During mastication, the temporomandibular joint (TMJ) transmits forces to the base of the skull similar in magnitude to those generated by the biting force. For this reason, the disk is fibrous; cartilage would not be tough enough to take the load without breaking up. During incision the reaction force at the joint is higher than it is on the incisors. A bite force at the molars, which is the location where the maximum bite force is possible, causes a reaction on the joint components which are slightly less than during incision. The joint surfaces have a low coefficient of friction. In order to prevent the condyle, sliding out of control during function, a group of muscles maintains the stability of the joint. The glenoid fossa slopes in a posterior direction, and this also assists in maintaining the stability of the condyle. The tension generated by some of the masticatory muscles which stabilize the joint may explain why these muscles may become fatigued and painful. Pain in the masticatory muscles may be referred to the joint. The joint itself is seldom the source of pain unless it has experienced some trauma. In spite of this pain in the masticatory muscles and limited movement of the jaw, this condition is usually referred to as temporomandibular joint dysfunction or disorder. There has been considerable attention paid to this so-called dysfunction of the joint. Invasive treatments have been popular in the past including surgery to the joint. There is now reliable evidence that reversible noninvasive management of dysfunction is successful. The essential component of psychosocial factors in pain has to be recognized.
9.1 The Mechanics of the Temporomandibular Joint
9.1.1 A Load-Bearing Joint
The load lifted by a class 3 lever is applied at the end of the lever, separated from the fulcrum by the lifting force. The forearm, lifting a weight in the hand is an example. The further away the load is from the lifting force, the greater the reaction at the fulcrum in order to keep the lever stable (▶ Fig. 9.1). If we transfer this analogy to the jaws, the articular eminence acts as the fulcrum. The load is the point on the arch of teeth, where the bite is applied. There is no muscle force which acts directly through the load, as all the teeth are anterior to all the muscles. The muscle force is therefore partly resisted at the TMJ and partly at the teeth. In equilibrium, the reaction at the joint plus the resistance to the load is equal to the force applied by the muscle (▶ Fig. 9.2). As the load moves forward toward the anterior teeth, the bite force on the bolus decreases, but there is a greater load on the TMJ. The TMJ, like the fulcrum of a lever, is always under load whenever any bite force is applied.
Fig. 9.1 Diagrammatic representation of a class 3 lever and the forces required to keep it stable. (a) Diagram of a class 3 lever. When the lever is stable, the force (F) is balanced if the load (W) × the distance (dw) is equal to the reaction force R × dR. (b) If the load is moved further from the fulcrum, it has greater leverage and would pull the lever down. To keep the lever stable, the load must be decreased so that W × dw remains the same. (c) An alternative to balancing the system as the load moves away from the fulcrum is to increase the force (F). As F increases, it must be balanced by an equal increase of dR × R. As dR is constant, it is the reaction at R which is increased when the load is moved further from the fulcrum.
Fig. 9.2 Diagrammatic representation of the mechanics of the human jaw which works as a class 3 lever. (a) The fulcrum is at the joint (JR). When the jaw is stable, the muscle force (MF) is balanced by the bite reaction (BR) and the reaction force at the joint (JR). (b) If the bite action moves to the incisors, and the muscle force remains the same, the bite reaction (BR) decreases, but the reaction force at the joint (JR) is increased. The temporomandibular joint is thus a stress-bearing joint, and it receives its greatest forces when the bite is forward of the molars. A lack of posterior teeth may therefore stress the joint more than would be normal.
This analogy of the TMJ to a class 3 lever assumes that there is a single fulcrum, but of course, there are two condyles. We now have to view the jaws as a curved plate rather than a beam. The plate has a triangular shape, with a fulcrum at two corners, and the teeth along two edges. The muscles of mastication pull upward on both sides of the plate, slightly more powerfully on the chewing side (▶ Fig. 9.3). There is therefore a reaction at both joints during the generation of a bite force on one (working) side. On the balancing side, if no teeth meet, the reaction of the bite force is resisted only by the joint on that side. On the working side, the reaction is shared by the teeth and the joint. The simplified diagrams in ▶ Fig. 9.2 and ▶ Fig. 9.3 are accurate in that they identify the source of the three major vectors of jaw mechanics, namely the muscle force and direction, the reaction at the joint, and the load due to the resistance of the food bolus. They are oversimplified representation because all the component muscles are represented by a single force.1
Fig. 9.3 Diagrammatic representation of the mechanics of the human jaw in a coronal plane. The jaw can be viewed as a triangular plate with a fulcrum (joint) on two corners. The muscle force (MF) on the working side is stabilized partly by the joint reaction (JR) and the bite reaction (BR) through the bolus. However, on the balancing side, even though the muscle force (MF) is slightly less, there is nothing but the joint reaction (JR) to stabilize the muscle force on this side, if there is no tooth contact. This suggests that for man the balancing condyle is under a greater load than the working condyle, unless there are balancing side contacts during function.
9.1.2 Variable Forces at the Teeth
The load is not always a fixed distance from the joint and neither is the force generated by the masticatory muscle. Furthermore, the load may be applied to more than one tooth in the arch. By virtue of the arch of teeth being on a plane, which is offset from the joint, several teeth may contact at once. The requirements for efficient use of the teeth as tools may demand that forces are not always at right angles to the lever arm. For example, shearing forces at the teeth require forces applied at an angle to the lever. The most important consideration during chewing is to develop the appropriate magnitudes and direction of force at the teeth.
9.1.3 Joint Stability
The joint surfaces have an extremely low coefficient of friction; in other words, they are slippery surfaces. The joint surfaces therefore allow easy movement under load, but the slipperiness needs to be controlled. If you are skating on ice, you are most stable when your center of gravity is over, that is perpendicular, to your feet. The mandibular condyle is stable when the reaction at the joint is perpendicular to the surface of the eminence. In a simple masticatory system, with predictable muscle forces and a limited variation in load position and direction (e.g., a rat’s incisor), the jaw could be stabilized if the contour of the joint surfaces was at right angles to the joint reaction. In humans, the jaw closing forces are generated by a number of muscles, each with a different origin, insertion, and direction of tension and each with more than one anatomically distinct part. Each anatomical section of a muscle has hundreds of separate functional units. It is clear that depending on which combination of these muscle units is active, a different force at the teeth and a different reaction at the joint will be produced.
The forces generated at the teeth will be influenced by tooth wear. The greater the cusp wear, the flatter the grinding surfaces and the more lateral the masticatory forces. It is not surprising, therefore, that the articular eminence has been found to remodel, becoming less steep as tooth wear occurs.2 It is possible that this remodeling is a result of changes in the directions of forces applied to the teeth, which adapt to changes in cusp angles brought about by tooth wear.
Given the complexity of the force, load, and hence joint reaction in man, it is clear that the resultant of the three major force components is highly variable. If the reactions at the joint are not perpendicular to the eminence, then movement, either up or down the eminence will occur.
Such sliding movement may be contrary to that required at the teeth and would have to be controlled. In view of the complexity of the balance between the force components of the jaws, Osborn and Baragar developed a computer model of the forces involved in biting and have been able to predict those muscles which would be most efficient in producing a bite force at the molar and at the incisors.3 The model also predicts what muscle action would be necessary to stabilize the jaw while the force was being produced at the teeth (▶ Fig. 9.4).
Fig. 9.4 Diagrammatic representation of the mechanics of the influence of control muscles on the stability of the temporomandibular joint. (a) The direction of some of the groups of jaw closing muscles which provide a power force (pf). They include vertical temporalis (pf-vt), superior masseter (pf-sm), deep masseter (pf-dm), and medial pterygoid (pf-mpt). The jaw joint slides readily and is only stabilized during a static bite force when the joint reaction (JR) is at right angles to the joint surface. (b) The control muscles are well placed to provide a stabilizing force (sf-) which influences the resultant muscle force (RMF) so that the joint reaction (JR) is at right angles to the joint surfaces. These stabilizing muscles are the lateral pterygoid (sf-lpt) and horizontal temporalis (sf-ht). (Adapted from Osborn and Baragar 1985.3)
The two muscles, which may have the greatest ability to stabilize the reaction at the joint, are the lateral pterygoid and the horizontal fibers of the temporalis muscle. Contraction of the lateral pterygoid would resist the condyle sliding backward up the slope of the articular eminence; conversely, contraction of the horizontal fibers of temporalis would resist the condyle sliding forward. The muscles which are well positioned to provide powerful closure are the deep and superficial masseter groups, the medial pterygoid, and the vertical fibers of the temporalis muscle.
The muscles of the human jaw can be therefore be divided into two major groups. Firstly, those whose prime function is to generate forces in the desired part of the arch in the necessary direction and magnitude to allow the teeth to function. These could be called the power muscles. Secondly, there are those muscles which control the movement of the condyle up and down the eminence and thus stabilize the joint. These are the control muscles.2 The jaw muscles are anatomically divided according to their ability to be visibly distinguished from each other. But we have noted that within muscles there are many functional units which can contract independently. The resultant of the activity of groups of these elements from different muscles is complex and continually alters as forces at the teeth are altered either in direction or magnitude.
9.1.4 Joint Instability and Muscle Fatigue
It follows from our analysis so far that as the destabilizing forces at the joint become more powerful, greater activity will be required by the stabilizing muscles to maintain condylar st1.0″. This relationship between destabilizing forces and the activity of stabilizing muscles may be of interest in understanding the source of muscle pains associated with the TMJ. Temporomandibular dysfunction (TMD) is a syndrome of signs and symptoms. The chief signs are limited jaw movement, particularly opening, and tenderness to muscle palpation. The main symptoms are a diffuse, unilateral pain around the region of the joint and ear, which increases when biting hard, and which may radiate across the face. The source of the pain in most cases may be traced to trigger points in the muscles of mastication from which pain is referred to the joint area. The lateral pterygoid muscle, although difficult to palpate, is the muscle most commonly reported to be tender. There is no satisfactory explanation for why this particular muscle should be so commonly affected, but it may be related to its role in stabilizing the joint. Patients are able to chew more comfortably on the same side as the pain than on the opposite side. From what is now known about joint loading, it appears that the joint on the same side as the working side has a lower reaction force than that on the balancing side. It therefore requires less effort from the controlling muscles, one of which is the lateral pterygoid.
9.2 The Structure of the Temporomandibular Joint
The joint structure is unusual, in that a fibrous disk separates the two joint surfaces into two compartments4 (▶ Fig. 9.5). The joint surfaces are also lined with dense fibrous tissue. This is in contrast to the cartilaginous disks and surfaces of other joints. While cartilage has a very high compressive strength, it has a relatively low tensile strength. As the human joint is loaded during sliding movements, shearing and tensile stresses are developed. The fibrous disk of the joint is therefore better suited to withstand these stresses than if it were composed of cartilage.
Fig. 9.5 Diagrammatic representation of the temporomandibular joint. Note that the disk separates the joint into an upper and lower compartment. The superior and inferior fibers of the head of the lateral pterygoid muscle insert into the neck of the condyle and the anterior margin of the disk, respectively. (Adapted from Rees 1954.4)
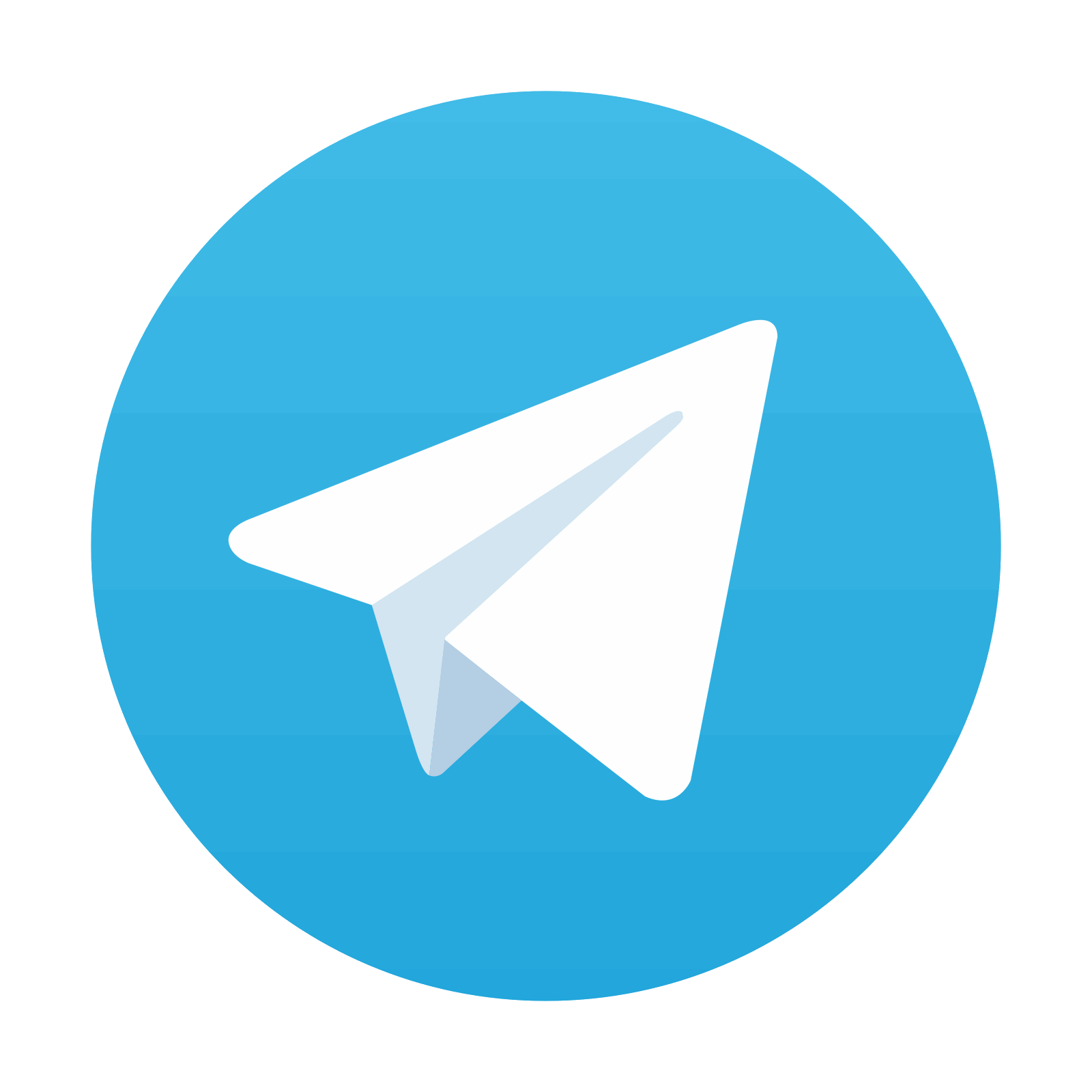
Stay updated, free dental videos. Join our Telegram channel

VIDEdental - Online dental courses
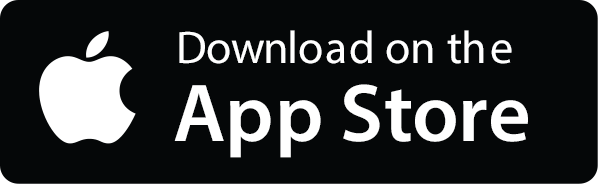
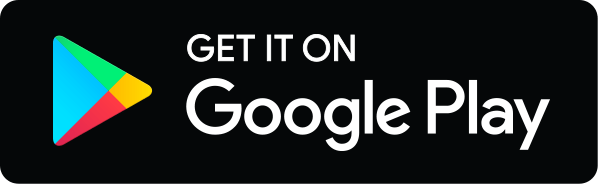
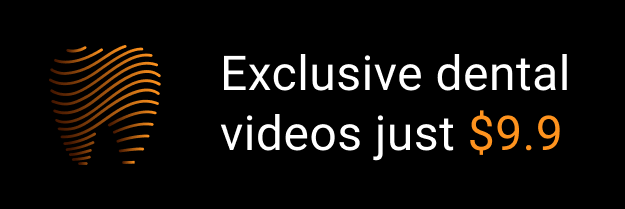