The oral cavity provides a variety of niche habitats for hundreds of species of oral bacteria. There are potential habitats on the teeth, tongue, or gingival sulcus and a constant supply of nutrients from food residues, saliva, and the products of other bacteria. The human host and its bacterial partners in the oral cavity and gut have a cooperative relationship which goes back millions of years. Cooperation between different bacterial species is just as important as competition in conserving this relationship; their interdependence holds together several different species in a consortium, a sort of mutual benefit community. If dental caries or periodontal disease occurs, it is always due to a disturbance in the dominance or hierarchy of bacteria within the consortium, often caused by something as simple as an increase of sugar in the diet, or at the other extreme, malnutrition. All the members of the consortium are well-established residents, so dental caries and periodontal disease are not the result of foreign pathogens but may be classified as noncommunicable diseases. The balance between the efforts of organisms to maintain growth on the oral surfaces, by both competitive and cooperative tactics, and the host’s factors which tend to support and tolerate certain commensals, but inhibit potential pathogens, is the theme of this chapter. Oral health strategies, which work best, are those based on an understanding of the principal factors which regulate and maintain a stable oral microbiome.
4.1 Introduction
Ecology is the study of the relationship between living organisms and their environment. An ecosystem is a specific environment in which plant and animal species live in an interconnected web of cooperation and competition. For example, a forest is an ecosystem in which trees, bushes, and smaller plants interact with insects, soil bacteria, birds, small mammals, and reptiles, in fact, a large spectrum of most major classes of living organisms. Within the forest, each tree could be seen as a small ecosystem on its own, as it supports a characteristic collection of interactive bacteria and insects. The complexity and stability of an ecosystem take a long time to develop. If a temperate forest were to be cleared to the ground, it is estimated that it would take 200 years to regain its original rich and varied number of species.
The oral cavity is an ecosystem on a smaller scale, which also requires time to acquire a mature ecological balance of organisms. The time is much shorter than that needed for a forest to mature, as the life cycle of bacteria is faster than trees, and there are only 500 or so species or bacteria in the mouth. However, it still takes 2 weeks before a film of plaque bacteria has reached maturity. The organisms which have established themselves in an ecosystem are those which have been able to adapt to the physical environment and to the other species in the ecosystem.
Not all the members on an ecosystem are of equal importance to its survival. Some, called keystone species, have a decisive influence even when they may represent a small proportion of the total numbers of organisms in the ecosystem. Keystone species determine the composition of the ecosystem and control the relative numbers of each species. An example of a keystone species is the sea otter. On the Western coast of North America, the otter keeps down the population of sea urchins which graze on the base of kelp. Without the sea otter the urchins would devour the kelp and destroy the entire ecosystem of the kelp beds.
There are keystone species in communities of bacteria, occurring in the gut and oral cavity, which communicate with their microbial community, via chemical messages, a communication which microbiologists have called cross-talk or quorum sensing. The chemical messages induce control of gene expression in the target organism. Communication between bacterial species is also observed in the spread of antibiotic resistance. Genes from bacteria which confer resistance to antibiotics are able to be shared with other species of bacteria.
Stability is a feature of successful ecosystems, and it is dependent on maintaining a balance in the hierarchy of member species of the system. This balance is maintained partly by the stability of the physical environment such as the availability of light, oxygen, and nutrients, but it is also dependent on the population control of each species. This control is achieved through processes of competition for resources and cooperation between species in maintaining a balanced and stable population.
The relationship between different species is not always competitive. In fact, cooperative partnerships are more significant in nature than competitive ones. In the long term (evolutionary length), competition for survival has not been the best strategy, as 99% of all the species that have ever lived are now extinct. However, there are some remarkable examples of cooperation which have lasted 3 billion years. The relationship between mitochondria and all nucleated animal cells started off as a host–parasite relationship. The cell gave shelter to the mitochondria which were able to use oxygen to produce high-energy molecules and thus could be a great benefit to an active cell. The partnership was so successful that it led to the evolution of the eukaryote cell, with nucleus, mitochondria, and cilia. The eukaryote cells enabled an unprecedented surge in evolution of multicellular forms of life. The mitochondria have retained their genetic identity over the millions of years of this partnership and their separate process of reproduction through the female lines of organisms. The relationship is still recognizable as a form of symbiosis, the living together of different organisms. There are many other examples of symbiotic relationships, which, as they are better understood, indicate that they are of significant importance. A close and highly interdependent relationship between bacteria is found in a biofilm. This is a dense aggregate of many species which have abandoned a solitary planktonic life for the benefits of a cooperative community existence.
4.2 The Oral Environment
The following section will describe the features of the oral environment which support and influence the ecology of the mouth. The oral cavity provides a range of fairly stable habitats for microorganisms. There is a plentiful supply of both oxygen and nutrients, and there are physical surfaces for attachment, although some surfaces are more liable to disruption than others. The oral mucosa, including the tongue and exposed tooth surfaces, is exposed to saliva flow and the disruption caused by shear forces which occur during swallowing and mastication. The surface epithelial cells of the oral mucosa are shed (desquamated) when mature and carry off with them any organisms which have colonized the cell’s surface (▶ Fig. 4.1). This process is particularly important for the health of the gingival sulcus, one of the most highly populated habitats in the oral cavity. This site provides shelter, and a rich supply of nutrients provided by the gingival crevicular fluid flowing out of the gingival sulcus. The cells of the junctional epithelium and gingival sulcus epithelium desquamate regularly, clearing away colonizing organisms. It has been noted in Chapter 3 Oral Mucosa and Periodontium that the turnover period of the junctional epithelium may be only a matter of days.
Fig. 4.1 The desquamation of the surface epithelial cells of oral mucosa. (a) A scanning electron microscope (SEM) image of a surface cell which has partly desquamated (magnification × 1,000). (b) A diagrammatic representation of the effect of desquamating epithelial cells on the control of colonization of epithelial surfaces by oral organisms.
For those organisms which are able to adhere to the exposed tooth surface, there are large areas to colonize. The sheltered tooth surfaces, such as the approximal areas and occlusal fissures, are more densely colonized than more exposed tooth surfaces, and it is these sites which have the greatest risk of developing dental caries (▶ Fig. 4.2). Oral organisms are unable to attach directly to tooth enamel, but they may adhere via an intermediary layer of proteins, the salivary pellicle.
Fig. 4.2 Oral habitats sheltered from salivary flow become readily colonized by oral organisms. An SEM image of a buccal/lingual section of a maxillary premolar. A pit in the center of the central fissure is a secluded habitat for bacteria. The proximity of the base of the pit to the dentin is an important factor in the progress of dental caries (magnification × 500). D, dentin; E, enamel.
The presence of a fixed restoration, which has defective margins, and a removable restoration, which covers the oral mucosa, provides a protected environment for organisms which allows the total mass to increase (▶ Fig. 4.3). When the teeth are lost, these habitats disappear and the oral flora is dramatically altered; in general, it is less diverse.
Fig. 4.3 SEM images of the surface of keratinized oral epithelium, the impact of a restoration. (a) The superficial squamous cells of the healthy epithelium of the hard palate have a pitted surface into which projections from the overlying cells interlock. The epithelial cells provide a habitat for oral organisms (arrows). (b) The palate of a complete denture was covering these epithelial cells. The surface microstructure has become altered, and there are large numbers and variety of oral organisms which have colonized this surface (magnification × 10,000).
4.2.1 Salivary Pellicle
Salivary pellicle is a thin layer (10 µm) of various salivary proteins which heap up on top of each other, on the surface of recently cleaned enamel, within a few hours. The smaller-molecular-weight phosphoproteins and sulpho-glycopeptides are the first to adhere to freshly cleaned enamel. Some of the phosphoproteins and calcium-binding proteins form ionic bonds with the apatite crystals of enamel. Other proteins adhere because bacteria have caused them to clump together; they are less strongly bound to the enamel surface. Most of the salivary proteins are rich in the amino acid proline and are collectively described as proline-rich proteins (PRPs). The coverage and composition of the pellicle change during its early formation. After the smaller-molecular-weight proteins, the larger glycoproteins adhere, and this stage is rapidly followed by the adhesion of the first oral organisms. Pellicle has the following influence on the oral environment:
-
It protects enamel from demineralization by providing a layer of proteins, which isolates the surface from changes in the acidity of fluids surrounding the tooth.
-
It influences the types of microorganisms which will adhere to the tooth surface.
-
It lubricates the enamel surface and may therefore reduce the rate of tooth wear (▶ Fig. 4.4).
Fig. 4.4 A diagrammatic representation of the role of salivary pellicle in adhesion of oral organisms. Calcium-binding phosphoproteins (yellow) bind onto calcium atoms of enamel. Larger glycoproteins bind to the smaller phosphoproteins to form a layer of salivary pellicle. Bacteria bind selectively to certain larger proline-rich proteins in pellicle.
Given a surface onto which oral organism are able to adhere, a further defining feature of the oral environment is the fluid surrounding the pellicle and bathing all the surfaces in the oral cavity. Oral fluid is a term which best describes the mixture of substances which might be found in a sample of fluid from the mouth. It would mainly consist of saliva from the major and minor glands, each with its characteristic feature. The oral fluid would also contain gingival sulcus fluid, desquamated epithelial cells, bacteria, and some blood cells, mostly neutrophils. It is difficult to detail the exact composition of saliva as the secretions from each gland are not identical, and they all vary with the rate of secretion of saliva.
4.2.2 How Saliva Defines the Oral Environment
The essential functions of saliva are both enabling and protective. Saliva enables mastication and speech as it lubricates the oral structures during function. It also protects enamel from demineralizing and enables a stable ecology of oral organisms to live as commensals while excluding more overtly pathogenic bacteria.
Lubrication: Lubrication is provided by mucins (glycoproteins) which are selectively adsorbed to mucosa and enamel. On mucosa, they protect the tissues against drying, irritants, and bacterial enzymes. Together with the water content of saliva, glycoproteins provide lubrication for speech and mastication and preparation of a food bolus which can be readily swallowed. It appears to be the carbohydrate part of the glycoproteins, which is important for lubrication. (see Chapter 6.2 Glycoproteins)
Mechanical cleansing: The flow of saliva and subsequent intermittent swallowing provide a flushing system which removes food particles, bacteria, and desquamated cells from the mouth. The rate at which this removal occurs is referred to as the clearance rate. The more complete the fluid eliminated at each swallow, the more rapid will be the clearance of a substance from the mouth. Clearance will also depend on the flow rate of saliva; it is thus dramatically reduced during sleep (see Chapter 4.2.3 Rate of Flow of Saliva). The clinical importance of clearance lies in the rate of elimination of sucrose and fluorides from the oral cavity. A high rate of salivary clearance in individuals has been associated with a reduction in caries prevalence.
Buffering capacity: Calcium apatite crystals on the enamel surface may become ionized and dissolve into the saliva, if the fluid environment drops below a pH of 5.3. Saliva contains buffers which tend to restore the pH to neutral or at least safer levels of acidity. The primary sources of the buffering capacity of saliva are bicarbonate ions (HCO3−). The products of the acid–bicarbonate reaction are water and carbon dioxide. Phosphates, protein, and urea are buffers of lesser importance. Organisms which are able to metabolize urea may form ammonia (NH3+) as an end product. This will raise the pH and tend to neutralize acids. The buffering capacity of saliva becomes greater as the flow of saliva increases.
Antisolubility: Calcium and phosphate ions in the saliva are supersaturated due to the presence of certain calcium-binding proteins (see Appendix D.1 Saturated Solutions). There is therefore little tendency for calcium ions from the enamel to leave the surface and enter an already saturated environment (▶ Fig. 4.5). The majority of salivary proteins (50–70%) are characterized by the frequency of the amino acid proline in their chain structure. Proline has a particular affinity for binding calcium. This accounts for the ability of PRPs to maintain a supersaturated solution of calcium in saliva. It also accounts for their ability to bind chemically onto the calcium in the hydroxyapatite of enamel during the formation of pellicle. The attachment of PRPs to enamel not only protects it from being dissolved by acids in the mouth, but in addition, it prevents the deposition of apatite crystal onto enamel. Without this function “secondary” crystal deposits would grow onto tooth surfaces. Crystal growth on enamel is not calculus, which is calcified plaque. The PRPs also bind selectively to microorganisms providing a chain which forms a link between organisms and tooth surfaces via the salivary pellicle. The PRPs cannot bind to organisms before they have been absorbed onto the hydroxyapatite of the enamel. Only then is a special sequence of amino acids revealed to which organisms can attach. The PRPs also bind onto some food proteins, in particular tannins, which would be otherwise be toxic.
Fig. 4.5 A diagrammatic representation of the effect of acidity on the degree of saturation of saliva and the dynamics of mineralization and demineralization. Saliva is a saturated solution of calcium and phosphate ions at a normal pH. If the solution becomes acid, the saliva is no longer saturated and hydroxyapatite ions dissolve (demineralize) and enter solution from the crystal. If the solution becomes alkali, saliva becomes saturated and ions precipitate (remineralize) back onto the crystal.
Antimicrobial peptides and proteins: Oral fluid has several components (about 45) which are antibacterial to a varying degree. Some of the proteins such as immunoglobulins (Igs), lactoferrin, and lysozyme are thought to have a role in defense although studies have not shown a correlation between the levels of salivary proteins and dental caries. The smaller peptides on the other hand do appear to have a more predictable defensive action on oral bacteria. These are collectively called antimicrobial peptides (AMP). They include defensins (alpha and beta), cathelicidin, and histatins. All are found in salivary gland and duct cells and in gingival crevicular fluid. Their sources in crevicular fluid are the neutrophils that migrate into the oral cavity. One of the AMPs, alpha defensin was found to be significantly lower in children with caries. This finding may lead to a useful measure of caries risk in children. The defensins and cathelicidins are broadly effective against oral microorganisms including Streptococcus mutans and Porphyromonas gingivalis, but it is difficult to generalize their antibacterial function. It is likely that they act synergistically with other antimicrobials. They also stimulate the acquired immune system and could enhance IgA production.
Lysozyme: Lysozyme is an enzyme present in saliva and tears. Its antibacterial effect is due to the splitting of a bond in the peptide chain of the cell wall of certain gram-positive organisms. The normal flora of the mouth seems to be little affected by lysozyme; it may be more important as a defense against exogenous (“foreign”) organisms.
Peroxidaze: Peroxidaze catalyzes the peroxidation of thiocyanate (SCN) to hypothiocyanite (OSCN) which inhibits bacteria metabolism. Peroxidaze is effective in even low concentrations when the pH of plaque decreases.
Lactoferrin: Lactoferrin is an iron-binding protein which inhibits the metabolism of some bacteria.
Immunoglobulins: A secretory component is added to immunoglobulins in the salivary glands, which activate its potential to neutralize bacteria in the oral cavity. These secretory immunoglobulin A (sIgA) do not operate as humeral antibodies, as they are not in a tissue fluid environment. They therefore cannot make a direct attack on an antigen causing agglutination, precipitation, neutralization, or lysis. Neither they can produce any amplification of the complement or anaphylactic system. Immunoglobulins appear to operate in the following ways:
-
sIgA neutralizes viruses, and toxins, or enzymes produced by microorganisms. For example, sIgA is able to neutralize the enzyme glycosyltransferase which is necessary for S. mutans to synthesis extracellular polysaccharides.
-
sIgA blocks the attachment sites for adhesion of bacteria to the mucosal surface. This blocking occurs in two ways: by covering the specific attachment sites on epithelial cells and by covering the part of the bacterial cell wall which is the active site for attachment. Some species of bacteria are more susceptible than others to the blocking effect of sIgA (▶ Fig. 4.6).
-
sIgA also cause bacteria to clump together (agglutinate) which facilitates clearance.
-
sIgA may bind to the active sites of food antigens thereby reducing the risk of developing an excessive immune response and allergy to certain foods.
Fig. 4.6 Diagrammatic representation of the action of sIgA on salivary organisms. (1) some organisms are unaffected by sIgA and adhere selectively to salivary pellicle, while others (2) adhere to oral mucosal surfaces. IgA causes clumping (3) of susceptible bacteria and blocks sites (4) of adhesion to other organisms. Some bacteria (5) are able to split the IgA dimer.
The defense mechanism of sIgA is not as effective as might be expected. Certain bacteria (Streptococcus, Bacteroides, and Capnocytophaga species) produce proteases which split the dimer of sIgA into two ineffective parts. These IgA proteases do in turn elicit antibody production, but they are so weakly antigenic that the response is not effective. This may explain why Streptococcus sanguis and Streptococcus mitior are able to remain constant members of the oral cavity throughout life. If a patient is treated with immunosuppressive drugs (e.g., used in bone marrow transplants), the secretion of sIgA is reduced, and the oral flora may become invaded with organisms from the gut. The presence of large numbers of gut organisms in the oral cavity causes a mucositis which is difficult to control. This mucositis suggests a wider protective role of sIgA. sIgA may be most effective in controlling the population of exogenous organisms in the mouth (see Chapter 4.7.3 Antigen Tolerance).
4.2.3 Rate of Flow of Saliva
The mouth is bathed by a resting flow of saliva which during the day is a total of about 1 L. This ensures that the mouth and throat are kept moist at all times. During sleep the rate of flow is almost unmeasurable and accounts for the dryness of the mouth on waking. In other circumstance, particularly feeding, the rate of secretion of saliva increases. The following factors are important in the stimulation of saliva which is driven by the activity of both sympathetic and parasympathetic nerve pathways.
-
Taste: Taste is the most potent salivary stimulant. Different modalities vary in their power to stimulate saliva flow. Sour food ranks highest through salt and sweet to bitter tasting foods. One of the functions of the water in saliva is to dissolve and spread food chemicals (see Chapter 10.6 Taste).
-
Mastication: When food is held between the teeth, a little saliva flows from the ipsilateral (same side) glands. As further bite force is exerted, receptors in the periodontal ligament send impulses to the brain and the rate of flow is increased. These events have been described as the masticatory–salivary reflex.
-
Touch: Stimulation of nerve endings in the oral mucosa, particularly by spicy or irritating substances, produces an increase in flow rate of saliva. Hot or cold water also has a stimulating effect. In some areas of the oral cavity, such as the soft palate, even light touch causes an increased flow of saliva. This may be a response to enable swallowing and a protective response to the onset of retching.
-
Sight, smell, and thought: The presence of food, or even the thought of it, increases salivary secretions. Some foods like lemons or onions have a particularly strong effect even at a distance from the mouth.
-
Nausea and vomiting: Copious amounts of saliva are released during vomiting. This may be a protective reflex, protecting the oral cavity from stomach acids which are sufficiently concentrated to damage the oral mucosa and etch tooth enamel.
-
Nutrition: Malnutrition causes irreversible damage to the salivary glands of rats if it occurs during infancy. During later years, malnutrition in human children has been shown to reduce the rate of saliva secretion and the protein content.
-
Drugs: Several drugs which are mood altering (antidepressants and tranquilizers) reduce salivary secretions.
-
Ageing: During ageing all mucosal secretions are reduced, and this may lead, in the oral cavity, to the onset of root surface caries.
-
Radiation: One of the undesirable side effects of radiation treatment for oral tumors is damage to the acinar cells of the salivary glands. The xerostomia (dry mouth) which develops is usually permanent and requires special preventive measures to be put in place in order to prevent caries in patients who have had radiation treatment.
Saliva is an active secretion from both major and minor salivary glands. The three major salivary glands are the parotid, submandibular, and sublingual glands. The composition of saliva varies according to the types of secretory gland (see Appendix D.2 Salivary Gland Secretion).
4.2.4 Gingival Crevicular Fluid
Filter paper inserted into the gingival sulcus soon becomes saturated with crevicular fluid. This fluid derives from the connective tissue of the gingiva, passes through spaces in the junctional epithelium, and enters the gingival sulcus. The rate of flow and composition of the gingival crevicular fluid (GCF) are influenced by the following factors:
-
The fluid flow is minimal in the morning and reaches a maximum at the end of the day.
-
Pregnancy causes the rate of GCF to increase. There is an increase in gingival inflammation represented by a tendency for gingival bleeding during pregnancy.
-
Fluid flow is minimal during the middle of the menstrual period.
-
One of the earliest signs of gingival inflammation is an increase in GCF flow. There is some correlation between the rate of flow and the severity of the gingival inflammation.
The spaces between the cells of the junctional epithelium comprise 18% of its volume. These spaces are large enough to allow the passage of large molecules and cells (see Chapter 3.7 Junctional Epithelium). Thus, bacteria and their toxic products and other antigens may enter the gingival connective tissue through the junctional epithelium. This constant minor invasion causes a mild inflammatory state in the lamina propria of the junctional epithelium. Hence, neutrophils which have migrated into the junctional epithelium in response to the presence of bacterial products are flushed out into the GCF fluid. From this route, 25,000 neutrophils enter the mouth every 15 minutes. Other cells found in the GCF are desquamated epithelial cells.
In health, the GCF is neither exactly like serum or an inflammatory exudate. There are slightly higher concentrations of inorganic ions than in serum and higher levels of carbohydrates. The serum immunoglobulins, IgG, IgM, and IgA, are all found in GCF (not the secretory version of IgA which is found in saliva). Their presence may regulate the entrance of bacteria into the epithelium. One of the functions of antibodies is in triggering the complement cascade of reactions which produce enzymes capable of inflicting damage to the cell walls of bacteria. These enzymes are also capable of damaging host cells, and this may be another reason for the inflammatory state of the lamina propria under the junctional epithelium. The enzymes found in GCF are evidence of the battle between invading bacteria and the defending neutrophils. For example, there is acid phosphatase (from cell breakdown), glucuronidase, lysozyme, hyaluronidase, and collagenases.
Review Questions
4.3 The Biofilms of the Oral Environment
The oral flora is established from birth by organisms which are passed from the mother to the child. Many bacteria in the air and in food also have access to the oral cavity but do not survive. The oral flora is therefore a specific and, in health, a fairly stable bacterial community. There are, even in healthy mouths, huge numbers of microorganisms. Saliva contains 100 million organisms per milliliter, but the gingival sulcus supports 100 billion bacteria per milliliter. This massive population of the gingival sulcus is possible because the oral organisms have adopted a communal existence. They have formed biofilms, in which a variety of species live in a densely packed mass. The oral biofilms depend on the establishment of a symbiotic relationship with their host and a symbiotic relationship between species, in order for their ecosystem to thrive.
4.3.1 Biofilms
Slime is an everyday description of the slippery film, which covers surfaces which are always wet. Slime collects on the inside of water pipes, the bottoms of ships, artificial heart valves, the lining of the gut, rocks, and plants in ponds, rivers, and sea. The word slime tells us that is slippery but not that is made up of living organisms, so biofilm is a more accurate description. Biofilms have a most interesting characteristic, in that the different species of bacteria show levels of interaction, cooperation, and organization not found in their free-swimming or planktonic forms. The collective behavior of organisms creates a microecosystem. Over time, a stable hierarchy and balance between the different species develop. As we have noted in other ecosystems, some organisms, the keystone species, have a disproportionate influence. These organisms may influence the structure of the biofilm, and if it is in a symbiotic relationship with a host, the keystone species determine the biofilms’ relationship with the host.
The deeper layers of a biofilm have a lower concentration of oxygen than the surface layer. These oxygen-depleted layers provide a suitable microenvironment for anaerobic organisms which are unable to survive in the oxygen-rich (aerobic) environment of the oral cavity. The deeper layers of the biofilm would also have lower concentrations of nutrients were it not for channels which are maintained for the transport of nutrients through the mass of organisms.
These channels may be seen in an oral biofilm which has calcified (calculus), a process which mineralizes the soft matrix and preserves its architecture (▶ Fig. 4.7). The gradients of substrate concentration, oxygen availability, and pH in the complex structure of the biofilm provide a wide variety of habitats for microorganisms. The structural organization and functional coordination of organisms in a biofilm and the specialized role of some species, which orchestrate the behavior of other species bring biofilms close to the realms of a single organism.
Fig. 4.7 A SEM image (magnification × 300) of calcified plaque (calculus) which was etched to remove all the organic material. The remaining calcified material reflects the structural organization of the living biofilm just as a piece of dried coral is a relic of the ecosystem of the living reef. The insert (magnification × 1000) reveals laminations and channels which the organisms have constructed to define a variety of habitats within the plaque biofilm.
The external environment has at first a decisive influence on the early colonizing species of a biofilm. As the biofilm matures, the cooperative influence of the early colonizers creates an inner structural and functional organization. The biofilm begins to create its own inner environment which supports organisms that would not otherwise survive in the external environment. This dynamic, in which organisms create a purpose-defined environment for the benefit of their own and other species, requires a review of accepted evolutionary theory. This orthodox view places the organism within an environment to which it must adapt or die. The restriction has not provided for the organism to create its own environment by cooperation with other species. Cross-talk between organisms, now known as quorum sensing, would have been ridiculed just 50 years ago. It is about 50 years ago an idea, suggested by James Lovelock, that the biosphere regulated itself was also ridiculed. The theory was unfortunately given a mystic name Gaia (earth mother), but it was supported by serious scientists. The more that is understood of the structural and organizational microenvironments created by biofilms, the more likely it becomes that the Gaia theory of biosphere self-regulation is a manifestation of an upscaled version of the self-organization found in biofilms (see Appendix D.3 Gaia Theory).
4.3.2 Calculus
Saliva is saturated with calcium and phosphate ions which may precipitate in an alkaline environment. If the precipitate occurs within plaque, it forms a firm, chalky deposit called calculus (Fig. 4.7). Plaque may become alkaline due to the activity of some oral bacteria which release ammonia during their metabolism of salivary urea. Calculus which is supragingival is generally white and chalky, whereas calculus which is subgingival is darker and harder. The precipitated calcium is either soft brushite (supragingival) or harder whitlockite (subgingival, perhaps from calcium in the crevicular fluid). The submandibular gland saliva has a higher concentration of calcium than other glands and has a relatively high pH. This may explain the rapid accumulation of calculus on the lingual surfaces of the lower incisors. Deposits of calculus have been implicated as a cause of gingivitis and periodontitis. Removal of calculus by prophylaxis has long been thought to help prevent periodontal disease although recent evidence challenges this assumption.
4.3.3 Growth of a Biofilm
Growth of a biofilm occurs in three distinct phases (▶ Fig. 4.8).
Adherence to a substrate is the first phase. Generally, hydrophilic (water-loving) surfaces encourage bacterial attachment and hydrophobic surfaces encourage their detachment. Many species of oral organisms produce biosurfactants, which decrease the surface tension of the substrate surface, causing it to become hydrophilic. These biosurfactants are of universal benefit to all species of adhering organism, so the production of them has communal value. The attachments made by organisms onto oral surfaces are either by specific protein adherent sites (ligands) on the bacterial cell membrane or nonspecific polysaccharide attachments via fibrils. Of these two forms of attachment, the fibrillar attachments, though less specific, are stronger than the rather weak but specific ligand connections.
Aggregation is the second phase of growth in which organisms clump together to form a complete layer. Aggregation may involve the adhesion of different species to each other. Two species which adhere to each other are P. gingivalis and Streptococcus gordonii. They also have significant levels of intercommunication which share changes in gene expression which prepare for a community existence in the biofilm. The partnership of these two species may become significant in orchestrating the early stages of gingivitis and periodontal disease.
The third phase of biofilm growth is the rapid increase in number of organisms. The concentration of organisms in a biofilm is likely to be 10,000 times greater than their concentration in planktonic form. This shift between a solitary planktonic existence, and a dense biofilm, requires some major adaptations of the bacteria which are achieved by gene regulation. This gene regulation is triggered by chemical messengers from other organisms, an example of quorum sensing (see Appendix D.4 Gene Regulation in Biofilms).
The benefits of existence in a biofilm as distinct from a floating (planktonic) existence in water are significant. The biofilm provides shelter, accommodation, food sharing, stability, and continuity. Planktonic bacteria are vulnerable to quite low concentrations of toxic chemicals (disinfectants). Bacteria in a biofilm are protected by the outer layers of organisms and very resistant to even high levels of disinfectant. The biofilm is only reliably disrupted by mechanically scraping or brushing it of the surface it is attached to (see Appendix D.5 Strategies for Controlling Biofilms).
4.3.4 Dental Plaque
Dental plaque is a biofilm which forms on oral surfaces. It is formed by a variety of oral organisms which form an aggregate within an adhesive matrix of salivary and bacterial polymers.
If a tooth surface is thoroughly cleaned, a layer of salivary pellicle forms within hours. Individual bacteria may soon be found attached to the pellicle (▶ Fig. 4.9). The early colonizers are mostly cocci and small rods which stain gram-positive bacteria (See Appendix D.6 Gram Staining Bacteria). Later colonizers are filamentous organisms which form a meshwork and may themselves become the site of attachment for smaller organisms. Adhesion to each other may improve the chances of survival where there is competition for surfaces. When viewed with a scanning electron microscope (SEM), these aggregates of organisms were described by Listgarten as “corn cobs.” A similar arrangement has occurred between some rod-shaped organisms and filament-shaped bacteria which has already attached to the pellicle. These aggregates have been called “bottle brushes.” The attachment of the filamentous organisms to the pellicle is by specific ligands, whereas the interbacterial attachments are by fimbriae. It is not clear what the relationship is between the two species of organisms in these aggregates, but it is likely that there is mutual benefit (▶ Fig. 4.10).
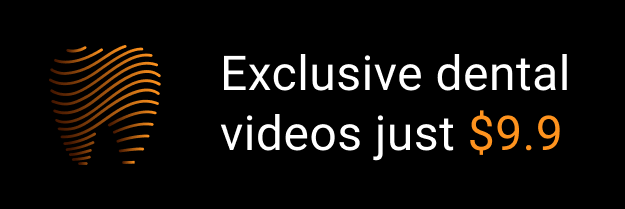