more pronounced in the cortical area nearby major sulci and fissures (Callaert et al. 2014). In terms of intrinsic functional connectivity, the variations in the order of pre‐processing steps of functional images may affect the resulting metrics of the connectome (Gargouri et al. 2018). As shown in Chapter 2, the methodological issues, such as how to create a brain region for analysis (i.e. the definition of a network node) and how to estimate the strength between brain regions (i.e. the definition of a network edge), play a critical role about how the network is represented (see Section 2.4). In sum, the findings suggest that the methodological issues should be carefully considered when one interprets the age‐related findings of the brain.
7.1.6 Summary
- As age increased, a global reduction in the volume and cortical thickness would be expected. This global change does not correspond with a homogenous change in all regions. In contrast, the rates of regional change differ substantially between brain regions.
- The region‐wise diversity is also significant in terms of resting‐state functional connectivity, which showed consistent age‐related differences in some networks (e.g. the DMN) but less clear‐cut results in other networks (e.g. the sensorimotor network).
- Cumulating evidence has suggested that the individual differences in brain mechanisms can be used as a clinical index for predicting individual performance or disease status. However, the methodological variances behind data analysis, which may lead to substantially different results, should be considered.
7.2 Age‐related Changes in Oral Sensorimotor Functions
7.2.1 Introduction
As noted in Chapter 1, the ‘functional view’ of the stomatognathic system focuses on how the oral structure adapts to the environment and maintains normal functions. Aging has an overall effect on many aspects of physical and mental functions. Therefore, to elucidate the association between the brain and oral functions, the influence of age should not be ignored, particularly in geriatric patients. In this chapter, we provide a brief introduction to the concept of ‘healthy aging’ and its association with general physical and mental conditions. The age‐related differences in the stomatognathic system, including the structural aspects (e.g. tooth loss) and the functional aspects (e.g. pain and mastication), are outlined.
7.2.2 General Health Conditions Associated with Aging
According to the World Health Organization (WHO), aging is associated with ‘a gradual decrease in physical and mental capacity, a growing risk of disease, and ultimately, death’ (https://www.who.int/news‐room/fact‐sheets/detail/ageing‐and‐health). At the biological level, aging is the result of ‘the accumulation of a wide variety of molecular and cellular damage over time’, according to the WHO (https://www.who.int/news‐room/fact‐sheets/detail/ageing‐and‐health). Therefore, as a natural process, aging relates to both physical and mental changes of individual health conditions. In the following sections, we focus on the general association between aging and health.
7.2.2.1 General Issues of Age‐related Effect on Physical and Mental Conditions
Instead of a discussion about all the physical and mental changes related to aging, we focus on the two common findings that have been repeatedly reported in geriatric research. Firstly, the age‐related effect varies between different parts of our body. This is exemplified by the physical changes of the skeletal muscle. As age increases, muscles become ‘atrophic’ in general, such as a decreased cross‐sectional area of muscles. The morphological change may be associated with the reduction in the number of muscle fibres or/and the atrophy of the fibre per se (McComas 1998). Notably, the aging‐related effects on muscle tissues differ between the jaw and the limb muscles. As age increases, the masseter showed a decreased proportion of the type I fibres and an increased proportion of the type II fibres, while the biceps showed no changes in the type I fibre but a decreased proportion of the type IIB fibres (Monemi et al. 1998). In jaw muscles, the nerve terminals in muscle fibres maintain a higher capacity of regeneration compared to the limb muscles (Avivi‐Arber and Sessle 2018). Clinically, jaw muscles are less susceptible to certain neuromuscular diseases and age‐related atrophy compared to limb muscles (Avivi‐Arber and Sessle 2018). The diversity of age‐related effects between the jaw and the limb muscles may underlie the different clinical symptoms and signs between the regions.
The second common finding from the literature is about the time course of the age‐related effect. It is widely accepted that as age increases, the capacity of mental functions may decline to some degree. However, the time course of the decline may vary between different mental functions. The capacity of some mental functions may show a life‐long decline, i.e. a decline throughout one’s lifespan. In other mental functions, a decline of capacity may occur in one’s late life (Hedden and Gabrieli 2004). The capacity for information processing, such as the speed of perceptual processing and working memory, presents a trend of life‐long decline. In contrast, the capacity of semantic memory presents a trend of late‐life decline. Finally, some capacity is relatively stable throughout one’s lifespan, such as autobiographical memory (i.e. the memory of personal history and self‐relevant information) (Hedden and Gabrieli 2004). In general, the capacity of mental functions may decline as age increases, but the time course of the declination may vary between different mental functions.
7.2.2.2 Relationship Between Aging and Diseases
Aging is the major risk factor for many disorders, including cardiovascular and neurodegenerative diseases (McHugh and Gil 2018). Traditionally, people tend to link aging with ‘losses’ in physical or mental capabilities. This belief is further reinforced by research findings from a comparison between different age groups. The comparison usually reveals a decreased performance in physical or mental tasks in older subjects compared to younger subjects (Rowe and Kahn 1987). However, the results usually ignore the heterogeneity within an age group. Even though an older group shows an averagely worse performance compared to a younger group, some older individuals may perform better than others. The variability of performance within older people can be interpreted by multiple factors, e.g. individual habits or psychosocial factors, which are extrinsic variables that modify the effect of aging (Rowe and Kahn 1987). In successful aging, these extrinsic factors may play a neutral or positive role that renders older people with little or no loss of physiological functions (Rowe and Kahn 1987). Based on the nation‐representative longitudinal data of Taiwan, researchers categorized the older cohorts into four types, successful aging, usual aging, health declining and care demanding, according to six indicators: chronic diseases, physical function difficulties, depressive symptoms, social support, social participation and economic satisfaction (Hsu and Jones 2012). Based on the selected indicators, the cohort with successful aging showed fewer problems in chronic diseases, physical function difficulties and depressive symptoms compared to the health‐declining and care‐demanding cohorts (Hsu and Jones 2012). Notably, different cohorts showed different trajectories of aging (i.e. the progression of the age‐related variable over time), supporting the validity of assessing aging using multiple indicators.
7.2.3 The Age‐related Differences in the Stomatognathic System
In the following section, we focus on the sensorimotor aspects regarding feeding behaviour, including teeth and dentition, orofacial muscles and salivary secretion. We also focus on the differences between different age groups based on cross‐sectional studies.
7.2.3.1 Tooth Loss
Tooth loss is commonly found in older people. Meta‐analytic findings reveal that the prevalence of severe tooth loss (i.e. having fewer than nine remaining permanent teeth) increased gradually with age (Kassebaum et al. 2014b). However, it should be noted that the loss of natural teeth cannot be fully attributed to aging since tooth loss is markedly associated with dental diseases, including periodontitis and severe dental caries, and the prevalence of periodontitis and dental caries is high among older people (Lopez et al. 2017). As age increases, the multirooted teeth are at a greater risk of periodontitis and tooth loss (Lamster et al. 2016). Interestingly, the incidence of severe periodontitis peaks sharply during the younger stage (20–40 years old), not in the elderly stage of one’s lifespan (Kassebaum et al. 2014a). In addition to oral diseases, fear and anxiety may mediate the association between aging and tooth loss (Friedman and Lamster 2016; Kossioni and Dontas 2007). According to a nation‐wide survey based on Finnish adults, a strong fear of dental visiting is prevalent in all age groups; even in the elderly group (more than 75 years old), there was a prevalence of 2.2% of being very much afraid of visiting dentists (Liinavuori et al. 2016). In general, both pathological and psychosocial factors may potentiate the rate of tooth loss as age increases.
7.2.3.2 Orofacial Muscles
As noted in the preceding section, the age‐related effect of skeletal muscles may vary between the jaw and limb muscles. The jaw muscles may be innervated by more motor units, compared to the limb muscles (McComas 1998). At the histochemical level, the jaw muscles are composed of type I fibres as well as type II fibres. Therefore, the jaw muscles have a longer contraction time, and they are less susceptible to fatigue (McComas 1998). Therefore, these muscles can perform the chewing movement for a long period. While animal research focuses on histological configurations of orofacial muscles, imaging methods make it feasible to assess the quality of jaw muscles. Recent studies using ultrasound sonography revealed that the echo intensity of masticatory muscles was not only associated with the displacement of the muscles (Hara et al. 2020) but also systemic factors of aging, including grip force and walking speed (Yamaguchi et al. 2019). Maximal tongue pressure (MTP), another critical physiological factor associated with mastication and swallowing, also declines as age increases (Liu et al. 2019; Ohno et al. 2020). Notably, in older subjects, a higher MTP was not associated with better echo intensity, an indicator of muscular quality, of the tongue muscle (Chantaramanee et al. 2019). In contrast, oral diadochokinesis was associated with the quality of the tongue muscle (Chantaramanee et al. 2019). The findings suggest a complex relationship between strength, structure and skill‐related tongue movement.
7.2.3.3 Salivary Secretion
Both hyposalivation (i.e. the reduction of salivary flow rate, SFR) and xerostomia (i.e. subjective perception of dry mouth) are commonly seen in older dental patients (Kossioni and Dontas 2007; Lamster et al. 2016). However, the association between aging and salivary secretion may be a more complicated issue because reduced saliva secretion, which can be quantified by SFR, is associated with both intrinsic factors (e.g. changes in salivary glands) and extrinsic factors (e.g. polypharmacy) (Villa et al. 2015). An early longitudinal study over three years on subjects with a broad age range (26–90 years) revealed no significant difference in stimulated or unstimulated SFR of major glands over a three‐year period (Ship et al. 1995). Findings from cross‐sectional research revealed a significantly lower unstimulated SFR in older groups (age > 40 years) compared to the youngest group (age ≰ 39 years). In contrast, the stimulated SFR of parotid saliva was not significantly different between the age groups (Percival et al. 1994). In another cross‐sectional study with a larger sample size (N = 540) of healthy adults, the researchers found that the older group (≱70 years) showed a significantly lower stimulated SFR compared to the other age groups (Smith et al. 2013). Meta‐analytic findings showed that of the stimulated and unstimulated SFR of the submandibular/sublingual salivary glands were significantly lower in older adults compared to younger adults (Affoo et al. 2015). The findings generally reveal a reduction in SFR in elderly people. However, the association between the reduction and the intrinsic/extrinsic factors requires more investigation.
7.2.4 Age‐related Changes in Oral Sensory Functions
In the preceding sections, we focused on the age‐related effect on the physiological foundations of the stomatognathic system, including jaw muscles and saliva secretion. In recent years, there has been an increased number of studies focusing on the age‐related effect on oral functions. As summarized in Table 7.1, in the following sections, we focus on the association between aging and changes in the threshold or the intensity of oral sensorimotor functions.
7.2.4.1 Somatosensory Functions
Earlier studies revealed a mixed result of the association between aging and oral somatosensation. For example, by investigating 60 healthy adults of a broad age range (23–96 years), Calhoun et al. (1992) found no significant age‐related differences in thermal sensitivity, somesthetic sensitivity, proprioception, vibration detection (on the soft palate) and two‐point discrimination (on the tongue and palate). In contrast, a declined ability of tactile, vibratory and two‐point discrimination was noted on the lip (Calhoun et al. 1992). In general, oral sensation remained good as age increases, with a slight decline after the age of 80 years (Calhoun et al. 1992). Another study based on 178 healthy adults aged 20–89 years revealed an elevation in sensory thresholds, including warming and cooling temperatures, pain, touch, two‐point discrimination and taste, for the older subjects (≱65 years) compared to the younger subjects (<45 years) (Heft and Robinson 2010). A main effect of sex was noted, but an interaction between sex and age was insignificant, except for the sensation of sour (i.e. older men having the highest sour threshold). Such an age‐related difference was also found for lingual tactile thresholds, which were higher in subjects aged >40 years (Bangcuyo and Simons 2017). In general, the findings suggest that changes in oral somatosensory functions, including elevation of thresholds and declined abilities of discrimination, may occur selectively in some regions (e.g. the lip and the chin). The diversity of results may be attributed to the variations of testing methodology (Heft and Robinson 2017). Furthermore, recent studies have presented a potential link between oral somatosensory functions and hearing threshold (Etter et al. 2016) as well as systemic factors (Silva et al. 2017), though the conclusions are limited by a smaller sample size. Notably, in older adults, there was no significant difference in the pain detection threshold or the tactile detection threshold between patients with burning mouth syndrome (BMS) and healthy controls (Honda et al. 2019). The findings reveal great individual variations in oral somatosensory functions in older adults.
Table 7.1 Recent findings on age‐related changes in oral sensorimotor functions (since 2015).
Source | Participants (region) | Methods (assessments) |
---|---|---|
Masticatory and swallowing functions | ||
Ohno et al. (2020) | 152 adults aged 20–79 years; Japan | GF, MBF, MTP, MP and swallowing threshold |
Hara et al. (2020) | 74 healthy adults (>65 years); Japan | Masseter thickness, masseter echo intensity, displacement of the masseter while biting forcefully and MBF |
Liu et al. (2019) | 100 healthy adults aged 22–89 years; China | MBF, MTP, maximum oral volume, unstimulated and stimulated saliva and chewing efficiency |
Chantaramanee et al. (2019) | 94 healthy adults (mean age = 71.1 years); Japan | Tongue thickness, MTP, echo intensity of the tongue and oral diadochokinesis |
Tactile functions and pain | ||
Honda et al. (2019) | 20 patients with BMS (mean age = 68.1 years) (20 healthy controls); Japan | Tactile detection thresholds and filament‐prick pain detection thresholds |
Silva et al. (2017) | 30 adults (mean age = 70.4 years); Brazil | Periodontal conditions, sensory testing (gustatory, olfactory, thermal, mechanical and pain thresholds) |
Bangcuyo and Simons (2017) | 48 adults; USA | lingual threshold sensitivity, suprathreshold sensitivity and taste bud density |
Etter et al. (2016) | 60 adults aged 19–84 years; USA | Pure‐tone hearing thresholds and labial vibrotactile detection thresholds |
De Rui et al. (2015) | 97 healthy adults of four age groups (65–69, 70–74, 75–79 and ≥80 years); Italy | The pressure pain threshold of the temporal muscle, the masseter and the cervical muscles. |
Gustatory functions | ||
Pushpass et al. (2019) | 31 younger (mean age = 24 years) and 25 older (mean age = 72 years) adults; UK | Taste, olfactory and chemical (capsaicin) stimuli |
Ogawa et al. (2017b) | 621 subjects aged 79–81 years; Japan |
Taste sensitivity (at baseline and 3 years later) |
Yoshinaka et al. (2016) | 996 young–old adults aged 69–71 years and 949 old–old adults aged 79–81 years; Japan | Recognition thresholds for the four basic (sweet, sour, salty and bitter) tastes |
Notes: BMS: burning mouth syndrome; GF: grip force; MBF: maximum biting force; MP: masticatory performance; MTP: maximum tongue pressure.
7.2.4.2 Pain
The study by Heft and Robinson revealed a higher threshold of thermal pain for older adults compared to younger adults, at the lip and chin sites (47.1 °C vs. 43.7 °C for older vs. younger male at the chin and 46.3 °C vs. 44.1 °C for older vs. younger female at the chin) (Heft and Robinson 2010). However, in a recent meta‐analysis of pain sensitivity on the hand and arm area in pain‐free healthy adults, the researchers did not find a significant age‐related difference in heat pain threshold (El Tumi et al. 2017). The meta‐analytic findings showed a lower pressure pain threshold in older adults compared to younger adults (El Tumi et al. 2017). In contrast, there was no significant difference in pressure pain threshold on masticatory and cervical muscles between different age groups in elderly people (De Rui et al. 2015). In addition to pain sensitivity, older subjects may show an increased tolerance of pain. For example, in male (but not female) subjects, their pain tolerance of receiving electrical stimuli on the alveolar ridge is positively correlated with age (Nakashima et al. 2015). In general, the findings suggest that age is associated with alterations in the sensory processing of pain.
7.2.4.3 Gustation
Aging is a predominant factor associated with changing gustation (Ogawa et al. 2017a). An earlier investigation on 3603 community‐dwelling adults (aged ≱40 years) revealed that the prevalence of smell and taste problems increased as age increased. The prevalence of smell and taste problems was the highest in subjects aged >80 years (i.e. 32% for smell problems and 27% for taste problems) (Rawal et al. 2016). Findings from cross‐sectional research revealed that the older subjects showed an increased threshold to both sour and salt compared to the younger subject (Heft and Robinson 2010). Older subjects also showed a lower perception of umami taste and menthol odour compared to the younger subjects. However, the chemosensation of the stimulants of transient receptor potential (TRP) channels was not significantly different between the older and younger subjects (Pushpass et al. 2019). The age‐related change in gustation may result from structural alteration of taste organs, including a reduced density of taste buds and a reduced number of taste cells in a taste bud (Feng et al. 2014). Notably, the association between aging and declined gustatory functions may be mediated by other factors (Ogawa et al. 2017a). Firstly, both sex and age are associated with individual differences in taste threshold in older adults. For example, findings from cross‐sectional research revealed that an age‐related increase in sour threshold was more pronounced in male subjects compared to female subjects (Heft and Robinson 2010). Findings from longitudinal research revealed that in elderly people, male subjects showed declined sensitivity in sweet and sour compared to female subjects (Ogawa et al. 2017b). A recent study reported that old–old (aged 79–81 years) subjects showed a higher threshold of recognizing four basic tastes compared to the young–old (aged 69–71 years) subjects. Moreover, among the young–old and old–old groups, female subjects showed a lower recognition threshold compared to male subjects in sweet, salty and bitter tastes (Yoshinaka et al. 2016). Secondly, declined gustatory functions are associated with medication and systematic diseases, especially metabolic and endocrine diseases, which are both common in older people (Ogawa et al. 2017a). Thirdly, cumulating evidence suggests that a greater decline in gustatory functions may be associated with poorer cognitive abilities. For example, a longitudinal study over three years reveals that subjects with a lower cognitive score showed a greater decline of salt taste (Ogawa et al. 2017b). Together, the findings suggest that the age‐related decline in gustation may be associated with systemic factors other than the changes in taste organs.
7.2.5 Age‐related Changes in Masticatory Functions
There exists a substantial association between aging and declined masticatory functions (e.g. more tooth loss and worse chewing ability). However, the association between aging, mastication and the structural and functional features of the stomatognathic system, such as tooth loss and salivary secretion, may be more complicated. The association between these factors is outlined in the following sections.
7.2.5.1 The Role of Dentition
Cumulative evidence has revealed that masticatory performance (MP) (see Section 3.1) decreased as age increased (Lin et al. 2017a; Kosaka et al. 2016; Ohno et al. 2020). However, the time course of the age‐related decline is more similar to a late‐life change rather than a life‐long change. In other words, MP was more stable during the young and middle ages and became gradually declined during an older age (Lin et al. 2017a). The association between aging and a lower MP may not be very pronounced for the young–old adults (aged 60–65 years), but become stronger in very‐old adults (aged 80–85 years) (Peyron et al. 2017). Such a relationship may be associated with multiple factors. Firstly, MP is closely associated with the number of tooth loss (Hatch et al. 2001; Ikebe et al. 2012; Kosaka et al. 2016), especially the posterior teeth (Ikebe et al. 2012). In an earlier study based on 631 subjects aged 37–80 years, Hatch et al. (2001) found that the number of functional tooth units and maximal bite force (MBF), rather than age per se, was the key determinant of MP. Therefore, an increased number of tooth loss, rather than aging, was regarded as the main factor of a lower MP in older individuals (Kossioni and Dontas 2007). Though the association between tooth loss and a lower MP has been widely reported, the number of tooth loss cannot fully predict individual variability in MP. In a large sample cross‐sectional study based on Japanese elderly people, Ikebe et al. (2012) investigated the association between MP (indexed by a cutting task) and the pattern of tooth loss, which was indexed by Eichner index. The dentition with Eichner index A represents a full occluding contact of bilateral molars and premolars. In contrast, the dentition with Eichner index C represents no occluding contact between anterior or posterior teeth. The average MP from subjects with Eichner index C was 1316 mm2, which was only half of the average MP from subjects with Eichner index A (2659 mm2). Notably, the standard deviation of MP in the subjects with Eichner index C was 894 mm2, which was as large as that of the subjects with Eichner index A (648 mm2) (Ikebe et al. 2012). The findings reveal that in the older individuals without any occluding contact, some of them would have substantial difficulty in chewing, but some still maintain an adequate masticatory function. Therefore, though tooth loss plays a major role in a declined masticatory function, other factors (see below) may contribute to mastication, for a compensatory role, to maintain masticatory function in older people.
7.2.5.2 The Roles of Masticatory Muscles, Sensory Feedback and Saliva Secretion
In addition to tooth loss, masticatory muscles also demonstrate several age‐related alterations. Decreased MP was associated with a lower MBF (Hatch et al. 2001; Kosaka et al. 2016), and in older subjects, the reduced strength of skeletal muscle was associated with reduced lean muscle mass (Goodpaster et al. 2006). The research using ultrasonography has reported that as age increased, the masseter muscle thickness decreased (Hara et al. 2020). Recently, Lin et al. (2017a) quantified the total volume of the masseter using structural MRI. They found an age‐related difference in the masseter muscle volume (MMV) between older (median volume = 21.8 mm3) and younger (27.0 mm3) groups. Across both age groups, a larger MMV was associated with a better MP. However, the association between the MMV and the MP was not statistically significant within the older group (Lin et al. 2017a). Notably, in the study, the MP was quantified by assessing the oral mixing ability (see Section 3.1) but not the cutting ability. It is possible that other factors, such as individual variability in oral sensory functions, may contribute to the association between the MMV and the MP in older subjects. One of the key factors for sensory feedback during mastication is the mechanoreceptors in the periodontal ligament (Trulsson 2006). Because periodontal diseases are common in older people, the detrimental effect on altered mechanoperception from periodontal diseases should be noted in older people. Clinical research revealed that patients with reduced periodontal support in their anterior teeth would apply a greater force to hold and split a peanut held teeth (Johansson et al. 2006). An increased alveolar bone‐height‐to‐tooth‐length (AB/T) ratio is widely adopted as an index of periodontal health. The subjects with a lower AB/T ratio (<50%), which may indicate moderate‐to‐severe generalized periodontitis, showed a lower MP compared to those with a higher AB/T ratio (De Freitas Borges et al. 2013). A large‐scale survey of 1875 Japanese elderly adults also revealed that periodontitis is a risk factor of a lower MP (Kosaka et al. 2016). Our masticatory function is also associated with salivation. The association between aging and salivation has remained unclear. Though hyposalivation and xerostomia are common in older people, these symptoms are largely associated with polypharmacy. Notably, saliva secretion of the parotid gland, as the major salivary gland, is stimulated by mastication. Consistently, an increased MP was associated with an increased stimulated SFR (when subjects were chewing a gum) rather than unstimulated SFR (when subjects were resting) (Lin et al. 2017a). Older people may increase the time for chewing to facilitate salivary output, which in turn facilitates the formation of a bolus to swallow (Peyron et al. 2017). In general, the findings suggest that age‐related differences in MP may be attributed to the alterations from multiple aspects, including dentition, masticatory muscles, the sensory feedback of periodontal tissues and salivation.
7.2.5.3 The Role of Motor Control and Behavioural Adaptation
During mastication, the tongue plays a key role in mixing and transporting food bolus in the oral cavity. In a recent study, Sagawa et al. (2019) investigated the cutting ability of 245 healthy older adults with 28 natural teeth. By controlling the number of existing teeth, they found that lower MP was associated with a decreased speed of tongue movement as well as tongue muscle force (Sagawa et al. 2019). The findings suggest that in addition to tooth loss, a declined motor skill of the tongue may contribute to a lower MP. In addition, Ohno et al. (2020) investigated 152 adults ranging from 20 to 79 years old. They found that both decreased MP (i.e. the cutting ability) and the threshold of swallowing (i.e. the chewing cycles before swallowing a jelly) were associated with aging (Ohno et al. 2020). Moreover, decreased MP was also associated with decreased tongue pressure and decreased grip force, consistent with previous findings (Ohno et al. 2020; Sagawa et al. 2019).
The cause–effect relationship between the change of motor skill and masticatory function is complicated. It is reasonable to conceive that those who have a better ability of motor control (on their tongue, for example) will show a better MP. However, it is also possible that they develop a better skill of tongue movement to compensate for the declined masticatory function, which may derive from tooth loss. In other words, older people may develop some strategies for adapting their oral conditions to maintain masticatory functions (see Chapter 8 for a detailed discussion). For example, due to a declined MP, older people may fail to reduce food into smaller pieces before swallowing it. However, as shown in Section, for a specific type of food, there exists small inter‐individual variability of the particle sizes that are ready to swallow (Prinz and Lucas 1995; Woda et al. 2006). For older people, to achieve a suitable size of bolus to swallow, they would increase the number of masticatory cycles before swallowing it (Peyron et al. 2017). Such a behavioural adaptation may be related to both age and food factors. For example, the number of pre‐swallow cycles of chewing soft elastic food is positively correlated with age (Peyron et al. 2017; Woda et al. 2006). In older people with a complete denture (CD), the number of masticatory cycles increased as the hardness of food increased (Veyrune et al. 2007). Notably, the pre‐swallow number of masticatory cycles should not be confused with masticatory frequency, i.e. ‘number of cycles per second with the sequence’ (of mastication) (Peyron et al. 2017). Masticatory frequency generally keeps constant as age increases (Lin et al. 2019; Peyron et al. 2004). Therefore, older people may adapt to tooth loss by increasing the number of masticatory cycles for processing food (Peyron et al. 2017; Woda et al. 2006).
7.2.6 Swallowing Functions
Tongue movement plays a key role in swallowing as well as in mastication. In general, the duration of swallowing may prolong in older people, partly because of the slowdown of the major movement (e.g. tongue thrust) during swallowing (Peyron et al. 2017). A recent study of 197 older subjects revealed that decreased tongue pressure was associated with increased age (in male subjects) and the occurrence of sarcopenia (in both female and male subjects). In contrast, the jaw‐opening force was only associated with the occurrence of sarcopenia in male subjects (Machida et al. 2017). In healthy older subjects, the tongue pressure was associated with the tongue muscle thickness (Yoshimi et al. 2018). The findings echoed the results from animal research, which revealed that aging was associated with a decreased number and size of muscle fibre in the tongue muscles, which may contribute to the reduction in the tongue pressure (Cullins and Connor 2017). Together, the findings highlight that physiological features of the tongue play a key role in swallowing performance.
As noted in Section, the repeated saliva swallowing test (RSST) has been adopted as a non‐invasive assessment of swallowing performance. A significant difference in the RSST score was identified between younger and older groups (Lin et al. 2019; Oguchi et al. 2000a, b). Because older people also show a lower SFR compared to younger people, the age‐related difference in swallowing performance may be attributed to an age‐related difference in SFR. The hypothesis was, however, not supported by research evidence. In their original research of the RSST, Oguchi et al. (2000b) did not find a significant difference in the RSST scores between the condition of dry swallow and the condition when artificial saliva was supplied in both younger and older subjects. In older subjects, the RSST score was not significantly correlated with either stimulated or unstimulated SFR (Lin et al. 2019). Consistently, multiple regression analyses revealed that both self‐assessed xerostomia and objective saliva secretion were not associated with the RSST score when gender, age and the number of prescribed medicines were controlled (Persson et al. 2019). How the performance of the swallowing test relates to individul differences in the stomatognathic system would require further research.
7.2.7 Summary
- The age‐related effects vary between different parts of our body. The capacity of mental functions may decline as age increases, but the time course of the declination may vary between different mental functions.
- Aging does not necessarily mean a disease condition of losses in physical or mental conditions. The practical goal of healthcare for geriatric people is to promote healthy aging, i.e. to help older people to maintain functional ability and well‐being, according to the WHO.
- Both pathological factors (e.g. increased rates of caries and periodontitis) and psychosocial factors (e.g. dental fear and anxiety) may potentiate the rate of tooth loss as age increases.
- Changes in somatosensory functions, including elevation of thresholds and declined abilities of discrimination, may occur selectively in some regions (e.g. the lip and the chin). The diversity of results may reflect the variations of testing methodology.
- Age‐related differences in MP may be attributed to the alterations from multiple aspects, including dentition, masticatory muscles and periodontal conditions.
- In older people, behavioural modification, such as increasing duration of mastication, may play a key role in adaptively maintaining masticatory function.
7.3 Association Between the Brain and Oral Functions in Older People
7.3.1 Introduction
As shown in the preceding chapters, aging is associated with a substantial structural and functional change in both the stomatognathic system and the brain. Notably, such an age‐related effect does not necessarily mean a disease condition – the age‐related changes may occur under healthy aging when individuals are free of age‐related diseases (e.g. neurodegenerative disorders). In the following sections, we review more evidence between the brain and oral functions in elderly people, focusing on mastication and swallowing. We highlight the brain–stomatognathic association primarily for people with healthy aging, while more issues of patients with age‐related diseases are discussed in the next chapter.
7.3.2 Association Between Mastication and the Brain in Older People
As discussed in the preceding sections, the age‐related decline of masticatory function can be attributed to peripheral factors, such as an increasing number of missing teeth and worse periodontal status. However, the central nervous system also plays a key role in the age‐related effect. For example, jaw movement is associated with mechanisms of rhythmic movement and reflexes, which are dominated by the brainstem (Lund 1991). The brain’s role in the link between aging and mastication is often overlooked by dentists. The next section outlines the neuroimaging findings of age‐related brain mechanisms of mastication.
7.3.2.1 Aging and the Brain Mechanisms of Mastication
With the advent of neuroimaging methods, researchers have started to investigate the role of aging in the brain mechanisms of mastication. In a pioneering study of this topic, Onozuka et al. (2003) compared the brain activation during gum chewing between younger, middle‐aged and older (aged 65–73 years) healthy subjects. The study provides three critical findings that have been identified in the following studies. Firstly, mastication was associated with the activation of several brain regions, including the primary motor cortex (M1) and the primary somatosensory cortex (S1). The pattern of activation was consistently identified in all age groups. In other words, the cortical processing of sensory and motor information of mastication is highly associated with mastication, as identified in a recent meta‐analysis (Lin 2018a). The activation of sensorimotor regions may represent a core mechanism of mastication, which is common to all age groups. Secondly, mastication was associated with the activation of more widely distributed brain regions, not limited to the S1/M1. Such an extensive activation was identified in the PMC/supplementary motor area (SMA), the PFC, the cerebellum, the thalamus and the insula (Onozuka et al. 2003). Consistently, a recent fMRI study revealed that mastication was associated with only the S1/M1 in younger dentulous subjects, but extensive regions (including in the PMC/SMA, the PFC, the cerebellum, the thalamus and the insula) in older dentulous subjects (aged 80–83 years) (Kobayashi et al. 2020). The findings suggest that older individuals compared to younger individuals may engage with a more complicated pattern of information processing during mastication. Thirdly, Onozuka et al. (2003) found that during mastication older subjects showed lower activation in the S1/M1 compared to younger subjects. Notably, they showed higher activation predominantly in the PFC compared to younger subjects (Onozuka et al. 2003). In another fMRI study, Fang et al. (2005) compared the brain activation between different age groups for hand and oral movement (including jaw opening/closing and chewing). They also found increased activation of the PFC during the chewing task. Notably, such a PFC activation was not found for hand movement (Fang et al. 2005). The PFC activation may highlight the role of cognitive processing in mastication, such as adaptation to a denture (Kamiya et al. 2016; Kimoto et al. 2011; Narita et al. 2019). In general, the studies consistently revealed both qualitative and quantitative differences in the brain mechanisms of mastication between younger and older adults. From the qualitative aspect, mastication was engaged with activation in more extensive brain regions in older adults. From the quantitative aspect, older adults showed both lower and higher activation in multiple brain regions compared to younger adults.
7.3.2.2 Recent Neuroimaging Findings of Aging and Mastication
Table 7.2 summarizes recent neuroimaging findings of the brain mechanisms associated with mastication in older adults. Several studies have been published for the association between structural brain features and the individual variability in masticatory function. Based on a random sample of 394 dementia‐free older adults (aged ≱60 years) from Sweden, Dintica et al. (2018) found a smaller total brain volume and whole‐brain GMV in subjects with complete or partial tooth loss compared to the group without tooth loss (adjusted for total intracranial volume and age). Kobayashi et al. (2018) found a smaller GMV in multiple brain regions, including the hippocampus, caudate nucleus and temporal pole, in edentulous subjects compared to dentulous older subjects. In cognitively healthy older adults, their MP (i.e. the mixing ability) was positively correlated with the regional GMV of the M1, the PMC and the dorsolateral PFC (Lin et al. 2015). In addition to grey matter, alterations in white matter may be associated with mastication. In patients with stroke, the amount of white matter hyperintense (WMH) lesions is associated with not only swallowing difficulty but also inadequate mastication (Moon et al. 2017). The association between the presence of moderate‐to‐severe WMH and edentulism was found, though both factors covaried with age (Del Brutto et al. 2016). In addition to the findings of structural features, findings from rs‐fMRI revealed that age may play a key role in the pattern of intrinsic functional connectivity related to mastication. For example, in cognitively healthy older adults, an increased MP was associated with a stronger connection between the cerebellum and the PMC/dorsolateral PFC (Lin et al. 2015). Comparing to younger subjects, older subjects showed a distinct functional network associated with mastication. In older subjects, an increased MP is further associated with an increased connection between the sensorimotor regions and the anterior insula, the cingulate cortex and the parietal lobe (Lin et al. 2017b). The findings echo the previous findings from task‐based fMRI studies (Kobayashi et al. 2020; Onozuka et al. 2003), revealing a more extensive area associated with mastication in older adults.
Several studies have focused on the association between oral rehabilitation (i.e. wearing a denture) and changes in brain activity. Earlier studies reported a reduced activation of the PFC in the condition of wearing an implant‐supported denture (IOD) compared to the condition of wearing a CD (Kimoto et al. 2011). The results suggest that when wearing an IOD (which provides better retention and stability of a denture), less cognitive effort was deployed for them to chew. Using fMRI, Kobayashi et al. (2020) found distinct functional features between older edentulous subjects with and without wearing a denture. When tapping their teeth, both younger and older dentulous subjects showed activation in the M1 and the S1 (Kobayashi et al. 2020), consistent with meta‐analytic findings (Lin 2018a). Moreover, when wearing a denture, the edentulous older subjects showed decreased activation in the S1/M1 area compared to the dentulous older subjects, which may reflect reduced sensory feedback from the periodontium (Kobayashi et al. 2020). Using functional near‐infrared spectroscopy (fNIRS), Kamiya et al. (2016) found that prefrontal activity increased when edentulous subjects were chewing by wearing a denture. Notably, during the chewing task, the level of prefrontal activity of older subjects wearing a denture was not much different from the activity of younger subjects (Kamiya et al. 2016). More recently, Narita et al. (2019) found that the prefrontal activity of chewing by wearing a denture was further associated with an increased peak amplitude of activities of the masseter and temporalis and decreased occlusal force. Together, the findings suggest that the experience of wearing a denture may be associated with prefrontal functions, which may reflect an adaptation of oral condition for improving mastication. It should be noted that, however, the PFC has diverse functions in cognitive processing. Further research is required to clarify the association between these functions and the adaptive process of oral conditions (Lin 2019).
Table 7.2 Neuroimaging research on brain mechanisms of aging and oral functions (since 2015).
Source | Participants | Methods |
---|---|---|
Mastication, with a focus on clinical factors | ||
Egashira et al. (2021) | 15 patients of cognitive impairment | sMRI/VBM |
Lin et al. (2020b) | 31 patients of cognitive impairment (31 healthy controls) | sMRI/VBM |
Lin et al. (2020a) | 40 patients of cognitive impairment (30 healthy controls) | sMRI/VBM |
Nagashima et al. (2020) | 35 healthy adults (mean age = 56.8 years) | fNIRS/mastication and foot stepping |
Dintica et al. (2018) | 394 dementia‐free older adults (≥60 years old) | sMRI/FreeSurfer |
Lin et al. (2017b) | 26 older (mean age = 64.4 years) and 26 younger healthy adults | rs‐fMRI/graph‐based network analysis |
Moon et al. (2017) | 63 patients with mild stroke (aged > 65 years) | sMRI/detection of white matter lesions |
Lin et al. (2015) | 25 older adults (mean age = 64.2 years) | sMRI, rs‐fMRI/VBM and functional connectivity |
Mastication with a focus on edentulism | ||
Kobayashi et al. (2020) | Adult dentulous (mean age = 30.7 years), older dentulous (mean age = 81.8 years) and older edentulous subjects (mean age = 79.1) wearing or not wearing dentures | fMRI/teeth tapping |
Narita et al. (2019) | 16 partially edentulous patients (mean age = 64.0 years) | fNIRS and EMG/chewing w/wo wearing a denture |
Kobayashi et al. (2018) | 13 edentulous (mean age = 81.8 years) and 11 dentulous (mean age = 77.1 years) patients | sMRI/VBM |
Kamiya et al. (2016) | 12 elderly edentulous subjects (mean age = 63.1 years) (12 young healthy controls) | fNIRS and EMG/chewing w/wo wearing a denture |
Swallowing/dysphagia | ||
Tomsen et al. (2020) | 58 patients with OD (mean age = 79.8 years) | EEG/swallowing boluses of TRPA1 and TRPM8 agonists |
Tomsen et al. (2019) | 28 patients with OD (mean age = 81.2 years) | EEG/oral capsaicin treatment |
Park et al. (2019) | 8 patients with dysphagia (mean age = 77.8 years) | PET before and after rTMS at the pharyngeal motor hot spot/water swallowing |
Lin et al. (2019) | 40 healthy older adults (mean age = 69.1 years) | sMRI/VBM, FreeSurfer |
Park et al. (2017) | 10 healthy older adults (mean age = 69.6 years) | PET and rTMS at the the pharyngeal motor hot spot/water swallowing |
Rofes et al. (2017) | 8 younger adults, 8 older adults without OD (mean age = 71.2 years) and 14 patients with OD (mean age = 79.6 years) | EEG/pharyngeal electrical stimulation |
Windel et al. (2015) | 27 old healthy adults (mean age = 64.8 years) and 24 young healthy adults | fMRI and SCR/water swallowing |
Notes: EEG: electroencephalography; EMG: electromyography; fMRI: functional magnetic resonance imaging; fNIRS: functional near‐infrared spectroscopy; OD: oropharyngeal dysphagia; PET: positron emission tomography; rs‐fMRI: resting‐state functional magnetic resonance imaging; rTMS: repetitive transcranial magnetic stimulation; SCR: skin conductance response; sMRI: structural magnetic resonance imaging; TRP: transient receptor potential; VBM: voxel‐based morphometry.
7.3.3 Association Between Swallowing and the Brain in Older People
Compared to mastication, more studies have been published for the association between aging and brain mechanisms of swallowing (Humbert et al. 2009; Malandraki et al. 2010, 2011; Martin et al. 2007; Teismann et al. 2010). The following section primarily focuses on the age‐related brain mechanisms for healthy and non‐dysphagic older people. The brain mechanisms regarding the development and therapy of dysphagia are discussed in Chapter 8.
7.3.3.1 Aging and the Brain Mechanisms of Swallowing
There have been more studies published for the association between aging and brain mechanisms of swallowing compared to mastication (Humbert et al. 2009; Malandraki et al. 2010, 2011; Martin et al. 2007; Teismann et al. 2010). Two earlier task‐based fMRI studies have reported a distinct pattern of brain activation associated with swallowing in older people, using different protocols of stimulation. Martin et al. (2007) investigated the brain activation of water and saliva swallowing in healthy older subjects (mean age 74.2 years) and found common activation at the M1/S1 and the SMA. Notably, they found a greater activation for water swallowing compared to saliva swallowing in the PMC and the PFC (Martin et al. 2007). Humbert et al. (2009) compared the brain activation of water, saliva and barium swallowing between younger and older subjects (mean age 72.3 years). They found that older subjects showed a greater activation at a widely distributed area, including the bilateral PFC and the bilateral parietal regions, compared to younger subjects. Notably, the activation of the S1/M1 showed a distinct pattern between hemispheres: higher activation in older (vs. younger) subjects in the right S1/M1, but higher activation in younger (vs. older) subjects in the left S1/M1 (Humbert et al. 2009). However, the study did not identify an extensive activation during water swallowing compared to saliva swallowing, as reported previously (Martin et al. 2007). Using magnetoencephalography (MEG), Teismann et al. (2010) reported that during swallowing older subjects showed an increased bilateral activation at the somatosensory area compared to younger subjects. The laterality of brain activation has been further investigated by Malandraki et al. (2010), who performed a region‐of‐interest (ROI) analysis on the brain activation of S1, the M1 and the PMC, associated with water swallowing. The authors found a greater activation at the right hemisphere in younger subjects during swallowing. In contrast, in older subjects (mean age 70.2 years), there was no significant difference between hemispheres (Malandraki et al. 2010). Furthermore, the activation of the PMC was identified during the preparatory stage of swallowing, which may be associated with motor planning of the movement (Malandraki et al. 2010). A subsequent ROI analysis reveals a generally decreased activation in the S1 and the M1 during water swallowing in older subjects compared to younger subjects (Malandraki et al. 2011). In contrast, during planning (i.e. anticipating to swallow), decreased activation was found only in part of the S1 (Malandraki et al. 2011). Together, the findings suggest that different brain mechanisms in motor control may underlie the age‐related effect on swallowing.
The results from the earlier studies, though not revealing a consistent finding of the age‐related brain mechanisms of swallowing, suggest several important clues about aging and swallowing. First compared to younger adults, older adults did not show an overall ‘reduction’ of brain activation in all areas associated with swallowing. They have distinct patterns of activation with some regions (particularly the PFC) showing a greater activation than younger subjects. Secondly, in older subjects, multiple regions (other than the S1/M1) would participate in swallowing, such as the PFC, the parietal, the temporal and the cerebellar regions. The behavioural meaning of such a sophisticated pattern of activation remains unclear. A potential explanation is that for older adults more efforts (e.g. attention and cognitive control) are demanded to maintain swallowing. Older adults would show a more symmetrical activation of brain regions as compensatory mechanisms of the brain (Malandraki et al. 2010), and such an ‘overactivation’ may reflect an increased task demand in older adults (Humbert et al. 2009). In general, the earlier findings revealed that swallowing, like mastication, was associated with both qualitative and quantitative differences between age groups.
7.3.3.2 Recent Neuroimaging Findings of Aging and Swallowing
The neuroimaging findings published more recently are summarized in Table 7.2. Using fMRI, Windel et al. (2015) investigated the brain activation associated with water swallowing in older adults. They investigated the brain activation associated with the movement of the thyroid cartilage, a critical feature of swallowing movement. Moreover, subjects’ skin conductance response (SCR), as an index of emotional arousal during swallowing, was recorded (Windel et al. 2015). In older subjects, there was higher activation in the PFC (BA10) compared to younger subjects. Notably, older subjects also showed a higher SCR compared to younger subjects, which may reflect a higher level of autonomic arousal and cognitive efforts during swallowing. In older subjects, a higher SCR was associated with higher activation in the inferior frontal cortex (i.e. BA45), the cingulate cortex, the insula and the hippocampus, as well as the S1/M1 area. The strong association between the SCR and the extensive brain regions outside the S1 and the M1 may reflect increased recruitment of attentional resources and an increased emotional valence of swallowing (Windel et al. 2015). Using electroencephalography (EEG), Rofes et al. (2017) investigated the event‐related potential (ERP) associated with the electrical stimulation at an intra‐pharyngeal site. They found decreased activity in the parietal area (including the S1 and the M1) and frontal area in older (non‐dysphagic) subjects compared to younger subjects. In contrast, older patients with dysphagia show decreased activity in the parietal area but increased activity in the frontal area compared to older non‐dysphagia subjects (Rofes et al. 2017). In terms of the structural features of swallowing, Lin et al. (2019) found that in cognitively healthy older adults, the GMV and regional volume of the posterior cerebellum were positively correlated with swallowing performance, as quantified by the repetitive saliva swallowing test (RSST). Notably, the posterior cerebellum is associated with not only motor control but also cognitive processing of movement (Stoodley and Schmahmann 2010). The findings strengthen the connection between swallowing and the cognitive–affective processing in older adults.
There have been more studies that investigate the interventional effect on improving swallowing compared to mastication (Table 7.2). For example, using positron emission tomography (PET), Park et al. (2017) identified a cortical activation of the bilateral sensorimotor cortex during swallowing in non‐dysphagic older adults. For older patients with dysphagia, they found that repetitive transcranial magnetic stimulation (rTMS) for two weeks would improve patients’ pharyngeal motor function and showed increased activation at the bilateral M1, PMC and the right PFC (Park et al. 2019). In contrast, the effect of rTMS was not significant in non‐dysphagic older adults (Park et al. 2017). Another strategy for improving swallowing functions is to improve the sensory processing of the pharynx, which is key to the reflexive movement during the pharyngeal phase of swallowing. Tomsen et al. (2019) manipulated the sensory processing using oral capsaicin treatment. They found that the patients who received capsaicin treatment showed an improved swallowing function compared to the placebo group. The improvement was associated with changes in the latency and amplitude of pharyngeal ERP (Tomsen et al. 2019). Furthermore, the researchers found that in patients with dysphagia, the stimulation of TRPA1 (an ion channel associated with airway irritation) would improve swallowing function, which was also associated with changes in pharyngeal ERP (Tomsen et al. 2020). These clinical findings, being limited in a smaller sample size and the heterogeneity of clinical conditions, highlight the role of the brain in the rehabilitation of swallowing functions.
7.3.4 Considerations of Research Design and Future Directions
The current neuroimaging evidence, though providing several important clues about the age‐related brain mechanisms of oral functions, has not formed a full‐fledging theoretical framework for explaining the association between aging and oral functions. From a clinical perspective, such a framework should be able to (i) form a better prediction of individual differences in oral functions, (ii) help evaluating the oral functions of older people with neurological deficits and (iii) help evaluating the treatment effect on oral dysfunctions (e.g. dysphagia). The current neuroimaging evidence has identified several brain regions associated with aging and oral functions, but the results have not been fully translated into clinical application. In order to bridge this gap, two general issues for future research are discussed in the following sections.
7.3.4.1 The Definition and Selection of the Study Group
One of the major limitations of the current studies is the lack of subjects from a broader age group. As shown in Table 7.2, most of the studies on mastication focused on patients aged from 55 to 70 years. Few studies focused on an even elder group (e.g. over 85 years old) and comparison between the ‘young–old’ vs. ‘old–old’ subgroup is still uncommon. It should be noted that not all the oral functions ‘decline’ with the same time course. For example, the MP may not drop dramatically until 70–80 years old (Lin et al. 2017a), while severe periodontitis, a major cause of tooth loss, may start even in the middle age (Kassebaum et al. 2014b). While most of the studies focused on a comparison between an ‘older’ (e.g. aged > 65 years) and a ‘younger’ group, a comparison between different age sub‐groups within elderly people may provide more information about the age‐related effect on oral functions. Another limitation of the current studies is the lack of a longitudinal dataset, especially the dataset consisting of general physical and mental functions. As shown in Chapter 8, individual physical and mental functions may form a critical ‘reserve’ for older people to adapt to changes in oral conditions. A better collection of the physical and mental functions also makes it feasible to predict oral functions in older people with physical/neurological deficits.
7.3.4.2 The Design for Functional Assessment
Another critical issue regarding experimental design is the assessment of masticatory and swallowing functions. As discussed in Chapter 4, most of the current studies focus on a comparison between task conditions, such as ‘chewing vs. resting’ or ‘swallowing vs. no swallowing’. Therefore, brain activation can be interpreted as the results related to one state but not the other. However, from the physiological perspective, both mastication and swallowing consist of multiple stages, and a comparison between different tasks cannot elucidate the dynamic changes between different stages. Moreover, aging may affect the interaction between the stages. For example, older subjects may require a longer preparatory stage for swallowing compared to younger subjects, even though they both show normal swallowing during the pharyngeal phase. In order to elucidate age‐related effects on the dynamic progression of mastication and swallowing, the neuroimaging tools with a higher temporal resolution (e.g. EEG and MEG) may be suitable to disentangle the brain activity linked to different stages or events during mastication and swallowing. Continuous recording of the physiological activities related to oral functions (e.g. jaw and tongue movement) during neuroimaging is pivotal to clarify the dynamic mechanisms.
7.3.5 Summary
- Neuroimaging studies of brain activation of mastication have revealed that mastication is not only associated with the S1/M1, consistently in all age groups, but also a more widely distributed brain regions, including the PMC/SMA, the PFC, the cerebellum, the thalamus and the insula.
- Task‐based fMRI studies reveal that older adults showed both lower activation (e.g. in the S1/M1) and higher activation (e.g. in the PFC) compared to younger adults during chewing.
- The recent findings suggest a potential association between individual differences in oral conditions (e.g. tooth loss and MP) and structural brain features. Moreover, the association may be mediated by both situational (e.g. wearing a denture) and personal (e.g. aging and cognitive status) factors.
- In older subjects, multiple regions (other than the S1/M1) would participate in swallowing, such as the PFC, the parietal, the temporal and the cerebellar regions, which may reflect that more efforts (e.g. attention and cognitive control) are demanded to maintain swallowing.
- A better definition of research subjects and design of functional assessment of neuroimaging research may help to form a framework for a better prediction of individual differences in oral functions, for an evaluation of the oral functions of older people with neurological deficits and for an evaluation of the treatment effect on oral dysfunctions (e.g. dysphagia).
7.4 Association Between Oral Conditions and Neurodegenerative Disorders
7.4.1 Introduction
Neurodegenerative disorders such as AzD and PD are one of the most challenging issues in geriatric healthcare. AzD and PD are featured with the pathological accumulation of characteristic proteins, which form insoluble aggregates and affect neurons and glial cells (Brettschneider et al. 2015). Both AzD and PD are well known for their high prevalence worldwide (Scheltens et al. 2016). Patients with neurodegenerative disorders would gradually lose their abilities of self‐care in daily life. For example, patients with moderate‐to‐severe AzD may have difficulty to independently carry out daily activities, such as eating and cleaning. The increased demand for caring leads to a great economic burden on the medical system and society (Dorsey et al. 2013). One of the key issues of dental patients with neurological disorders is their declined oral health (Cerutti‐Kopplin et al. 2016; Tada and Miura 2017; Tonsekar et al. 2017; Wu et al. 2016). The section focuses on cognitive impairment and AzD. The association between oral health and cognitive impairment, and the potential brain mechanisms underlying the association, is outlined in the following sections.
7.4.2 The Oral–Cognitive Association
The association between neurodegenerative disorders and declined oral health has been reported in various clinical and epidemiological studies, which have been summarized in previous reviews (Cerutti‐Kopplin et al. 2016; Tada and Miura 2017; Tonsekar et al. 2017; Wu et al. 2016). While some researchers confirmed the association between worse oral factors and worse cognitive conditions (Cerutti‐Kopplin et al. 2016; Tada and Miura 2017), others considered it inconclusive based on current evidence, which is mostly cross‐sectional and correlational (Tonsekar et al. 2017; Wu et al. 2016). In the following section, we briefly outline the status quo of the research and highlight the reason why neuroimaging plays a key role in clarifying the oral–cognitive association.
7.4.2.1 Clinical Findings of Oral–Cognitive Associations
When it comes to the oral–cognition association, it is worthy of reviewing one of the earlier reports of the topic, i.e. the 2007 research derived from the famous ‘Nun study’, a cohort study for clinical risk factors of AzD. In this study, Stein et al. (2007) analyzed 144 subjects for their medical and dental records over approximately 12 years. They found that ‘a low number of teeth increased the risk of higher prevalence and incidence of dementia’ (Stein et al. 2007). As usually cited in the following studies (e.g. Cerutti‐Kopplin et al. 2016), the occurrence of new dementia cases (i.e. the incidence) was higher in the group with fewer (0–9) non‐third molar teeth compared to the group with more (≱10) non‐third molar teeth (Stein et al. 2007). However, in the original study by Stein et al. (2007), several key findings were less cited and largely neglected. Firstly, at the initial stage of the longitudinal investigation (i.e. the time when subjects received the first cognitive examination), the association between the number of tooth loss and dementia was not significant after adjusting for systemic factors. Secondly, they found an association between the tooth–dementia association and the polymorphism in the apolipoprotein E4 allele (APOE4) allele: in the subjects without APOE4, a lower number of teeth were associated with dementia prevalence (Stein et al. 2007). These critical findings suggest that dementia was not only associated with tooth loss. There may exist an interaction between oral factors and individual factors (i.e. the APOE4 genotype). Cumulating evidence has suggested that AzD is associated with multiple factors that are interwoven in a complex relationship (Scheltens et al. 2016). For example, another earlier longitudinal study over two to three years revealed that changes in SFR, but not the number of missing/decayed teeth, significantly decreased in patients with AzD compared to healthy controls (Ship and Puckett 1994). In the five‐year longitudinal study based on 1566 non‐demented older subjects (aged ≱60 years) in the Hisayama area, Japan, the association between the number of remained teeth and the risk of AzD was only borderline (p = 0.08) (Takeuchi et al. 2017). Another recent study from the Netherlands on 114 older subjects with dementia has revealed that their MP was negatively correlated with the score of general cognition. However, the association was not established when the factor of independence of daily activities was included (Weijenberg et al. 2015). Finally, a recent study on 537 older subjects (aged ≱65 years) revealed that those who had at least one APOE4 allele and fewer than nine teeth would show an increased risk of mild memory impairment (MMI) compared to those who had neither factor. Notably, the risk did not show a significant increase in the subjects with either one factor alone (Okamoto et al. 2017). These findings did not totally falsify or support the oral–cognitive hypothesis as recently debated. However, the evidence suggests that tooth loss is not the only (or even the major) factor contributing to cognitive impairment. Instead, oral factors can be one of the multiple factors that are associated with cognitive impairment. AzD would be associated with the interaction between the oral factors and other systemic factors rather than the oral factors per se.
7.4.2.2 Revisiting Oral–Cognitive Associations
What needs to be clarified first is the question itself: what are the objects to be studied in terms of the oral–cognition association? While some research focuses on the association between very specific conditions, such as the association between ‘tooth loss’ and ‘dementia’ (Thomson and Barak 2020), other research focuses on the association from a broader scope, such as the association between ‘oral health’ and ‘cognitive status’ (e.g. Wu et al. 2016). The different associations (in plural form) should be defined respectively. For example, if ‘cognitive status’ is the condition one hopes to investigate, we may need to define what mental functions are included in the cognitive status. Some studies (predominantly animal research) may focus on the function of spatial memory and learning (Fukushima‐Nakayama et al. 2017). A conclusion that ‘tooth loss may impair spatial memory’ from animal research should not be over‐interpreted as ‘a decline of cognitive functions (of all aspects)’ since spatial memory is part of the cognitive functions (Lin 2018b). A precise definition of the association also helps to avoid a premature claim of the cause–effect relationship between the variables. The term ‘declined oral health’ may represent multiple deficits or suboptimal oral conditions. For example, a recent study in Spain reported that patients with AzD showed poor periodontal health, a lower SFR and a higher incidence of candida infection compared to non‐AzD controls (Aragón et al. 2018). A longitudinal study in Japan with a larger sample size (N = 1566) revealed that tooth loss is a risk factor for all‐cause dementia and AzD. However, the proportion of tooth loss also covaried with other oral factors, such as the frequency of tooth brushing and attending dental care, as multiple systemic factors (Takeuchi et al. 2017). Therefore, it should be noted that there may exist multiple versions of the oral–cognitive association, from a narrower but clearly defined version (e.g. the association between tooth loss and deficits in spatial memory) to a broader but vaguely defined one (e.g. the association between oral health and mental health). From the point of research design, the different aspects of the oral–cognitive associations should be investigated with different research approaches.
7.4.3 The Role of the Brain in the Oral–Cognitive Associations
From the perspective of the brain–stomatognathic axis (BSA), the oral–cognitive associations can be examined from three aspects, which respectively correspond to some testable hypotheses proposed previously (Lin 2018b). As mentioned in Chapter 1, under the framework of the BSA, the brain, the stomatognathic system and feeding behaviour are bidirectionally linked (Figure 7.1a). The framework is based on the oral‐brain‐behaviour (OBB) model, which highlights both the CNS modulation on oral functions and the sensory feedback from the stomatognathic system, which together maintains feeding behaviour. Moreover, the BSA framework highlights the role of our brain in cognitive, affective and motivational processing of the adaptation related to feeding behaviour. These mental functions are crucial for individuals to adapt to changes in oral conditions. In addition, the brain also plays a key role in the behaviour of oral healthcare, which in turn influences the structural and functional integrity of the stomatognathic system. In the following sections, we discuss how the BSA framework helps to clarify some major postulations regarding oral–cognitive associations.

Figure 7.1 Conceptual links between oral health and cognitive functions. (a) The framework of the brain–stomatognathic axis (BSA). The framework highlights that the brain plays a key role in behavioural adaptation in feeding and oral care behaviour, which further relates to oral health. (b) The potential role of the brain in behavioural adaptation. Poor oral health may be attributed to increasing difficulty in conducting health‐related behaviour (e.g. being unable to brush teeth), which is derived from neurodegenerative disorders. (c) The potential role of oral factors in brain pathologies. The ‘oral‐on‐brain effect’ may be associated with multiple factors, such as reduced sensory feedback from the loss of occlusal contact or increased periodontal inflammation/infection. Notably, when the brain is affected by poor oral health, it may be followed by worse adaptation of feeding and oral care behaviour, which furthers exacerbates one’s oral health. (d) The potential role of a common factor that affects both cognitive and oral functions. For example, aging is associated with structural and functional alterations of the cerebellum, which relates to not only oral sensorimotor functions but also cognition. The arrow filled with a slash pattern denotes the potential causal links of the framework.
7.4.3.1 An Emphasis on the Brain’s Role in Behaviour Adaptation
Firstly, poor oral health may be attributed to more difficulty in conducting health‐related behaviour (e.g. being unable to brush teeth) (Figure 7.1b). The frequency to self‐care one’s oral hygiene decreased in patients with neurodegenerative disorders (Aragón et al. 2018). Notably, cognitive impairment may also relate to a declined ability of behavioural adaptation, such as learning a new motor skill. In older people, sensorimotor adaptation and learning (see Box 7.1) demand a great cognitive effort, such as an increased attention control on a sensorimotor task (Seidler et al. 2010; Ward 2006). For example, during eating, older individuals may spend more time chewing and pay more attention to their oral conditions during chewing (Peyron et al. 2004; Zelig et al. 2019). Therefore, patients with neurodegenerative disorders may be less able to adopt new skills for maintaining oral hygiene, thus leading to worse oral conditions.

VIDEdental - Online dental courses
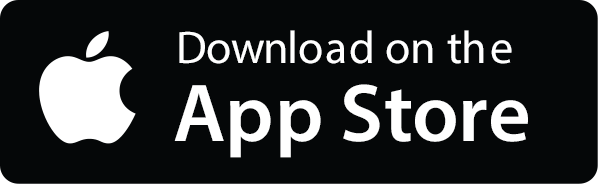
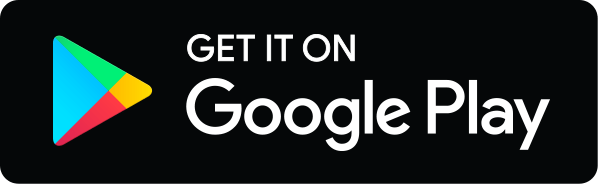