Fig. 16.1
TEM image of a multi-walled CNT
The introduction of MWCNTs into the HAp matrix and dip coating of nanocomposite on titanium alloy (Ti-6Al-4V) plate was applied by Abrishamchian et al. [38] in an attempt to improve the performance of the HAp-plasma-coated implants. They noticed that the addition of low concentrations of MWCNTs to HAp improved the mechanical properties and reduced the surface roughness of the coating. Furthermore, biological evaluation revealed normal cell adhesion and growth process on the HAp/MWCNT composite coating, comparable with that of pure HAp coating.
The in vivo behaviour of plasma-sprayed CNT-reinforced HAp coating on titanium alloy implants embedded in rodents’ bone was examined by Facca et al. [39], who observed no adverse effects or cytotoxicity attributed to the addition of CNT to bone tissues. Balani et al. [40] also investigated the non-toxicity of HAp-CNT coating on Ti-6Al-4V implants, and the results of those cell culture studies showed unrestricted growth of human osteoblast hFOB 1.19 cells near CNT regions.
The in vitro bioactivity of a HAp-TiO2-CNT nanocomposite coating was investigated by Zhang and Kwok [41], who observed that, after immersion in Hanks’s solution for 4 weeks, thick layers of apatite were formed on the surfaces of the monolithic HAp and HAp-CNT and HAp-TiO2-CNT nanocomposite coatings. They noted that the addition of TiO2 and CNT in HAp did not affect the apatite-forming ability on their surfaces.
16.3.2 Collagen
Considered to be one of the most useful biomaterials for applications such as tissue scaffolding, collagen is readily available and biocompatible and exhibits properties such as negligible cytotoxicity and hydrophilicity and has good haemostatic properties [42]. The seminal experiment that demonstrated bonding of bone collagen to bioactive glass composition 45S5 was published by Hench et al. in 1971 [43]. Bone is a composite of an organic collagen matrix and inorganic calcium phosphate crystal reinforcements which have inspired researchers to theorise that a collagen-calcium phosphate composite coating mimicking the unique nanocomposite structure of native bone tissue could present an added value over the individual components. Fan et al. [44] and later Wahl et al. [45] hypothesised that the poor fracture toughness of calcium phosphate can be compensated by the ductile properties of collagen and the use of collagen-calcium phosphate composite coatings also offers an added advantage in that the coating resembles the unique nanocomposite structure of native bone tissue.
Uezono et al. [46] investigated bone formation around Ti samples with a machined surface, HAp coating, and HAp-collagen nanocomposite coating. Their histomorphometric analyses revealed that the HAp-collagen group had the greatest bone contact ratio among all candidates. Furthermore, the results from bonding strength tests indicated that the HAp-collagen group exhibited the greatest bonding strength to bone.
In an attempt to improve the anchoring and long-term behaviour of Ti implant materials, nanoscale calcium phosphate and collagen-calcium phosphate composite coatings were examined by de Jonge et al. [47]. The biological property of the coatings was characterised by in vitro cell culture. According to their findings, improvements in both osteoblast differentiation and mineral deposition were most prominent when collagen was co-deposited with calcium phosphate. Osteogenic effects were also displayed for coatings with thicknesses well below 100 nm.
16.3.3 Titanium Dioxide (TiO2)
The use of titanium dioxide or titania (TiO2) as a bioactive coating has attracted much interest, and it was believed that using TiO2 within the coating as reinforcement was one method for improving the mechanical reliability of HAp. Furthermore, it was also believed that TiO2 is capable of inducing cell growth and enhancing osteoblast adhesion [48, 49]. In 1999, Webster et al. [48] observed an increase in osteoblast adhesion with decreasing titania grain size, correlating to the greater surface area displayed by the nanomaterial. They hypothesised that an increase in the surface area would lead to an increase in osteoblast adhesion. To improve the strength and adhesion of the coating, to reduce loosening of implants due to failure at the metal-coating interface, and to increase biological responses, researchers have used various approaches to fabricate a TiO2-HAp nanocomposite coating. Such a coating is capable of supporting osteogenic differentiation of osteoblast-like and mesenchymal stem cells (MSCs) [50–52].
Kuwabara et al. [51] attempted to investigate the biological response associated with bone formation on HAp-TiO2 composite coatings with a thickness of approximately 100 nm. Rat bone marrow-derived osteoblast-like cells were cultured on the coated surfaces with different electric charges. They discovered surfaces coated with both HAp and TiO2 have greater effects on cell attachment and spreading, as well as bone-titanium integration, as compared with either HAp- or TiO2-coated surfaces. Dimitrievska et al. [52] also investigated the mechanical and biological properties of TiO2-HAp nanocomposite coatings. They also observed an increase in proliferation and osteoblastic differentiation of human mesenchymal stem cells (hMSCs) on the nanocomposite coatings.
16.4 Advances in Calcium Phosphate Scaffolds for Tissue Engineering
The development of bone tissue engineering in the past has been directly related to changes in materials and nanotechnology. While the inclusion of materials requirements is standard in the design process of engineered bone substitutes, it is also vital to incorporate clinical requirements such that clinically relevant devices can be engineered.
Traditionally, biological methods for the management of bone defects consist of autografting and allografting. In addition to design and material factors, four biologically important components are needed for bone regeneration: (1) a morphogenetic signal, (2) responsive host cells that will respond to the signal, (3) an appropriate carrier of this signal and delivering it to specific sites, (4) scaffolding for the growth of the responsive host cells, and a well-vascularised host bed [53–55]. The purpose of scaffolding material is to act as a carrier or template for implanted bone cells or other agents. They can also be used to induce the formation of bone from the surrounding tissues.
The process of bone regeneration is common to the repair of fractures. The incorporation of bone grafts, the skeletal homeostasis, and the cascading sequence of biological events are often described as the remodelling cycle. Stem cells have been incorporated into a range of bioceramics and, when implanted, can combine with mineralised 3-D scaffolds to form highly vascularised bone tissue. These nanoscale cultured cell-bioceramic composites can be used to treat full-thickness gaps in long bone shafts, providing an excellent integration of the ceramic scaffold with bone and a good functional recovery. In a HAp-tricalcium phosphate composite, it was observed that the nano-sheet architecture promoted primary human osteoblast adhesion as reflected by the activation of focal adhesion kinase and expression of actin in human osteoblasts [56].
Bioactive composite grafts consisting of bioactive ceramic filler in a polymeric matrix have attracted a significant amount of attention during the past three decades. These bioactive composite grafts are designed essentially to achieve interfacial bonding between the graft and the host tissues. HAp-collagen, HAp/polyethylene (PE), and HAp/Ti-6Al-4V are notable examples of bioactive composite grafts [57, 58]. Of particular interest is the combination of HAp with collagen as a bioactive composite, as this appears to be a natural choice for bone grafting [59].
Skeletal bones are composed mainly of collagen and carbonate-substituted HAp, both of which are osteoconductive components. A composite matrix, when embedded with human-like osteoblast cells, showed better osteoconductive properties compared to monolithic HAp and produced calcification of an identical bone matrix [60].
The reconstruction of bone tissue using nanocomposite bone grafts with composition, biomechanical, structure, physiochemical, and biological features that mimic those of natural bone is an objective to be achieved. It has been well established that natural bone is made up of platelike nanosized crystals of HAp grown in intimate contact with an organic matrix which is rich in collagen fibres. Utilising strategies found in nature to produce nanocomposite bone grafts has received much attention recently and is perceived to be beneficial over conventional methods. Several methods have been used for the production of collagen-HAp composite gels, films, collagen-coated ceramics, ceramic-coated collagen matrices, and composite scaffolds for spine and hard tissue repair [45].
Based on the works of Daculsi et al. [61] and LeGeros et al. [62, 63], Ebrahimi et al. [64] proposed the utilisation of nano-HAp-TCP biphasic composites and that simple incorporation of collagen may improve physical properties of biphasic calcium phosphate (BCP) scaffolds and increase their bioactivity. The dimensional shrinkage after sintering of large scaffolds was found to be lower than small ones and scaffolds with high nano-HAp ratios experienced higher dimensional changes than those with higher β-tricalcium phosphate ratios.
Various biodegradable synthetic and natural polymeric materials have been proposed by Reis et al. [57, 65] and investigated in conjunction with HAp as scaffolding materials for tissue engineering applications. Man-made or synthetic polymers possess a number of advantages such as the physical-chemical properties can be modified and, by changing the chemical composition, the degradation and mechanical properties can be tailored to specific needs. Another advantage of synthetic polymers is its ability to be bioactivated with specific molecules by combining side chains and functional groups.
16.4.1 Calcium Phosphate Nanocoated Coralline Apatite
A number of natural and synthetic bone-graft materials currently in use are produced from coralline HAp. As a result of the conversion process, commercial coralline HAp has retained coral or CaCO3 and the structure possesses nanopores within the inter-pore trabeculae, resulting in high dissolution rates.
Under certain conditions, these features reduce durability and strength, respectively, and are not utilised where high structural strength is required. To overcome these limitations, a double-stage conversion technique was developed by Ben-Nissan and co-workers [1, 2]. The method involves a two-stage application route whereby, in the first stage, a complete conversion of coral to 100 % HAp is achieved. In the second stage, a sol-gel-derived HAp nanocoating is applied directly to cover the meso- and nanopores within the intra-pore material, while maintaining the large pores for appropriate bone growth (Fig. 16.2).
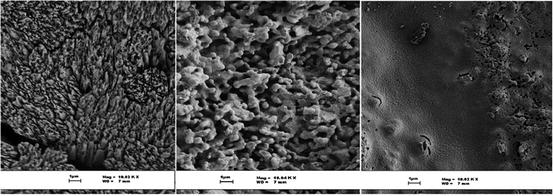
Fig. 16.2
Stages of nanocystalline HAp-coated coralline apatite formation. The first figure shows an enlarged micrograph of a coral skeleton spine area that has very sharp meso- and nanopore platelet regions. The middle micrograph shows surface morphological changes of the coral after conversion to hydroxyapatite, while the last one is the converted coral coated with HAp nanocoating, modifying the surface features to more bioactive nanoscale grains
Mechanical properties such as fracture toughness, Young’s modulus, and compression and biaxial strengths were each improved as a result of this unique double treatment. Application of the treatment method is expected to result in an enhanced bioactivity due to the nanograin size – and hence large surface area – that increases the reactivity of the nanocoating. It is anticipated that this material could be applied to load-bearing bone-graft applications where high-strength requirements are pertinent.
For these studies, the coral was obtained from the Australian Great Barrier Reef and contained micropores of 100–300 μm size. The coral was shaped and treated with boiling water and 5 % NaClO solution. A hydrothermal conversion was carried out in a Parr reactor with a Teflon liner at 220 °C and 3.8 MPa pressure with excess (NH4)2HPO4. A total conversion to HAp was achieved in this way. Nanocrystalline ceramic coatings were produced using the sol-gel process. For this, the precursor solution was formed using the previously reported method [1, 2]. Coatings were formed using these solutions, followed by subsequent heat treatments. Mechanical testing involved a standard, four-point bend test according to ASTM C1161, to measure the flexural strength and flexural modulus of the natural coral. Comparative compression and biaxial strength tests were also carried out.
Characterisation studies of the natural and converted corals using XRD, SEM, DTA/TGA, nuclear magnetic resonance (NMR), and Raman spectroscopy have been previously reported. These results showed a large increase in all mechanical properties, specifically the compression strength, due to hydrothermal conversion and nanocoatings methods. The bioactivity was enhanced through the nanocrystalline formation, due to the HAp nanocoating [66].
In addition to enhancing the bioactivity and the mechanical properties, HAp coatings can also be used in controlling the rate of drug release from coralline apatite delivery system. A bone-stimulator drug, simvastatin, was successfully loaded with the coral-derived β-tricalcium phosphate, and in an attempt to control the release of simvastatin and reduce its release rate, a lipid coating was utilised [67].
16.4.2 Liposomes and Calcium Phosphates
Liposomes are one of the most clinically established nanometer-scale systems currently employed to deliver non-toxic and antifungal drugs, genes, and vaccines. Liposomes consist of a single layer, or multiple concentric lipid bilayers, that encapsulate an aqueous compartment. When compared to other delivery systems, the outstanding clinical profile of liposomes is based on their biocompatibility, biodegradability, reduced toxicity, and capacity for size and surface manipulations [68].
The encapsulation of nanoparticles such as calcium phosphate within liposomes may lead to an increase in nanoparticle hydrophilicity, stability in plasma, and an overall improvement in their biocompatibility [68–70]. Moreover, by utilising the ability of liposomes to carry hydrophilic and hydrophobic moieties, combinatory therapy/imaging modalities can be achieved by incorporating therapeutic and diagnostic agents into a single liposome delivery system [68].
Based on their excellent biocompatibility, calcium phosphate-based hybrid nanoparticles have shown great promise as candidates for drug delivery and bone regeneration systems [71–73]. Recently, composite scaffolds composed of collagen and HAp with liposomes were proposed by Wang et al. [72] to provide a sustained drug release platform in bone regeneration and repair. The liposomes were composed of distearoylphosphodioline, cholesterol, distearoylphosphoethanolamine-poly(ethylene glycol), and a bone-binding bisphosphonate attached to the liposome. By taking advantage of the specific interaction between the liposomal bisphosphonate and the HAp incorporated into the scaffold, the bisphosphonate-decorated liposomes were shown to display a strong affinity to collagen-HAp scaffolds. They reported there were no differences in drug release from the liposomes whether the liposomes were bisphosphonate decorated or not. Whereas unencapsulated drugs and drugs encapsulated in PEG-liposomes displayed rapid release from the scaffolds, the drugs entrapped in bisphosphonate liposomes showed a slower release from the collagen-HAp scaffolds.
HAp-coated liposomes were successfully manufactured by Xu et al. [71] and filled with a model hydrophobic (lipophilic) drug, namely, indomethacin (IMC). In this process, the HAp layer was precipitated onto the liposomes, the aim being to provide the HAp-coated liposomes with two functions: (1) that the inner core liposome provides a sustained drug release and (2) that the outer HAp layer provides the osteoconductivity for bone cells. The liposomes were formed from 1,2-dimyristoyl-sn-glycero-3-phosphate (DMPA) and 1,2-dimyristoyl-sn-glycero-3-phosphocholine (DMPC). The results reported by the authors indicated that precipitating HAp onto the liposome reduced the release rate of IMC compared to uncoated liposomes. In fact, under the conditions used, the 5 h period required to release 70 % of the IMC from the liposomes was extended to 20 h when the liposomes were coated with HAp. Perhaps, more importantly, IMC release from the uncoated liposomes occurred more rapidly at pH 7.4 than at pH 4, whereas the HAp coating reduced the release rate at pH 7.4 compared to that at pH 4. Based on these findings, they suggested that this effect might open up the possibility of creating “smart” (pH-controlled) targeted drug delivery devices.
Osteoporosis, a degenerative bone disorder, is one of the leading causes of morbidity in the elderly. Lewis [73] examined the possibility of using liposomes to encapsulate various micro- and nanosized calcium-based mineral compounds for direct delivery to the bone which may be beneficial to bone health. Calcium phosphate mineral including HAp, dicalcium phosphate dihydrate, as well as multiphase and substituted calcium phosphates was produced using biomimetic process. Preliminary cell culture studies showed no direct effect on osteoblast-like Mg63 or Saos-2 cells or osteoclast resorption, measured by bone collagen release. Macrophage response was explored using U937 cell line. Expression of TNF-α and IL-1, markers of inflammation, increased with liposome treatments compared to the negative control but decreased compared the positive control. The Mg63 cells given U937 supernatants showed liposomes increased OPG production, but this was regardless of mineralisation (Fig. 16.3).
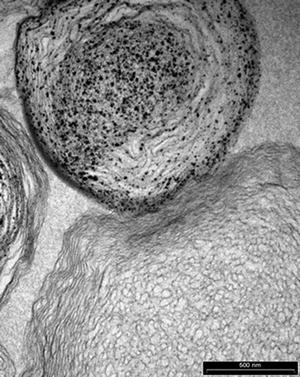
Fig. 16.3
Multilayer liposome with nano-HAp particles (Courtesy of Dr. K. Lewis)
The clinical significance of hydroxyapatite (HAp) as a bone substitute has become apparent in recent years, and bone morphogenetic protein (BMP) has attracted much attention. In a study conducted by Ono et al. [74], 1.2 cm-diameter bone defects created on rabbit cranium were treated with the BMP-2 gene (cDNA plasmid) introduced with porous HAp after completion of haemostasis, and the effect of using cationic liposomes as a vector to the amounts of new bone formation was examined. Four groups of rabbits were compared. In the HAp group, the cranial bone defect was treated with HAp containing 40 μg of liposomes and a dummy gene. The BMP gene HAp group was treated with HAp soaked in liposomes and 10 μg of the BMP-2 gene. Although new bone formation was evident surrounding the scaffold 3 weeks post-operation, the induced bone tissue did not fill all the pores of the scaffold even at 9 weeks post-operation. They hypothesised the clinical usefulness of gene therapy for bone formation, using the BMP-2 gene combined with cationic liposomes as a vector.
When Huang et al. [75] used liposome-coated HAp and tricalcium phosphate as bone implants in the mandibular bony defect of miniature swine; they found the liposome-coated materials to be biocompatible. Moreover, the clinical endpoint was enhanced compared to that in the absence of liposomes. It was hypothesised that HAP coating and tricalcium phosphate with negatively charged liposomes might improve the nucleation process for new bone formation. In experiments conducted in miniature swine, artificial bony defects on one side were implanted with either HAp-coated or tricalcium phosphate-coated liposomes, while defects on the other side served as controls. Histology and radiography performed at 3 and 6 weeks after surgery showed the coated liposome materials to be biocompatible. At 3 weeks, the implant material was surrounded by dense connective tissues, while by 6 weeks new bone formation was visible near the implanted material. Liposomes immobilised in agarose gel and implanted in the defects also showed new bony bridge formation.
16.4.3 Chitosan and Calcium Phosphate Composites
Despite being one of the most extensively investigated biomaterial in tissue engineering, the bioactivity of chitosan needs to be enhanced for specific tissues and making them not as ideal when used as a stand-alone material [76]. As a result, new composites are created by combining chitosan with other bioactive materials such as HAp [76–79]. Nano-HAp and its composites with variety of chitosan content were investigated by Tavakol et al. [76], and they observed that the degree of bone regeneration potential was greater in nano-HAp powder than in nano-HAp-chitosan composites. Cell culture studies were attempted by Tomoaia et al. [77] on fibrous biocomposite scaffolds made of nano-HAp particles, chitosan, and type I collagen. They noticed that the incorporation of small amounts of silicon, magnesium, and zinc within the nano-HAP lattice could improve the in vitro biological activity of human osteoblasts on these scaffolds. Im et al. [78] hypothesised that chitosan nanocomposite with both nano-HAp and single-walled carbon nanotubes (SWCNT) could significantly enhance the mechanical properties of the scaffold and synergistically improve scaffold cytocompatibility for osteoblast adhesion and proliferation. Nanocomposites containing magnetically synthesised single-walled CNT (SWCNT) had superior cytocompatibility when compared with nonmagnetically synthesised SWCNT. Their study also revealed that osteoblasts favoured magnetically synthesised SWCNT which has smaller diameters and were twice as long as their nonmagnetically prepared counterparts indicating that the dimensions of SWCNTs can have a substantial effect on osteoblast attachment.
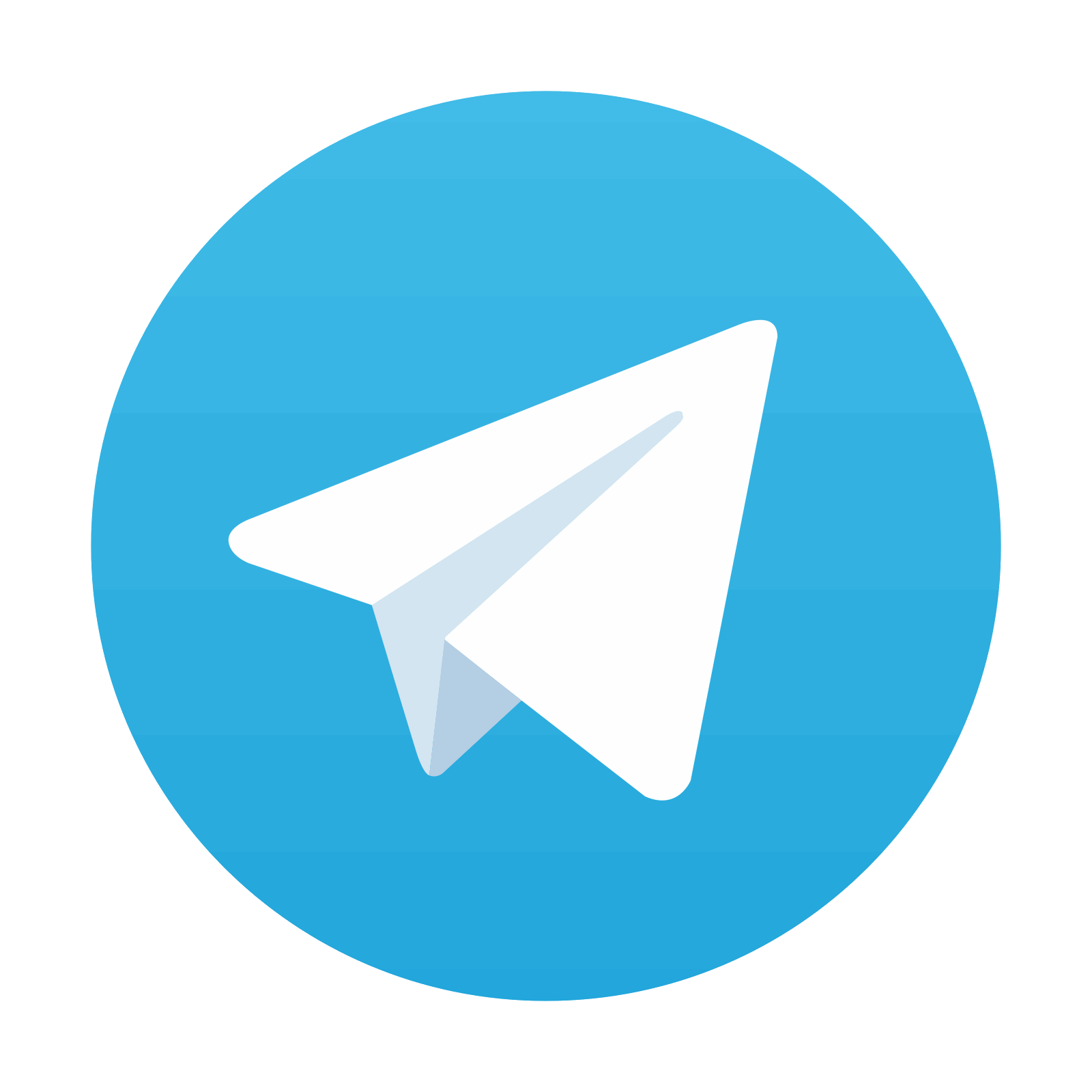
Stay updated, free dental videos. Join our Telegram channel

VIDEdental - Online dental courses
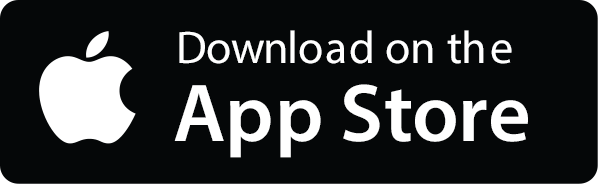
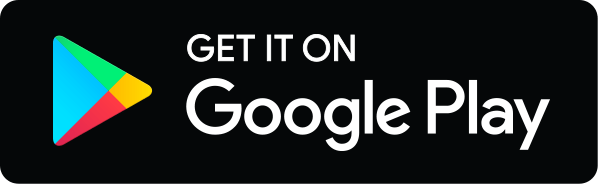