Chapter 8
Fluid Dynamics of Irrigation within the Root Canal System
Franklin R. Tay
Department of Endodontics, Georgia Regents University, Augusta, GA, USA
Introduction
The time-honored endodontic triad of debridement, disinfection, and obturation has been recognized for over a century (1). However, our discovery of the strategies for achieving these objectives has not followed a linear logic path, but has been sidetracked for decades by misunderstood scientific observations. Hunter’s focal infection theory (2) was supported by the voluminous, yet specious, work of Price (3), but was challenged by clinicians like Grossman in the 1930s (4) who cultured materials taken from the root canals in a crude culturing incubator fabricated from nothing more than a lunch box and a light bulb. The culturing era basically ended in 1964 when Bender and Seltzer (5) published their seminal paper: “To culture or not to culture?” As it turned out, their work also was based on misunderstood and inaccurate scientific observations. Forty-two years later, Spånberg (6) criticized in an editorial that this paper began a 10-year “walk in the desert” until Byström and Sundqvist (7) reestablished the importance of microorganisms in apical infections. Interestingly, Spånberg’s editorial was written at about the same time as the discovery of endodontic biofilms. These biofilms were subsequently identified as the causative agents for apical periodontitis (8).
A thorough review of the fluid mechanics of root canal irrigation has already been performed by Gulabivala et al. (9). Readers are encouraged to read this excellent work. It cannot be denied that the subject of endodontic fluid dynamics is also wrought with myths, misunderstood observations, prejudices, biases, or often a simple lack of understanding. Accordingly, it is necessary to identify and explain these misconceptions, via evidence-based studies, that have unwittingly been embedded into this subject. These include (i) apical vapor lock effect, (ii) hydrolysis of organic components of the soft and hard pulp tissues and canal wall biofilms, (iii) technique misunderstandings, and (iv) patency safety issues. Following these demystifications, evidence-based studies should be used to identify strategies for attaining safe and effective intra- and extracanal irrigant fluid dynamics.
Misconceptions
Apical vapor lock (AVL)
The AVL is a physical phenomenon wherein CO2 and NH3 arising from the hydrolysis of organic tissues mix and form a gas bubble in the apical portion of the root canal. This bubble cannot escape into the apical tissue if the apex is oriented in the upward position. When the apex is orientated downward, the gas bubble is trapped by the weight of the irrigant and its surface tension force resists the buoyancy force of the trapped gas (Figure 8.1). Furthermore, any attempt to “pop” the bubble in the latter orientation is counterproductive as explained in Figure 8.2. In 1983, Chow (10) observed air bubbles or columns in-vitro that could not be displaced or passed around except by placing a small needle very close to the apex without binding, a practice that would later prove to be potentially dangerous (11). Recent findings have discovered that the interfacial force equilibrium at the dentin, gas, fluid interface can be disrupted only by the apical negative pressure or effective microstreaming (12).
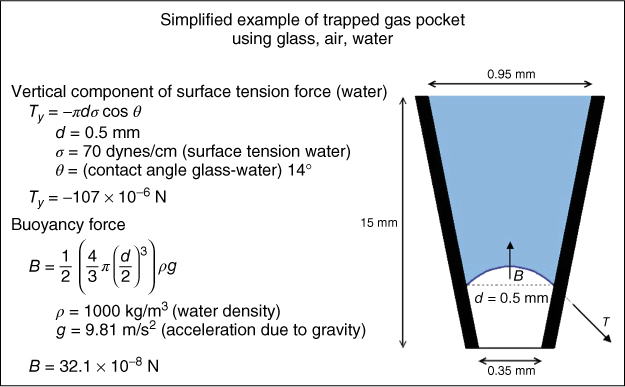
Figure 8.1 To explain how a gas pocket can be trapped under a fluid column, a simplified glass tube shaped as a 4% tapering cone with an apical diameter of 0.35 mm is filled with water, leaving a gas pocket at the bottom. The gas buoyancy acts vertically upward and will act only on the part of the pocket that is immersed in liquid, which is shown as a dotted line. The buoyancy force (B) is determined by the size of the immersed part that is determined by the shape of the meniscus, which can be calculated by the Young–Laplace equation. For simplicity, if the shape is considered to be spherical and of the diameter equal to that of the cone at that point, then B will be about 300 times smaller than the vertical component of the surface tension force (Ty). This means that the bubble is trapped. The surface tension force and the weight of the liquid are supported by the increase in pressure of the air pocket.
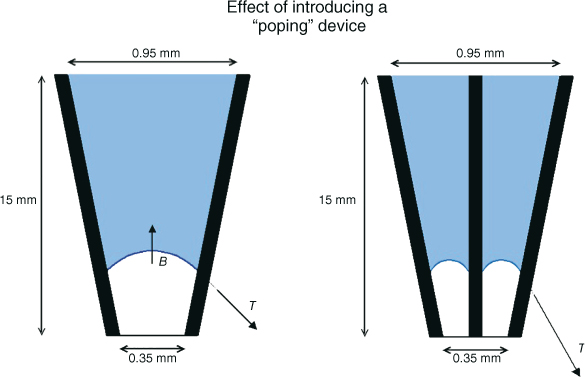
Figure 8.2 Elimination of the vapor lock via “popping” of the pocket is not an option, as inserting any object, such as a gutta-percha point, file, or needle into the hypothetical root canal space will only make the space smaller, with the effect of increasing the surface tension. In fact, when attempting to pop the bubble, the diameter of bubble ring that is formed is decreased and can be half of the initial diameter of the glass tube. The resulting surface tension to buoyancy ratio then can be roughly an entire order of magnitude higher, yielding a ratio of approximately 3000 to 1.
History of discovery
The history of the discovery of AVL is important, because as recently as 2012, investigators were still failing to consider the AVL effect (13) during a protocol design involving endodontic irrigation systems and techniques believed to dissolve organic material in inaccessible areas. Although the use of sodium hypochlorite (NaOCl) as a tissue solvent had been documented as early as 1967 (14), it was not recognized widely until 1974 when Schilder (15) reported the use of an average of 39 ml of NaOCl per visit, combined with patency filing, to debride the instrumented root canal space. Schilder’s irrigation protocol became the benchmark of excellent clinical treatment. Subjective radiographic inspection of his cases seemed to validate that intracanal irregularities and accessory canals were in fact cleaned and obturated, an observation that was proven inaccurate 43 years later (16).
In 1971, Senia et al. (17) authored a classic paper in which he tested the efficacy of 5.25% NaOCl to dissolve intracanal pulp tissue in the mesial roots of lower molars. Briefly, the authors instrumented the mesial roots of lower molars to a size 30 instrument. Then, “the apex was sealed with green stick compound in order to retain the irrigating solutions within the canals, and the root was mounted upright on a glass slide.” Final irrigation was accomplished by applying 5.25% NaOCl and stirring with a size 10 file for either 15 or 30 min. On histological examination, significant residual pulp tissue was found throughout the apical termination and the study concluded that “the value of sodium hypochlorite as an irrigating agent for dissolving pulp tissue in the apical 3 mm of narrow root canals is questionable.”
Six years later, Salzgeber and Brilliant (18) conducted a clinical study to determine the flow characteristics of root canal irrigants in mandibular molars. The authors slightly altered the final shaping of the canal as described by Senia et al. (17), by enlarging the apical termination to a size 35 instrument. Also, they used a radiopaque dye (Hypaque) instead of NaOCl. This study observed that in spite of an extremely cautious irrigant delivery technique during treatment, Hypaque always reached the apical termination of the root canal, but also flowed into the apical tissue of infected teeth (Figure 8.3). The authors concluded that “the solution is not confined to the instrumented space in cases with necrotic pulps and may occupy random dimensions when extruded into the apical lesion. Salzgeber summarized that “The study by Senia et al. (17) showed that little or no sodium hypochlorite reached the apical 3 mm when the root canals were enlarged to a size 30 instrument. The current study showed that the irrigant reaches the apex when the canals are opened larger than a size 30 file.” The flaw with the Salzgeber and Brilliant study was the use of Hypaque instead of NaOCl, because Hypaque is biologically inert while NaOCl hydrolyzes organic materials, releasing NH3 and CO2, and in a closed root canal system forms the AVL.
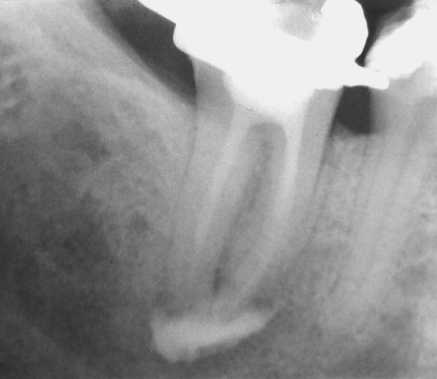
Figure 8.3 Apical radiograph of tooth 30 demonstrating excessive apical extrusion of Hypaque, a radiopaque contrasting medium, into the periapex, despite meticulous care in needle placement and clinical delivery, to ensure the delivery needle did not bind in the canal. Being biologically inert, Hypaque neither hydrolyzes organic material nor produces any gas.
(Salzgeber and Brilliant (18). Reproduced with permission of Elsevier.)
It would be impractical to review the list of irrigation-related articles that were published between the publications of Salzgeber and Brilliant (18) and O’Connell et al. (19), as investigators examined instrumentation/irrigation regimes, different irrigants and their concentrations, as well as time and temperature of exposure. However, the O’Connell article epitomized the furthest advancement in canal system preparation and irrigation techniques in that time period. Importantly, the authors adopted the “Baumgartner protocol” (20) of sealing the apical foramen during instrumentation and irrigation. They used NiTi rotary instrumentation to open the apical foramen to a size 40 at a 4% taper, while irrigating with copious quantities of 5.25% NaOCl and 15% ethylenediaminetetraaceticacid (EDTA). Even with such canal enlargement and the use of dual irrigants to dissolve the organic and inorganic components of the canal wall smear layer, the authors reported that canal walls along the apical 1/3 of the root canals were not clean and the dentinal tubules were covered with the smear layer. However, 3 years later, Torabinejad et al. (21) reported extremely clean walls and open dentinal tubules in the apical 1/3 of the canal space, even though the apical foramen of the root canals were instrumented to a size 30 instrument, the same size as Senia et al. (17) had used 32 years earlier. In the work by Torabinejad et al. (21), the most favorable results were achieved when the authors used a combination of MTAD (an antimicrobial irrigant containing doxycycline, citric acid, and a surfactant) and a low concentration of NaOCl.
When these findings were published, Schoeffel was developing the EndoVac system of apical negative pressure for safety purposes. As the protocol by Torabinejad et al. (21) called for lower and safer concentration of NaOCl, Schoeffel tested the commercially available BioPure® MTAD in early 2005 (Figure 8.4a,b), using the standard “Baumgartner protocol” to seal the apical foramen. It was readily apparent that clean dentinal tubules could not be realized in the apical 1/3 of the canal space by using BioPure MTAD. This was in stark contrast to the results obtained when a root canal with a sealed apex was irrigated with apical negative pressure (Figure 8.4c,d). Careful rereading of the Torabinejad et al. (21) paper revealed the following: “Opening the apical foramen of each tooth to a size 30 file and free flow of irrigant through the apical end of the root canal may have allowed more cleaning of the apical portions of root canals in this experiment.” Schoeffel immediately realized that Senia et al. (17) had inadvertently created the conditions necessary to form an AVL when the authors mounted the roots in greenstick compound. Salzgeber and Brilliant (18) could never have formed an AVL with the use of a biologically inactive irrigant. Torabinejad et al. (21) had a vague idea that a flaw existed in their protocol, because the authors further elaborated: “Studies are in progress to determine the efficacy of various other techniques to carry MTAD into the apical portion of the root canal systems with closed apexes.” Schoeffel’s theory was first publicized in 2008 (22); was validated in-vitro in 2010 (23) and in-vivo in 2012 (24). The AVL is apparent in-vivo (Figure 8.5) and can be broken by either acoustic microstreaming, apical negative pressure, or by placing the irrigation needle at or extremely close to full working length (10). The last method produces very high apical extrusion pressures under normal clinical delivery rates (11), which is addressed in the safety section.
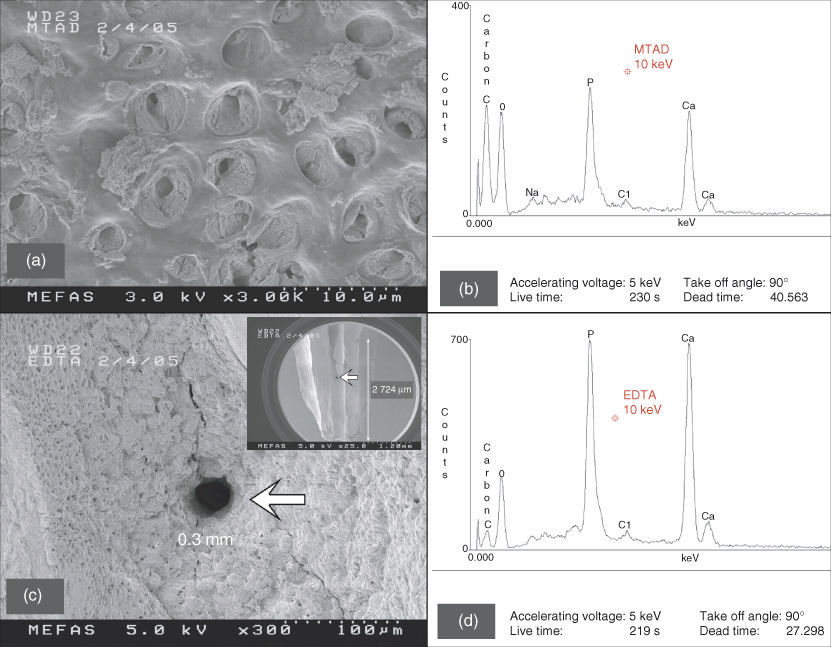
Figure 8.4 These two scanning electron microscopy (SEM) images were derived from noncarious teeth during the early stages of research and development of the EndoVac apical negative pressure irrigation system. (a) A representative SEM image of the dentin wall after treatment using BioPure MTAD according to manufacturer’s instructions. The area is taken from the coronal portion of the apical 1/3 of the canal space where there is an abundance of dentinal tubules. The material in the tubules is undissolved pulpal debris. (b) Energy-dispersive spectroscopy (EDS) spectrum of the area shown in (a) that displays a wealth of carbon. The Ca, O, and P signals originate from hydroxyapatite. (c) A representative SEM image of the canal wall from the apical 1–3 mm of the canal space that was irrigated using apical negative pressure. A clean lateral canal (arrow), 0.3 mm in diameter, can be seen and is located at 2 mm from working length (Inset: arrow indicates the position of the lateral canal). (d) EDS spectrum of the area shown in (c) that reveals a very low carbon level, probably associated with collagen and noncollagenous proteins derived from the mineralized radicular dentin.
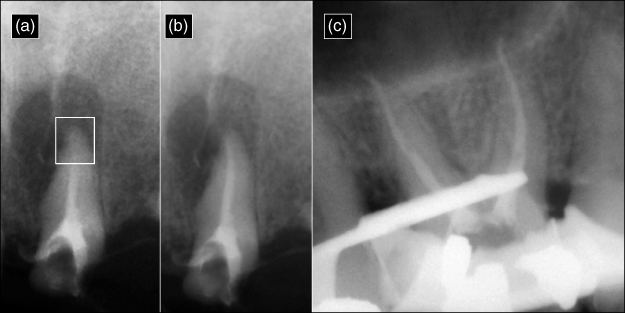
Figure 8.5 The radiopaque appearance in each radiograph is derived from a 1:1 mixture of omnipaque (Iohexol—an angiocardiography contrasting medium) and NaOCl. Because the radiopaque omnipaque is mixed with NaOCl, the exact location of the irrigant mixture can be determined. (a) A vapor lock (box) is present in the apical third of the canal space. (b) The irrigant flowed successfully to the apical termination after ultrasonic activation. (c) The mesial and distal roots of a maxillary molar after using the EndoVac microcannula to deliver the irrigant to full working length. The premolar was isolated with a rubber dam, cyanoacrylate, and a blockout resin.
(Radiographs courtesy of Dr. Jorge Vera, Puebla, Mexico.)
The significance of the AVL is that several studies have erroneously assumed that an irrigant is present throughout all phases of canal preparation and irrigation, thus leading to inaccurate observations. For example, Zehnder (25) demonstrated that two liquids (coronal and apical) can be mixed once the canal is instrumented to size 40; however, the apical liquid would be a gas and would not mix. Boutsioukis et al. (26) assumed that a canal was completely filled with irrigant, without giving considerations to the existence of the AVL, when the authors designed their computational fluid dynamic model. Vera et al. (27) recognized the vexing problem associated with the AVL in root canal irrigation: “Factors such as complex root canal anatomy and the vapor lock phenomenon have been shown to limit the penetration of irrigating solutions into the apical third in both in-vivo and in-vitro studies involving small and wide canals.” Jiang et al. (13) mixed dentin chips with 2% NaOCl for 5 min before placing the mixture in an apical canal groove model, mistakenly assuming that the groove was devoid of organic material (Figure 8.6a).
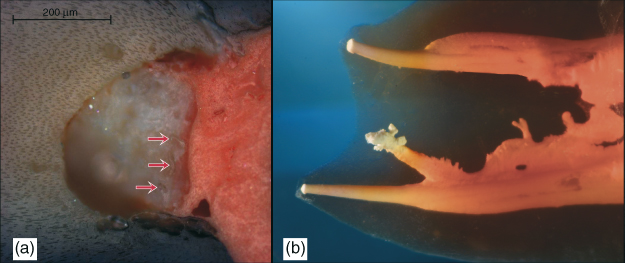
Figure 8.6 (a) Cross-section of a root canal at 5 mm coronal to the working length showing inaccessible amorphous organic mass with dentin shavings (red arrows) apparent at instrumentation interface. (De-Deus et al. (28). Reproduced with permission of Elsevier.) (b) Complex canal anatomy demonstrates several areas where biofilm may flourish in inaccessible areas, including the smallest fins, cul-de-sacs, and isthmus areas and the lateral canals that provide the portal to the apical area. Accordingly, debridement and disinfection via complete hydrolysis throughout the root canal system is essential to prevent biofilm recolonization, possibly from infected tubules.
(Dr. G. John Schoeffel, Dana Point, California. Reproduced with permission of G.J. Schoeffel.)
Dead water space or stagnation plane
As a matter of historical importance, prior to the in-vitro proof regarding the existence of the AVL (23), a similar fluid dynamics problem was referred to as the Dead Water Zone (29), whereby irrigants are effectively exchanged only a short distance in front of the irrigation needle even though the entire root canal is completely filled with the irrigant. This phenomenon was first described by Chow (10) in 1983, who found that irrigation was strictly a function of the depth of the needle. In 2010, Gulabivala et al. (9) referred to the “Dead Water Zone” as the stagnation plane and devoted a comprehensive research paper attempting to identify a mechanism to move the stagnation plane apically to be coincident with the canal terminus, without extruding beyond it. After a thorough investigation of needle designs, flow rates, and passive ultrasonic techniques, Gulabivala et al. concluded that “despite the significant advances in root canal treatment, there remains a lack of clarity about the mechanisms of irrigant delivery, replenishment, mixing, flushing, and wall erosion.” It is important to realize that Gulabivala et al. (9) submitted their paper on June 17, 2009, almost a year before the work by Tay et al. (23) Regardless of AVL, dead water space, or stagnation plane, the resulting problem turned out to be the same always—lack of adequate irrigant exchange in the apical termination to effectively hydrolyze organic debris.
Hydrolysis of pulpal soft tissues and organic components of hard tissue debris
The subject of this chapter is fluid dynamics of irrigation within the root canal system. For effective debridement, there must be an exchange of irrigants throughout the root canal system including canal fins, cul-de-sacs, and isthmus areas. Thus, irrigants must be bioreactive chemicals in order to effectively dissolve, via hydrolysis, the variety of organic molecules encountered in the root canal system. It is interesting to note that sterilization is not part of the endodontic triad because that would require total eradication of every microbe in every tubule. In 1995, Peters et al. (30) reviewed the fate of bacteria in the dentinal tubules and concluded that “there is no evidence, however, that special measures should be taken to kill the bacteria in the dentinal tubules. Should time permit, a sound obturation technique immediately following the cleaning, shaping, and disinfection phases allows the remaining bacteria in the tubules to be either inactivated or prevented from repopulating the (former) canal space. In the vast majority of cases, those bacteria appear not to jeopardize the successful outcome of root canal treatment.” Figure 8.6b exemplifies the concept proposed by Peters et al., wherein the very complex root canal system is fully obturated after successful chemical debridement via hydrolysis. Three-dimensional obturation of the root canal system deprives the space for biofilm to form. It should be further noted that complete obturation of the canal space is impossible if residual dead biofilm remains. This would leave behind spaces for repopulation by other biofilm colonies.
Irrigant concentration and exchange
Issues of irrigant type, concentration, activation, and delivery time must be considered. If one considers a biofilm simply as an organic material (similarly to pulp tissues) that must be hydrolyzed into gas, the disinfection problem then becomes one of simple debridement via hydrolysis (Figure 8.7). Hydrolysis via NaOCl is a simple equilibrium reaction, as demonstrated by the reaction of NaOCl with lysine: 18ClO− + C6H14N2O2 ⇒ 18Cl− + 8H2O + 2NH3 +6CO2. As reported by Hand et al. (31), the speed of the dissolution of the organic material is a function of NaOCl concentration. These authors demonstrated that 0.5% NaOCl dissolved approximately 55,000% less necrotic tissues per unit time than 5.25% NaOCl. Although chlorhexidine has also been advocated as an antimicrobial irrigant, its hydrolytic effect on oral biofilms is not as pronounced as NaOCl (Figure 8.7). Clegg et al. (32) examined several contemporary irrigants against oral biofilms using both scanning electron microscopy (SEM) and culturing methods (Figure 8.8). Their results are in agreement with what is shown in Figure 8.7, in that chlorhexidine has minimal effect in destroying biofilms. Likewise, Siqueira et al. (33) was unable to achieve 100% negative culture when chlorhexidine was employed as an irrigant clinically. Ercan et al. compared the antibacterial activity of chlorhexidine versus NaOCl (34) and concluded that “although it [NaOCl] is an effective antimicrobial agent and has excellent solvent properties for vital, necrotic, and fixed tissues, it is known to be highly irritating for apical tissues, especially at high concentrations. Because of the adverse effects of this irrigant, researchers have developed alternative irrigants.”
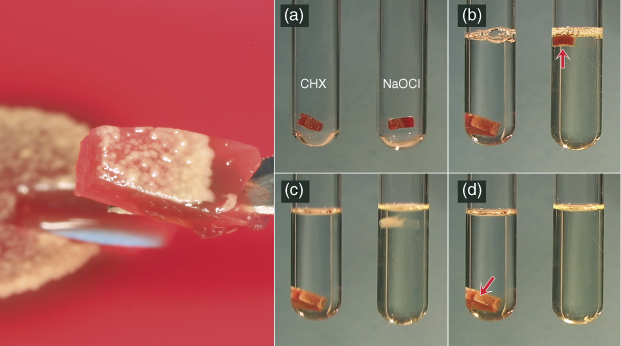
Figure 8.7 A heterogeneous mixture of oral microbiota was grown on blood agar and cut into small sections (left panel). (a) The agar and microbiota are placed in test tubes that will receive either 2% chlorhexidine (CHX) or 6% sodium hypochlorite (NaOCl). (b) Immediately after the solutions are added, the agar specimen in the NaOCl rises to the top as hydrolysis begins, indicated by the bubbles released on the bottom (red arrow). (c) A few minutes later, only a faint hint of the polysaccharide composing the agar is apparent. (d) Finally, both the agar and microbiota are completely reduced to carbon dioxide and ammonia, while both the agar and now dead microbiota in the CHX remain in their original state. In the root canal scenario, this debris would prevent adequate obturation.
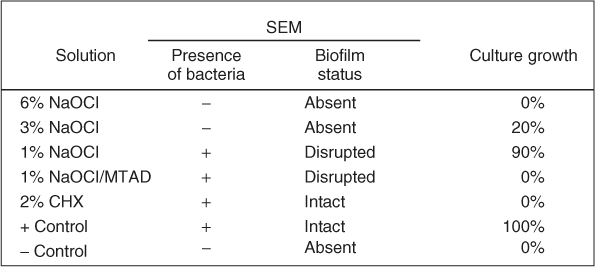
Figure 8.8 Only 6% NaOCl was able to completely remove biofilm and prevent growth.
The negative implication to NaOCl’s aforementioned positive attributes is safety. It can cause severe tissue reaction (35), neurological complications (36), facial deformity (37), life-threatening situations (38), as well as litigation considerations (39). Accordingly, the dental profession has been looking for methods to safely deliver NaOCl in concentrations higher than 3% safely to full working length. As mentioned earlier, because of the existence of an AVL, there are three proven methods to deliver NaOCl to full working length. The safety consideration of using positive-pressure delivery close to full working length is discussed later. Munoz and Camacho-Cuadra (12) demonstrated that irrigants can be delivered high up in the canal and worked to the apical termination with ultrasonic microstreaming. Alternatively, those irrigants may be pulled to the apical termination via apical negative pressure. Recently, three clinical studies tested both these methods. Cohenca et al. (40), using apical negative pressure irrigation (EndoVac), achieved 100% negative cultures both after final irrigation and 1 week recall. Paiva et al. (41), using passive ultrasonic instrumentation (PUI), failed to observe a significant difference in the bacteriologic status. The authors concluded that “although supplementary disinfection with either PUI or a final rinse with chlorhexidine can reduce the number of cases with positive culture and polymerase chain reaction results for bacteria, many cases still remain with detectable bacteria in the main root canal.” In contrast to the clinical study by Cohenca et al. (40), Pawar and coworkers (42) failed to identify a significant difference when using the EndoVac. However, it must be pointed out that their study used only 0.5% NaOCl instead of the 5% concentration recommended by the EndoVac technique—a difference of 1000%. It must be recalled that Clegg et al. (32) demonstrated that less than 3% NaOCl produced approximately 90% culture growth and did not remove biofilm. Pawar et al. (42) stated that “the use of 0.5% NaOCl in this study could be considered responsible for the lack of significant differences in antimicrobial efficacy between EndoVac irrigation and standard irrigation.” The Pawar study affirms the necessity to use NaOCl at concentrations higher than 3% during endodontic irrigation.
Irrigant activation via agitation
Proper instrument agitation in the amplitude range of 75 μm and driven at 30 kHz cavitation causes more effective intracanal debris removal than lower frequency and higher amplitude devices that are designed to operate in the range of 1.2 mm amplitude and 160 Hz (43). Furthermore, during ultrasonic activation, because the tip is smallest in diameter, it has the largest displacement thereby generating the largest shear stress. This is an important consideration once the NaOCl has been exhausted (9). However, microstreaming is dependent on the freedom of the instrument movement and, until recently, has not been evaluated for efficacy when its movement is restricted by a well-defined canal curvature. This point is addressed in detail later.
Chemical limitations of passive ultrasonic irrigation (PUI)
When the PUI technique is employed, the apical portion of the root canal is initially devoid of the irrigant (Figure 8.5a). Once PUI begins, acoustic microstreaming allows the irrigant to reach full working length (Figure 8.5b). Assuming all the irrigant delivered to the root canal system is circulated through the apical termination via microstreaming, the total volume would equal ≈0.0126 ml (Figure 8.9), as opposed to the ability of apical negative pressure (EndoVac) to circulate 1.8 ml at full working length (44). Thus, a difference of ≈14,000% volumetric exchange is realized between apical negative pressure irrigation and acoustic microstreaming via PUI, with the former providing a constant supply of NaOCl (>5%) that results in a consistent high-reaction velocity without irrigant depletion. Although the physical action of acoustic microstreaming is active during PUI, the ability of NaOCl to hydrolyze existing organic tissues is quickly exhausted, because less than a quarter of a drop of irrigant can be circulated to the apical termination per application. Accordingly, the problems with PUI are fluid exchange and concentration resulting from fluid dynamics encountered in a closed root canal system.
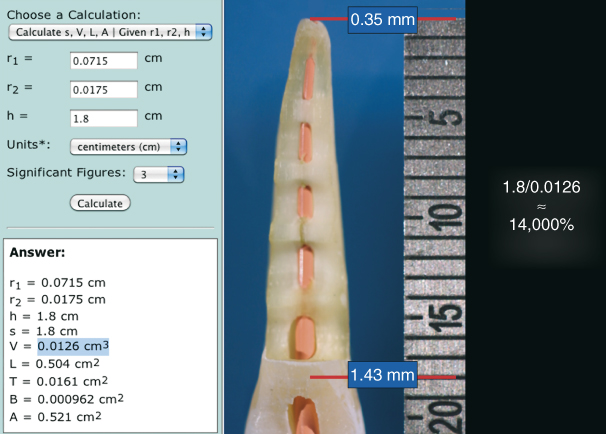
Figure 8.9 The volume of space in a root canal may be roughly calculated using a frustum calculator. In this example, a 1.8 cm long root canal instrumented to size 35, 0.06 taper would hold about 0.0126 ml of fluid. Assuming an irrigant delivery needle is not positioned closer than 4 mm from the working length, the apical vapor lock in the terminal 3 mm will not be filled until microstreaming is initiated. At that point, the clinician has only about 0.0126 ml of NaOCl to activate. The NaOCl will be quickly exhausted. However, apical negative pressure can draw approximately 1.8 ml of irrigant through the entire working length. That is approximately a 14,000% volumetric differential and helps to maintain the hydrolysis reaction at its fullest potential throughout the final irrigation phase.
In concert with his clinical study, Cohenca and coworkers (45, 46) conducted two in-vitro studies using teeth infected with Enterococcus faecalis biofilms and examined the results of the final irrigation via culturing technique as well as SEM examination of the canal walls. Figure 8.10 demonstrates results from the Hockett study (45) comparing different preparation techniques and used a traditional irrigation technique or apical negative pressure for the final irrigation. Regardless of the preparation technique, all specimens treated with the EndoVac system tested negative, while 66% of the teeth treated with the traditional irrigation technique tested positive for microbial culturing. Figure 8.10a shows a thick biofilm at the apical termination. Figure 8.10b shows the biofilm completely removed; the absence of dentinal tubules is indicative that this section has been taken in the apical 2 mm of the root canal, wherein the tubules are obliterated by sclerotic mineral casts. Figure 8.10c shows dentinal tubules devoid of bacteria while the canal wall surface is devoid of bacteria or smear layer. SEM examination confirmed complete eradication of the canal wall biofilms. Figure 8.11 demonstrates results from the Saldivar study (46) that tested the efficacy of PUI in addition to apical negative pressure and traditional irrigation techniques. In this study, the EndoVac again produced 100% negative cultures, while the PUI group produced 53% positive cultures. The positive control (Figure 8.11a) demonstrates thick biofilms in the last apical 2 mm of the canal space. The PUI group (Figure 8.11b) shows canal walls covered by smear layers created during PUI as well as some intact clusters of E. faecalis. The EndoVac group (Figure 8.11c) demonstrates clean open tubules and complete eradication of bacteria. It is encouraging that the PUI technique had a profound effect on reducing the extent of biofilm, despite the fact that irrigant exchange at the apical termination was minimal following the fluid dynamics of irrigant exchange mentioned in the previous paragraph.
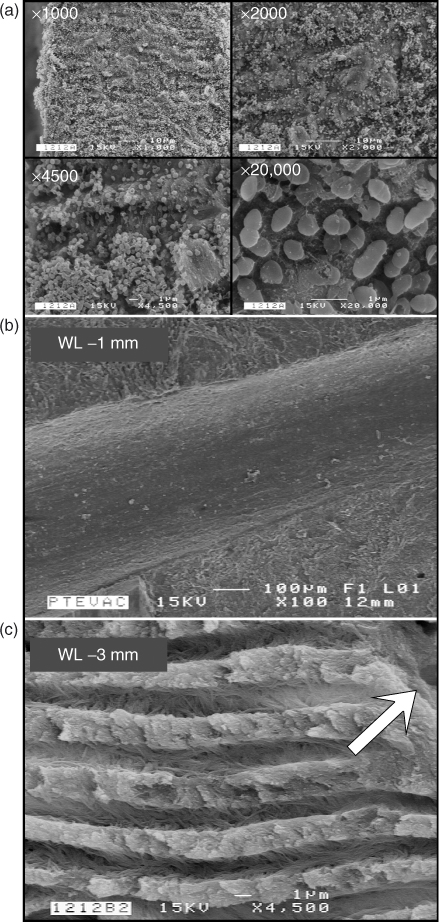
Figure 8.10 (a) A series of four SEM images taken with different magnifications from the positive control showing the presence of a thick biofilm in the apical 2 mm of the instrumented canal space. (b) SEM image of the instrumented canal wall at 1 mm from the working length. The canal wall is devoid of patent dentinal tubule orifices (sclerotic radicular dentin) and is completely devoid of bacteria biofilms. (c) SEM image of fractured radicular dentin at 3 mm from the working length. The surface of canal wall (arrow) is rendered smear-layer-free by the use of apical negative pressure irrigation and contains patent tubular orifices. No bacteria can be identified within the subsurface dentinal tubules.
(Hockett et al. (45). Reproduced with permission of Elsevier.)
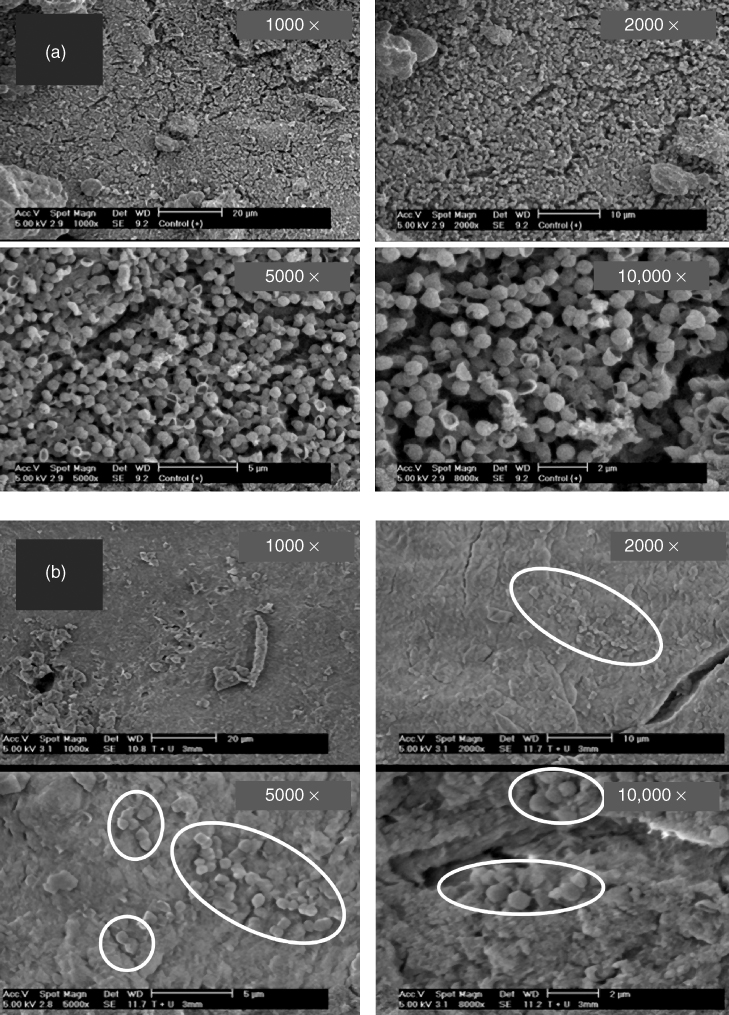
Figure 8.11 (a) A series of SEM images taken at different magnifications from the positive control showing thick biofilm formation in the apical 2 mm of the canal wall. (b) A series of SEM images taken at 3 mm coronal to the working length showing the conditions of the canal wall following irrigation with passive ultrasonic irrigation (PUI). Clusters of bacteria could be identified from the debrided canal wall (enclosed in ovals). (c) A series of SEM images taken at 3 mm coronal to the working length showing complete absence of smear layer, patent dentinal tubule orifices, and absence of bacteria after irrigation with 5.25% NaOCl and 17% EDTA with EndoVac negative apical pressure irrigation technique.
(Images c/>
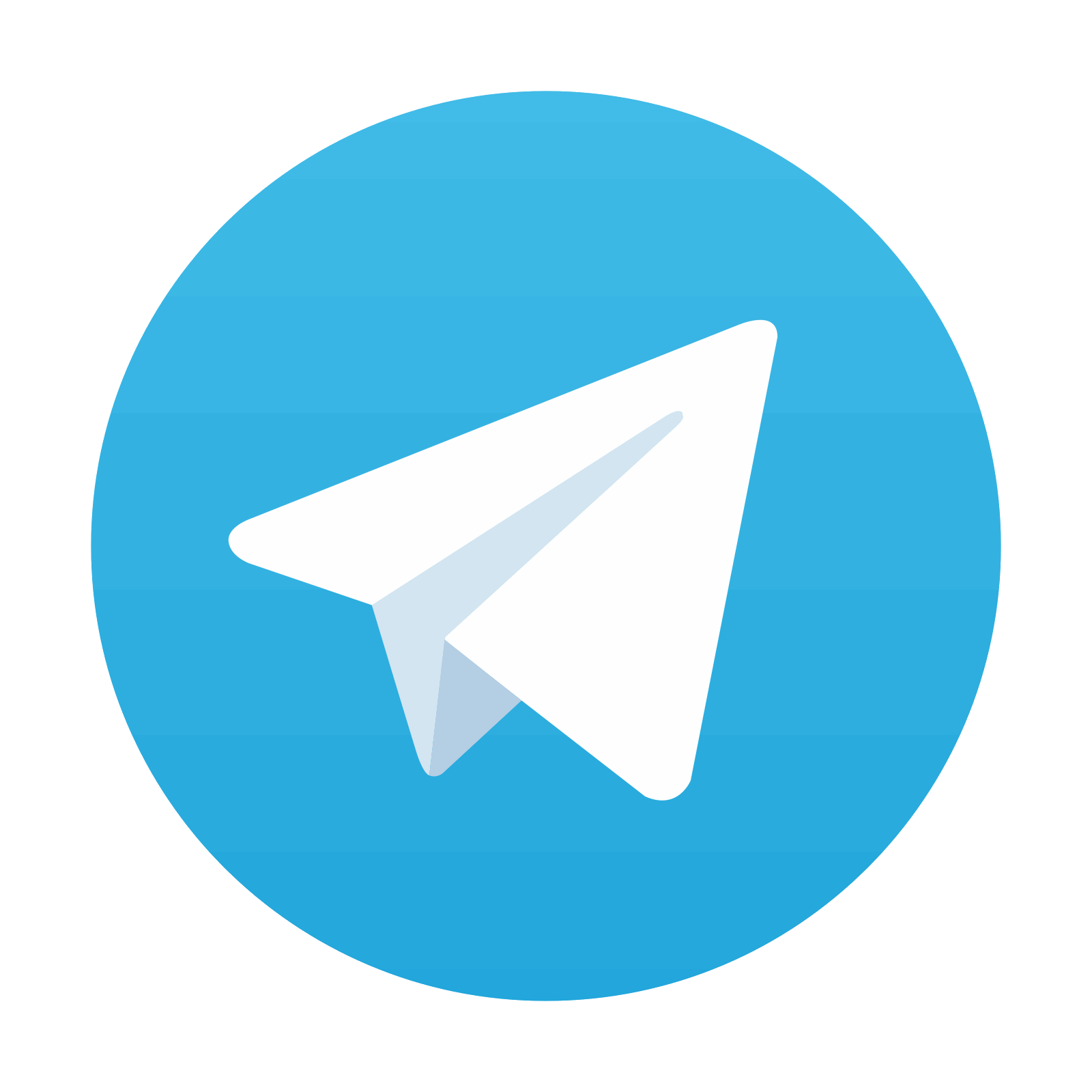
Stay updated, free dental videos. Join our Telegram channel

VIDEdental - Online dental courses
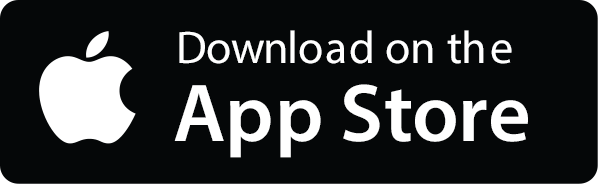
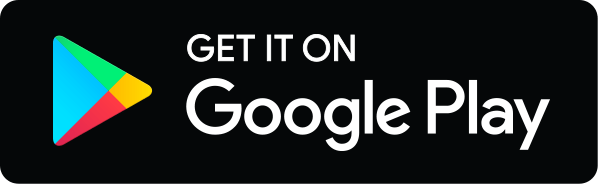