This chapter covers a wide range of topics in order to link the structure of a tooth with its vulnerability to bacteria and their products. The hard tissues of teeth are not merely passive participants in their destruction. There is a constant ebb and flow of demineralization which is dependent on macroscopic conditions, such as the diet and level of oral hygiene, but equally on the microscopic environment of the tooth such as the presence of pits and fissures in enamel. Some demineralization of dentin is prevented by the defense barriers set up by living cells of the pulp–dentin. A knowledge of the natural defense capacity of enamel and dentin to resist destruction by bacterial products allows us to rethink orthodox restorative practices. The use of low-intervention clinical procedures has arisen through awareness of the capacity of the dental hard tissues to resist destruction and repair. The search for minimally invasive procedures must continue while too many teeth are not saved by dentistry but are caught up in a downward spiral of treatment and retreatment until they are eventually beyond further repair.
2.1 Enamel: Clinical Aspects
2.1.1 Enamel Minerals
Enamel is about 95% mineral by weight but 87% by volume. Most of this mineral is hydroxyapatite, but there are other apatites and other minerals (magnesium and carbonates), so the mineral phase of enamel is not pure hydroxyapatite. The less mineralized the enamel is, the whiter and more opaque it is; the more mineralized, the more translucent enamel allows the yellow color of the underlying dentin to show through. Examples of white, poorly mineralized enamel are the enamel of deciduous teeth and chalky patches of fluorosis and early carious lesions in adult teeth. Enamel crystals are about 10 times thicker and much longer than the apatite crystals of bone or dentin; the volume is about 1,000 times greater. The surface area is therefore relatively low, and this factor makes enamel crystal less reactive and soluble to acids than are apatite crystals of bone or dentin. The crystal also contains very little of the more soluble carbonates and magnesium salts found in dentin and bone. The enamel crystals are highly orientated and tightly packed. These factors account in part for the greater hardness of enamel in comparison to dentin and also for its resistance to acid demineralization. In addition, enamel has a lower percentage of organic material than dentin (see Appendix BTable B.1).
Enamel crystals are packed into larger units, the enamel prism, which is the structure made by each ameloblast as it lays down enamel during development. The prisms are about 100 crystals wide but are very long and may run uninterrupted from the dentin to the tooth surface. Both crystals and prisms have their long axis parallel to each other and are directed toward the surface of the tooth. However, at the prism boundaries, the crystals tend to turn outward and face their neighboring crystals. In this interprismatic zone, the enamel crystal orientation is slightly less ordered, and there is a little more space between them (▶ Fig. 2.1). This makes the interprismatic regions slightly weaker and more easily disrupted by tooth wear. During wear, whole prisms break off leaving a sharp edge to the enamel which is needed to shred food (▶ Fig. 2.2). During preparation of a cavity for a composite or amalgam restoration, the margins of enamel must be prepared so that all the enamel prisms are supported. This prevents their chipping off later and causing a failure at the margins of the restoration (▶ Fig. 2.3) (see Appendix B.1 Physical Properties of Enamel and Dentin).
Fig. 2.1 A diagrammatic representation of the formation of enamel prisms during tooth development. Ameloblasts secrete enamel prisms parallel to each other with the enamel crystals orientated in the same direction. In the interprismatic zone, the crystals turn outward. The enamel matrix is secreted and mineralized by the ameloblast (red arrow), and the protein then resorbed (blue arrow).
Fig. 2.2 A magnified view of a fractured enamel surface reveals parallel prisms packed together. Scanning electron microscope (SEM) image (magnification × 300) of an enamel surface, prepared by fracture in the direction of the enamel prisms. The fractured surface reveals enamel prisms stacked like the grain in a log of wood. The surface of the tooth was etched to reveal the ends of the enamel prisms.
Fig. 2.3 Diagrammatic representation of the orientation of enamel prisms of a tooth illustrating the importance of avoiding leaving undermined enamel prisms during cavity preparation. (a) During cavity preparation it is important to ensure that angle of the cavity wall is prepared along the axis of enamel prisms. (b) Undermined margins leave fragments of prisms behind, which may fracture away from the cavity wall leaving a defect between the restoration of the tooth surface.
2.1.2 Enamel: Non-Minerals
The non-mineral phase of enamel consists of proteins and water. This phase is not mixed randomly within the mineral but surrounds the large mineral crystals which are packed tightly together. The minute spaces in between the enamel crystals account for the slight porosity of enamel. During tooth development, the first formed enamel is very poorly mineralized. It appears chalk like and is dissolved readily by acids. As the newly formed enamel matures, proteins are removed and the enamel becomes more resistant to dissolution by acids (see Appendix B.2 Enamel Proteins).
2.1.3 Enamel Etching
The disorientation of crystals in the interprismatic zone makes it more susceptible to acid demineralization than the well-orientated crystals of prismatic enamel. It is the interprismatic zone which first demineralizes due to early caries. However, the dissolution pattern varies when organic acids are used to etch enamel. Etching enamel is a routine procedure when using restorative resins, because the resin bonds to a roughened surface better than to a smooth one. The two common etching patterns are etching of the prism center and etching of the interprismatic substance (▶ Fig. 2.4).
Fig. 2.4 The effect of acid etching an enamel surface. An SEM image (magnification × 1,000) of enamel surface after acid etching reveals that the interprismatic enamel has dissolved more rapidly than the prism core leaving a raise projection. In other areas, the core of the enamel prisms has dissolved more rapidly leaving a pitted surface, which provides mechanical retention for adhesive resins such as fissure sealants.
2.1.4 Early Enamel Caries
Plaque is an aggregate of bacteria in a sticky matrix which forms on the surface of enamel. Acid which has been formed as a product of bacterial metabolism may dissolve the surface enamel. If the surface breaks down, it may form a visible defect in the enamel which under normal light appears as a white patch. Early enamel caries seen under polarized light reveals four distinct zones of mineralization (▶ Fig. 2.5). The outer surface zone is well mineralized by replacement ions from plaque and saliva. However, the body of the lesion is poorly mineralized. Deeper to the body of the lesion, a darker zone represents some remineralization, while the deepest zone is yet again demineralized. These zones of demineralization and remineralization illustrate the dynamic series of events which are occurring in the early lesion. Caries is not simply a process of continued demineralization.
Fig. 2.5 A diagrammatic representation of the layers which can be defined in an early caries lesion which illustrate the dynamic nature of demineralization and remineralization. The early enamel lesion consists of four zones of alternating levels of mineralization. The surface zone (SZ) is remineralized but blocks the passage of calcium ions into the body of the lesion (B) which remains demineralized. It may have to be removed to allow the lesion to become arrested. The dark zone (DZ) is well mineralized, but the translucent zone (TZ) is yet again demineralized. (Adapted from Kidd and Joyston-Bechal 1987.)
2.1.5 Arrested Enamel Caries
The early lesion in enamel may be reversed and remineralized if plaque is removed. Ion exchange on the surface of enamel is a dynamic process which is occurring all the time. It is influenced by the degree of saturation of calcium and phosphate salts in plaque and saliva and the level of fluoride in the enamel and the adjacent plaque fluid. If the fluid surrounding the early lesion is highly saturated, crystals of apatite may form on the outer surface zone and remineralize the enamel of the early lesion. However, minerals in saliva may also block further penetration of calcium ions into the deeper layers of the lesion. If remineralization takes place slowly, and in the presence of fluoride, extensive mineralization occurs throughout the lesion.
The major contribution of fluoride in caries prevention appears to be in the process of arresting early lesions and preventing their progression into irreversible enamel loss and the formation of a cavity. Fluoride is concentrated in arrested enamel lesions and at the margins of actual enamel cavities. This may be due to the breakdown of the enamel surface at the center of an early lesion and the subsequent massive loss of mineral in the center, leaving the margins relatively intact with high fluoride levels.
Arrested enamel lesions are often seen on an approximal surface some time after an adjacent tooth has been extracted. Simply by improving the self-cleansing of the approximal area, the extraction of a neighboring tooth helps to reduce plaque mass. The lesion is recognizable by a brown stain, sometimes surrounded by a halo of chalky white enamel. Fluoride accumulates in remineralizing enamel, making the enamel more resistant to subsequent acid attack. So, an arrested enamel lesion is more resistant to acid demineralization than it was originally, as each cycle of demineralization leaves the enamel stronger than it was before (▶ Fig. 2.6).
Fig. 2.6 SEM images of enamel reveal remineralization of early caries. A SEM (magnification × 5,000s) of etched enamel surface from an area recognized clinically as arrested caries. (a) In the center of the lesion, there are clusters of remineralizations (R) which resisted acid etching during preparation of the specimen. (b) Toward the periphery of the lesion, the enamel has become well remineralized and the lesion has become arrested (A).
Sometimes, the lesion progresses in spite of the availability of calcium and fluoride ions (▶ Fig. 2.7). This may be due to the presence of the barrier in the superficial zone, which we have noted above, restricts the movement of calcium and fluoride ions into the demineralized zone. This barrier may be due to plugging of the spaces between enamel crystals with salivary proteins.
Replacement minerals cannot get to the damaged site, but if the enamel surface is treated with a deproteinizing agent, remineralization occurs more readily. Remineralization may also be achieved by the removal of this superficial zone of the early lesion. This of course is more destructive than deproteinizing the surface, but there is evidence that tooth wear may be a significant process in arresting enamel lesions. If white lesions return to normal-looking enamel, either after orthodontic band removal or acid etching, it appears to be due to abrasion of the weakened enamel crystals by food and brushing. If remineralization does not occur as fast as the demineralization of enamel, eventually normal enamel structure is lost and a cavity develops.
Calcium hydroxyapatite ions are dissolving from and precipitating onto the enamel surface all the time. By reducing the acidity of the fluid surrounding the enamel surface, the balance toward mineralization instead of demineralization may be sustained. Even established lesions of demineralization may be reversed by fluoride treatment and careful oral hygiene.
2.2 Pulp–Dentin: Clinical Aspects
The pulp–dentin is a highly vascular loose connective tissue surrounded by a component of calcified connective tissue, the dentin. Although there are very obvious structural differences between pulp and dentin, the following reasons suggest they be treated as an integrated complex.
-
During tooth development dentin is formed by odontoblasts of the pulp. After tooth development, peritubular and secondary dentin continue to be formed by the pulp odontoblast.
-
The sensitivity of dentin depends on the presence of odontoblasts.
-
The capacity of dentin to respond to irritation such as tooth wear, or dental caries, depends on odontoblasts.
-
Both sensitivity and response to irritation are directly affected by the state of the entire pulp tissue.
-
From an operative point of view, any procedure involving dentin is a procedure involving the pulp; any material or medication placed against dentin is a material or medication placed against the pulp.
2.2.1 Physical Properties
Dentin consists of mainly a collagenous matrix, mineralized to a moderate degree with a variety of apatite salts and magnesium and carbonates. By weight, it consists of 72% mineral, 18% organic matrix, and 10% water. It is less brittle than enamel (the modulus is about one-tenth of enamel), but is softer (about one-fifth of the hardness) and so wears more rapidly (see Appendix B.1 Physical Properties of Enamel and Dentin). The physical properties of dentin contribute to two important mechanical functions of the tooth. The first is to provide resilience to fracture. This resilience is due to the presence of proteoglycans. These molecules are resilient and prevent the propagation of cracks. Resilience is also provided by the collagen fibers which are orientated at right angles to a crack which may form in adjacent enamel (see Appendix B.3 Composites and Resistance to Fracture). The second important mechanical property of dentin is to provide a composite tooth surface for efficient mastication. Under the influence of a course diet the dentin wears faster than the enamel, leading to the formation of a concave grinding surface surrounded by a sharp cutting edge (see Chapter 1 The Origins of Teeth).
2.2.2 Tubules
Dentin is laid down by odontoblasts during tooth development. As the odontoblast retreats away from the secreted dentin, it leaves behind a process of the cell which remains into adult life, inside a fine tube or tubule. There is a greater density of tubules in cervical and root dentin than in coronal dentin (▶ Fig. 2.8 and ▶ Fig. 2.9).
Fig. 2.8 A SEM image (magnification × 300, window × 1,500) of dentin tubules viewed from the coronal pulp. The odontoblasts and their processes have been removed. Note the high density and large diameter of the tubules, which would make this area of the pulp–dentin highly permeability to fluid movement.
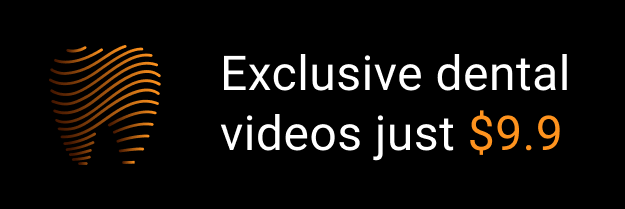