8
Anchorage Problems
Introduction
Definition
Classification of anchorage
Intramaxillary anchorage
Soft tissue anchorage
Free anchorage
Intermaxillary anchorage
Occlusion
Differential timing of force application
Extraoral anchorage
Skeletal anchorage
Prosthodontic implants
Temporary anchorage devices
Indications
Complications
Future of TADs
References
Introduction
Anchorage in orthodontics is a critical issue independent of the technique or philosophy followed by a clinician. Inadequate anchorage can be the most limiting factor of the therapy and unwanted side effects are frequently seen due to insufficient anchorage. In relation to the treatment of adult patients, many of the traditional means of obtaining anchorage are only of limited use.
In young and growing individuals, tooth movement depends on the interaction between ongoing growth and changes produced by the orthodontic appliance. Expressions such as ‘favourable or unfavourable growth pattern’ are often found in the orthodontic literature, clearly reflecting the impact of growth on the orthodontic treatment result. In the case of the adult patient, there is no growth to interfere with the effect of the forces generated by the orthodontic appliance and the tooth movement reflects the applied force system more closely. However, the soft tissue balance and muscle function still modify the effect of orthodontic treatment that aims to change the arch form or the facial height.
Definition
The first mention of orthodontic anchorage was in the Standard Dental Dictionary where the word was explained as ‘the base against which orthodontic force or reaction of orthodontic force is applied’ (Ottofy 1923). This definition did not assign any value to the word but it is clear from the definition of anchorage given by Daskalogiannakis (2000) that loss of anchorage is undesirable. In the ‘Glossary of Orthodontic Terms’, anchorage was defined as resistant to unwanted tooth movements of the reactive unit. Proffit offered a more detailed definition when he described anchorage as the resistance to reaction forces usually provided by other teeth or sometimes by the palate, head and neck, or implants in bone (Proffit and Fields 2000).
Classification of Anchorage
Conventional orthodontic anchorage can be classified as intraoral or extraoral (Melsen and Verna 1999). The former can be divided into intramaxillary and intermaxillary.
- Intraoral intramaxillary anchorage can be further subdivided into dental and extradental. In the case of extradental, the reactive forces will not be distributed to other teeth but to other tissues, that is, the mucosa of the palate, such as in the Nance appliance, or to alternative devices, such as wires or metallic implants.
- Intermaxillary anchorage comprises Class II and Class III elastics in addition to different types of bite-jumping appliances such as the Herbst appliance, bite correctors and the various types of appliance transferring forces from one arch to the other. Occlusion itself is also a type of intermaxillary anchorage.
- Extraoral anchorage implies reactive forces transferred to the head, the neck or both at the same time or to the chin.
In the work-up of the treatment goal it should be determined which teeth will be moved, i.e. belong to the active unit, and which will be considered anchorage i.e. belong to the reactive unit. In relation to space closure, anchorage has been defined as (Burstone 1982):
- Type A, when space is closed primarily by retraction of anterior teeth
- Type B, when there is equal contribution of the anterior and posterior units to closure of space
- Type C, when space closure is primarily by protraction of posterior units.
Type B anchorage can be considered the easiest to achieve, since movement of both units is desired, while type A and, especially, type C anchorage are more critical, since the movement of the active unit must take place without any movement of the anterior reactive unit.
Many different approaches have been applied in order to obtain differential tooth movement in spite of the fact that every orthodontic appliance stays in a state of equilibrium. When inserting a continuous levelling arch, the force distribution will be a product of the mutual position of the teeth in each arch (Burstone and Koenig 1974) and there is no differentiation between an active and a reactive unit. Differentiation can only be obtained by segmenting the appliance (Burstone 1962, 1966; Ricketts 1980; Mulligan 1998). The appliance connects the active part of the dentition, the part to be moved, and the reactive anchorage unit, which should remain unaltered, and is mostly designed to deliver a specific force system to the active units. The force system delivered to the reactive unit, on the other hand, will maintain the equilibrium of the system independent on the type of appliance. The total sum of moments and forces generated by the appliance is always zero according to the third law of Newton. However, the line of action of the force can pass at different distances from the centre of resistance, for which reason the moment-to-force ratio may differ with respect to the two units, giving rise to differential tooth movements. When the line of action is not parallel to the occlusal plane, the equilibrium will be maintained through the generation of a force rotating the unit, in this case influencing the occlusal plane (Fig. 8.1) (Melsen and Bosch 1997).
Fig. 8.1 (1) Sagittal force application between the brackets on two teeth. Two equal and opposite forces and moments are generated. (2) If the force vector is not parallel to the occlusal plane different moments will be generated at the two units and the vertical forces will affect the occlusal plane.
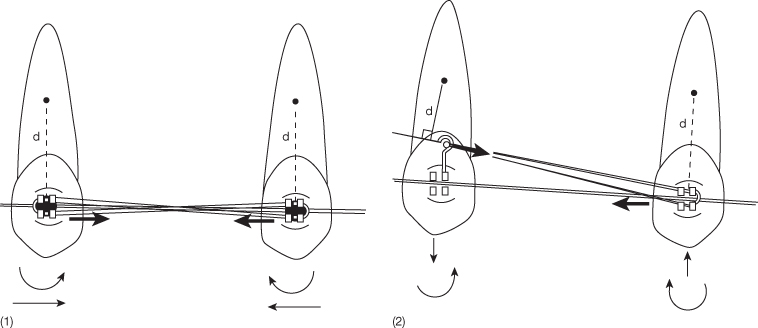
In the following sections, the basic principles of anchorage will be presented and the scientific and biomechanical background will be discussed.
Intramaxillary Anchorage
The traditional concept of anchorage is based on the use of many teeth against fewer teeth (Fig. 8.2). The principle behind this concept is that undesirable tooth movements of the anchorage unit will not occur because the forces acting on the anchorage unit are below the necessary threshold value required to generate movement. This hypothesis was supported by Quinn and Yoshikawa (1985), who found a linear relationship between force level per tooth and rate of tooth movement in six clinical studies, and by Freeman (1965), who instead of using the number of teeth, assigned anchorage values based on the root surface area. However, neither the number of teeth nor root surface measurements can be used as expressions for resistance to movement, since the alveolar surface is only vaguely related to root surface, as recent data obtained by micro-computed tomography (CT) of alveolar bone (Dalstra et al. 2006) demonstrates a pronounced variation in level, density and quality of alveolar bone. A more plausible explanation in support of the more teeth against fewer teeth theory is most likely grounded in the contribution of the occlusion to the anchorage.
Fig. 8.2 Distalization of canines against six posterior teeth. In spite of the larger number of teeth in the anchorage unit, this set-up frequently leads to anchorage loss. Occlusal forces can prevent the loss of anchorage. (1) The canine being distalized against the posterior teeth with coil springs to the power-arms. (2) Power-arms are also placed lingually in order to avoid rotation.
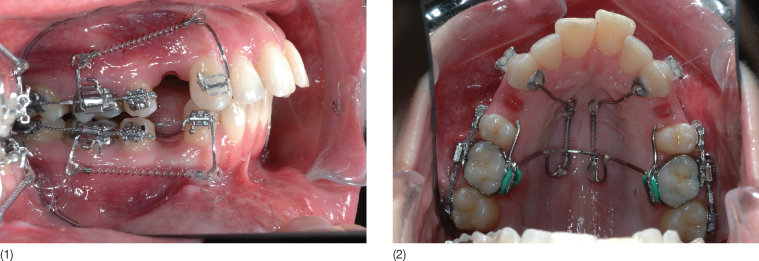
The more teeth against fewer teeth hypothesis was never supported scientifically as Weinstein in 1967 demonstrated that a few grams of force are sufficient for movement of a tooth. More recent studies focusing on the optimal force magnitude for orthodontic tooth movement have further shown that there is no scientific evidence regarding a threshold for force level that would switch on tooth movement (Ren et al. 2003). Minimal forces, leading to minute changes in the stress–strain distribution within the periodontal ligament (PDL) may be able to trigger the necessary tissue reaction. This implies that the higher force levels commonly used in orthodontic treatments do not necessarily enhance tooth movement but may have negative effects, such as hyalinization, which can delay tooth movements (Reitan 1967).
Another common anchorage principle has been the use of rigid wires such as transpalatal arches, lower lingual arches, cast structures or stiff stainless steel wires to keep the teeth of the anchorage unit together (Fig. 8.3). The advantage of a rigid wire is in prevention of intra-segmental displacement. The combination of stiff passive wires and large segments allows for the maintenance of chewing function and thereby a positive interaction of occlusion with the forces attempting to displace the anchorage. However, bending of a heavy stainless steel wire passively is extremely difficult. It is therefore advantageous to bond the wire directly to the teeth of the reactive unit (Fig. 8.4). The teeth of the reactive unit can also be consolidated with fibre-reinforced composites (Freudenthaler et al. 2001) (Fig. 8.5).
Fig. 8.3 (1) Transpalatal bar used to reinforce the anchorage unit. (2) Iron cross used to consolidate the posterior unit to improve anchorage. (3) Iron cross soldered to bands. (4) Iron cross soldered to custom-made cast bands for the posterior segments. (5) Cast anchorage units extending from 13 to 28. 17 is being mesialized with a coil spring extending from the anchorage unit to a transpalatal bar connected to the left side with a hinge. The transpalatal bar prevents rotation of the molar during the mesial movement.
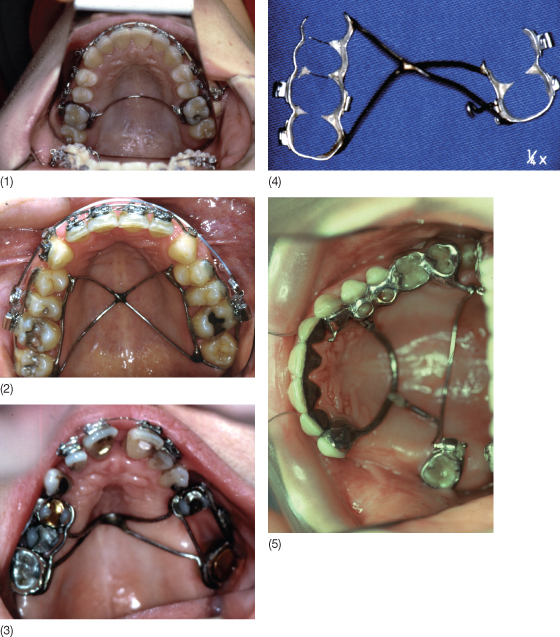
Fig. 8.4 Passive buccal segments constructed from 0.021 × 0.026 inch stainless steel wires onto which small metal bases are welded. These passive segments are bonded directly on the teeth that should remain mutually passive throughout the treatment.
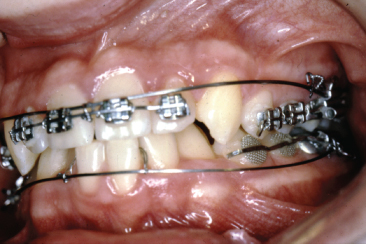
Fig. 8.5 (1,2) Consolidation of the reactive unit by fibre-reinforced composites. The active unit is the molar to be uprighted.
(Courtesy of CJ Burstone and P Depasquale.)

In the light of the recommendation to use rigid wires as anchorage, it may seem controversial that several authors (McLaughlin et al. 2001; Damon 2005) recommend that sliding of teeth (the active unit) should be done only on heavy wires. The reason for doing this, however, is to avoid the bending of the wire and thereby the undesirable tipping and anchorage loss that will occur when sliding along a flexible wire. The latter would lead to pronounced jiggling, which would increase the risk of root resorption. However, a stiff wire generates more friction and the lace-backs must be reactivated frequently (Fig. 8.6).
Fig. 8.6 Lace-backs for distal movement of canines following extraction of first premolars.
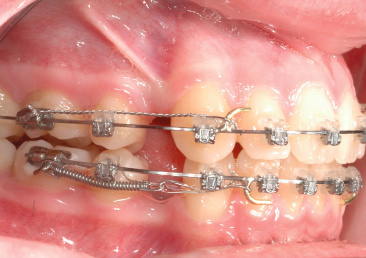
The hypothesis that rigid wires render more anchorage than flexible wires can be explained by the influence of both friction and binding, both of which increase with increasing wire dimension (Drescher et al. 1989; Kusy and Whitley 1997). Friction is positively correlated with the size of the wire–bracket contact surface whereas binding is a product of the wire–slot angle (Kusy and Whitley 1999; Thorstenson and Kusy 2002; Kusy 2004).
Another traditional concept, introduced by Tweed (1966) and still used by many clinicians, is that of anchorage preparation. The basis behind the concept is that the direction of the force with respect to the root is related to the anchorage delivered by the teeth. During anchorage preparation, the lower molars and premolars are tipped distally by adding tip-backs to the lower buccal segments, usually 5° for each tooth with respect to the preceding tooth. Class II traction would thereby act almost perpendicularly to the distally tipped molars, which are therefore anticipated to generate better anchorage than if the teeth had been in their normal inclination. A description of the distribution of the resorptive and the appositional areas with and without anchorage preparation has been used to explain the rationale behind this hypothesis. Scientifically, the concept cannot be supported and the effect may in fact be the opposite to that intended. The tip-back could lead to bite opening and posterior rotation of the mandible that could even accentuate the Class II malocclusion already present (Fig. 8.7). In addition, the beneficial effect of occlusion would be lost, since the dorsal tipping of the molars would reduce the occlusal forces considerably. This theory is also in complete contradiction to the view that anchorage teeth should be kept stable and cellular activity around the reactive unit should be as low as possible in order to maximize the anchorage potential.
Fig. 8.7 Anchorage preparation by distal tipping of the molars so that the force will be more perpendicular to the roots when Class II traction is used. (1) The force systems generated during anchorage preparation. (2) Tooth movement resulting from the anchorage preparation.
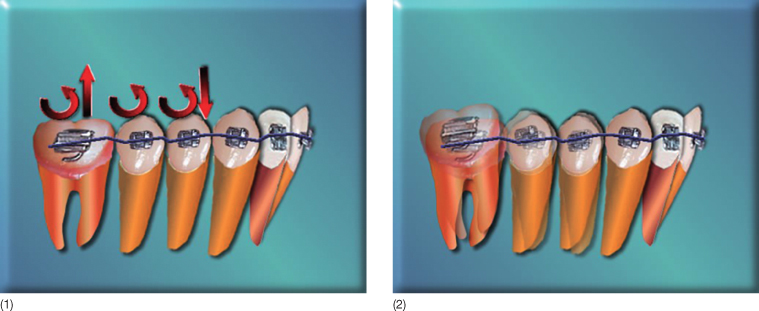
The difference in bone turnover between cortical and trabecular bone led Ricketts (1976a,b) to introduce the concept of cortical anchorage. The theory is based on the observation that when the roots of molars are moved against or into the buccal cortex before serving as anchorage, their anchorage potential increases due to the greater resistance of cortical bone. However, there is no evidence that roots cannot move through cortical bone, as alveolar bone dehiscences and fenestrations with root surface exposures have clearly demonstrated the opposite in an animal model (Thilander et al. 1983). The key to the ‘cortical anchorage’ must therefore be the lag time between force application and tooth movement. This lag time is longer for cortical bone than for trabecular bone, since fewer osteoclasts and osteogenic cells are available to accomplish the remodelling process. The initial movement through cortical bone is thus delayed but once started the cortical bone perfectly allows the tooth to be moved through it.
Differential anchorage, introduced by Burstone (1982) and Melsen et al. (1990), is based on the fact that tipping is an easier tooth movement to achieve than translation (bodily movement) and that the moment-to-force ratio delivered to the bracket determines the type of tooth movement (Figs. 8.8 and 8.9). The limitation, though, is the inevitable generation of vertical forces during the deactivation phase, which can generate unwanted tooth movements, if not balanced by occlusion or adjunctive orthodontic mechanics (Fig. 8.10). Both vertical forces and the large moment often delivered to the posterior unit may result in distal tipping of the posterior segment, leading to the development of two occlusal planes and a primary contact in the premolar region. Burstone and Koenig (1976) advocated the addition of a high-pull headgear with a short extraoral bow to control the distal tipping and the extrusion of the posterior segment. However, this solution is not ideal, as the extraoral traction is only used intermittently and with a different force magnitude than the mechanics applied for space closure.
Fig. 8.8 Different types of tooth movement depending on the moment- to-force ratio delivered to the bracket.
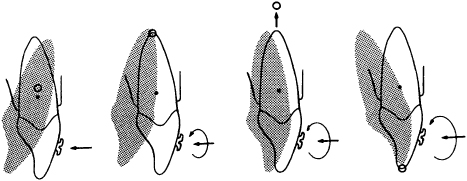
Fig. 8.9 Type A and C anchorage. In the case of type A anchorage, the anterior teeth will be displaced and the posterior teeth serve as anchorage. The line of action of the necessary force system is indicated and passes below the centre of resistance (CR) with respect to the active unit whereas it passes through the CR of the anchorage unit. The space closure will thus start with controlled tipping, intrusion of the anterior unit and translation and extrusion of the posterior anchorage unit. In the case of type C anchorage, the line of action of the force will generate translation, extrusion of the anterior unit and tipping and intrusion of the posterior unit.
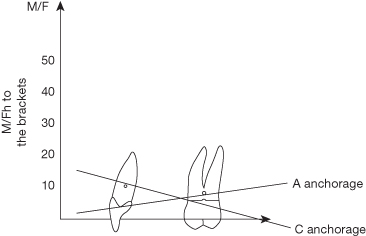
Fig. 8.10 (1) Illustration of a side effect of differential anchorage generated by a T-loop delivering a large moment to the posterior unit. This has resulted in a distal tipping of the posterior unit and thus two occlusal planes, which can be avoided by keeping moment to the anchorage unit below the level where posterior tipping moments are generated. (2) Clinical example of a posterior unit that has tipped distally as a result of a too-large moment delivered from the T-loop.
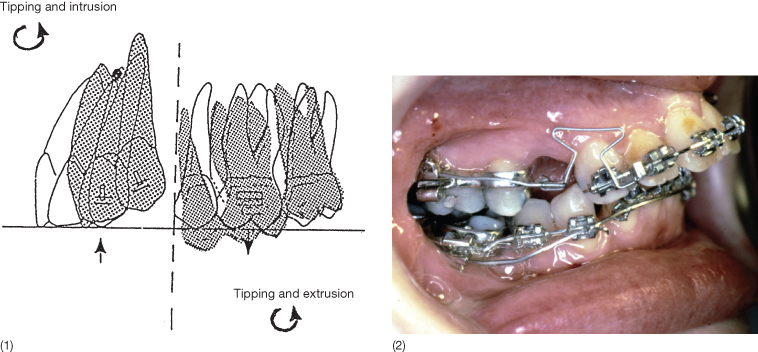
Soft Tissue Anchorage
With the aim of solving all the problems related to the patient’s compliance, many clinicians started developing intraoral devices that could minimize cooperation and be completely under the orthodontist’s control (Hilgers 1992; Jones and White 1992; Carano and Testa 1996; Fortini et al. 1999; Carano et al. 2002). The majority of these devices, generally used as molar distalization appliances, consist of an active force-delivering unit and an anchorage unit, e.g. a Nance button, an acrylic button held against the anterior part of the palate and connected by two stiff stainless steel wires to molar or premolar bands (Fig. 8.11). It has never been shown that the palatal mucosa can withstand the mesially directed molar relapse forces without being compressed and with problems such as inflammation, ischaemia and necrosis. Post-treatment evaluations of the efficiency of these compliance-free devices have demonstrated significant anchorage loss and increased anterior inclination of premolars and anterior teeth (Ngantung et al. 2001; Taner et al. 2003; Fortini et al. 2004), confirming the inability of these devices to deliver absolute anchorage.
Fig. 8.11 Nance button used as anchorage for distal movement of the molars in a 14-year-old boy. (1) At the beginning of treatment, a sectional jig assembly was used to distalize both the first and second maxillary molars simultaneously. The anchorage comprised a Nance acrylic button on the palate (palatal anchorage) connected by stainless steel wires to the premolar bands (dental anchorage). (2) The maxillary dental arch after 5 months of active treatment: distalization of the first molars to a super Class I molar relationship has been achieved. (3) Pre-treatment cephalogram. (4) Cephalogram after 5 months of active treatment. (5) Superimposition of the maxilla (best fit on the palatal surface) of the pre- and post-treatment cephalometric tracings. The Nance button and anterior teeth mesial to premolars were not able to resist the mesially directed reciprocal forces and anchorage loss is evident, expressed mainly through mesial inclination of the premolars and mesial movement and proclination of the incisors. In addition, unwanted distal tipping of the first molars is also observed.
(Courtesy of Moschos A. Papadopoulos.)
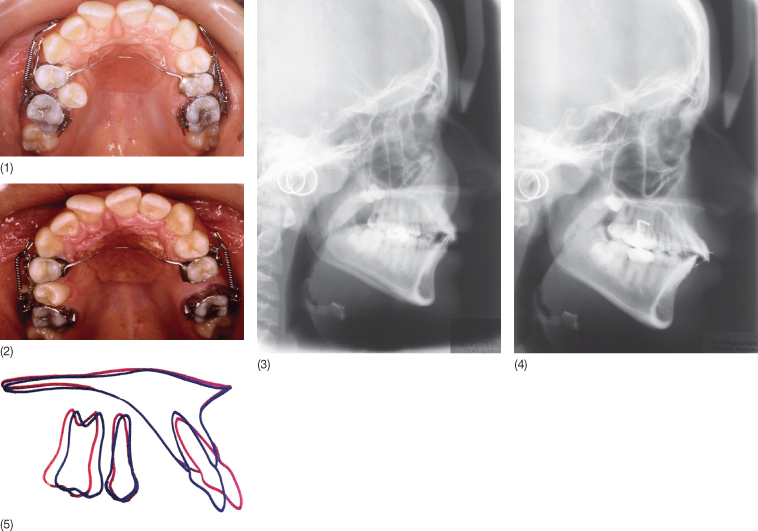
Free Anchorage
A completely different approach to anchorage is the application of the so-called ‘free anchorage’. Free anchorage indicates that no ‘price’ has to be paid in terms of undesirable force on teeth belonging to the anchorage unit. The principle is that reactive forces are transferred to teeth which are to be extracted according to the treatment plan, and so there are no adverse effects on the teeth that will remain in the arches following treatment.
The patient in Figure 8.12 was referred from the gnathological department as she had temporomandibular disorder (TMD) due to a laterally forced bite. Following treatment with a fully balanced splint, the symptoms disappeared but a crossbite remained and only few occlusal contacts were present laterally on the left side. In order to maintain the structural position of the mandible, intermaxillary elastics could not be used. In this case, the anchor tooth was the first right premolar planned for extraction. The patient had already had three premolars extracted in relation to orthodontic treatment and had an asymmetrical upper arch form. The asymmetry was caused by collapse of the maxilla on one side because of uncontrolled space closure following extraction of 24. The ‘free anchorage’ principle has also been used in the correction of arch asymmetry with collapse of both arches on the same side (Fig. 8.13).
Fig. 8.12 (1–3) Intraoral frontal view taken with the mandible in the structural position, which has to be maintained if the patient is to be free of symptoms. No intermaxillary elastics could thus be used. (4,5) Occlusal photographs of the upper and lower arches. Note the asymmetry of the upper arch created during space closure following extraction of 24. (6) 14 was used as anchorage. The first appliance was a transpalatal arch that moved 14 buccally and 26 buccally and distally whereby the crossbite was corrected. (7) The mandibular position was maintained by a bonded onlay on the lower teeth. (8) Second appliance. A transpalatal arch with built-in expansion was inserted. The expansion on the left side was desirable. The expansion on the right side was transferred to 14 by means of a cantilever. (9) As a result of the correction of the crossbite on the left side, 14 was now in scissors bite and could be extracted. (10–14) Post-treatment photographs. The crossbite had been corrected by unilateral expansion against the premolar that was later extracted.
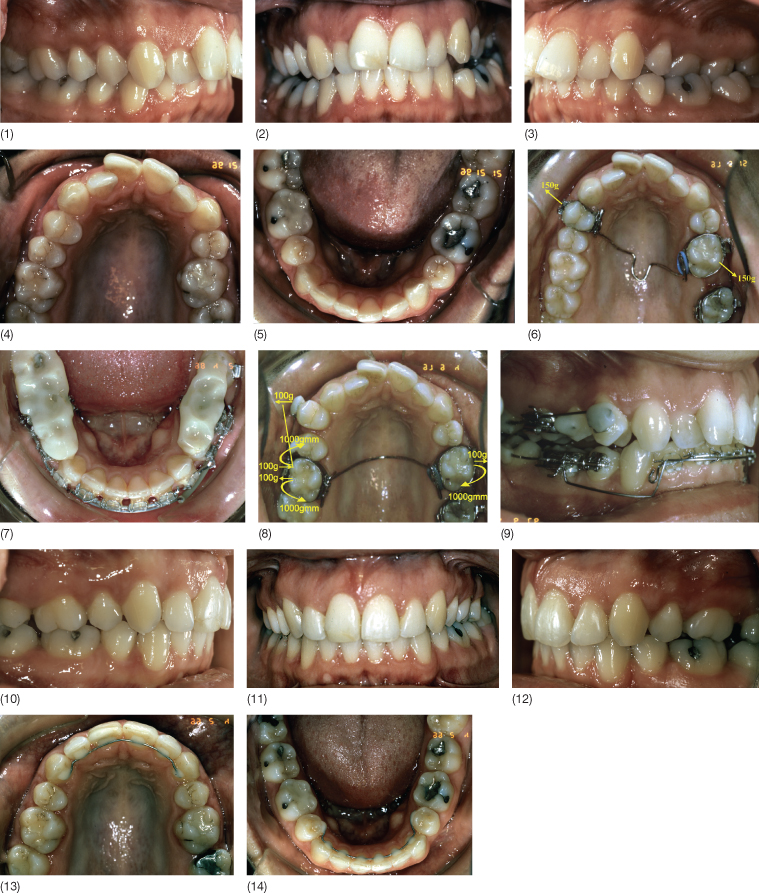
Fig. 8.13 (1–3) Intraoral photographs before treatment. The patient presented with bimaxillary protrusion and four premolar extraction treatment was indicated. (4,5) Occlusal photographs of the study casts demonstrating that both upper and lower arches were saddle shaped on the right side. (6) VTO indicating the need for unilateral expansion on the right side to achieve symmetrical arch forms. (7,8) Two premolars, one upper and one lower, were extracted on the right side. (9) The force system applied. It can be seen that the expansion force on the left side is being transferred to the premolar. Bilateral expansion combined with distal rotation was delivered by a transpalatal arch in the upper arch and by a lower lingual arch in the lower arch. On the right side, the effect was desirable; on the left side a cantilever was inserted and activated to deliver a buccally directed force to the left premolar. The reaction from this cantilever neutralized the expansion and rotational force acting on the molar. The appliance delivering the unilateral expansion is shown. (10–12) Following the unilateral expansion: note the expansion at the premolars to be extracted. (13–15) Once the desirable expansion was obtained, the premolars that had served as anchorage were displaced buccally and could be extracted. Unilateral expansion of both arches was achieved without any side effects and the case could be finished. (16–20) Intraoral photographs following treatment.
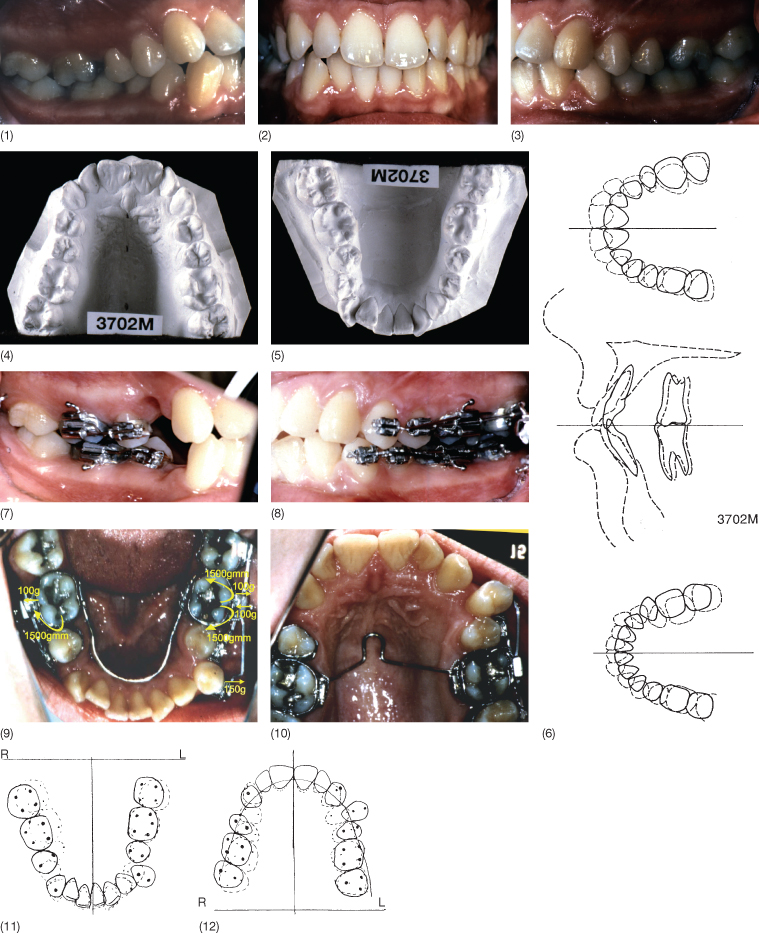
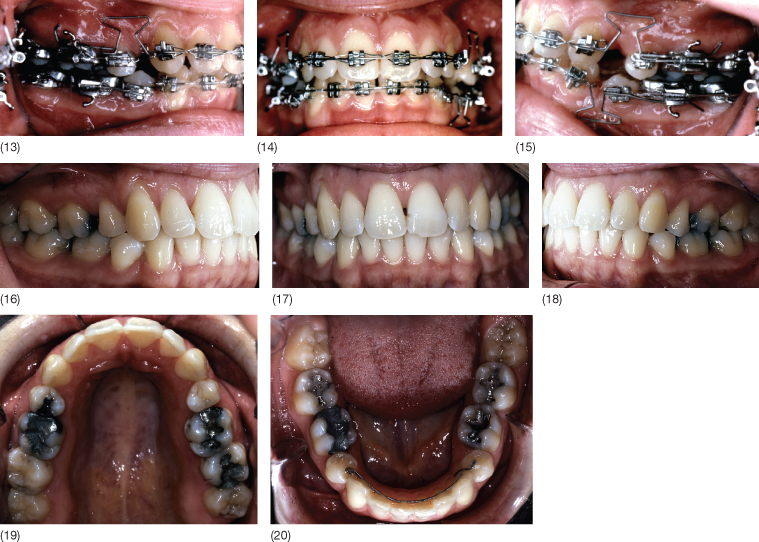
In so-called non-extraction cases, it is often desirable to remove the third molar if no space is available for free eruption. The third molar however, can also serve as free anchorage as seen in the patient in Case 1 (see the supporting companion website for this book: www.wiley.com/go/melsen). Another use of ‘free anchorage’ is via the use of ankylosed teeth. The use of ankylosed teeth was studied in rhesus monkeys in whom Guyman et al. (1980) ankylosed the lateral incisors. Parker et al. (1964) generated ankylosis by extracting the teeth and keeping them outside the socket for 60–75 minutes before reimplanting them. During that period, the pulp was extirpated and the roots were allowed to dry out. Eight weeks after reimplantation, the teeth showed signs of ankylosis and were loaded. During the loading, the teeth remained stable and histological examination carried out at the end of the experiment demonstrated replacement resorption of the root surfaces. Several authors have ankylosed deciduous canines and used them as abutments for maxillary protraction (Kokich et al. 1985; Omnell and Sheller 1994). Although ankylosed deciduous canines have proved to be efficient, the drawback is that the age group in which this can be applied is rather limited. However, if a tooth ankyloses either spontaneously, as often seen in temporarily ankylosed second deciduous molars or secondary to trauma, it can deliver anchorage for movement of teeth within the same arch in any direction. Kofod et al. (2005) used an upper incisor that had been ankylosed as a result of earlier trauma as anchorage for the correction of distal occlusion before the tooth and its surrounding bone was extruded by a distraction osteogenesis to the normal occlusal level (Fig. 8.14).
Fig. 8.14 (1) Intraoral photographs before treatment. The left upper incisor had luxated and was reimplanted several years earlier and was ankylosed. (2) This tooth was used as anchorage during the correction of the distal relationship in both posterior segments. (3,4) Following the correction of the sagittal problem, the ankylosed tooth and the adjacent bone were extruded by distraction osteotomy. (5) View immediately after the distraction of the ankylosed tooth.
(Kofod et al. (2005). Reproduced with permission from Elsevier.)
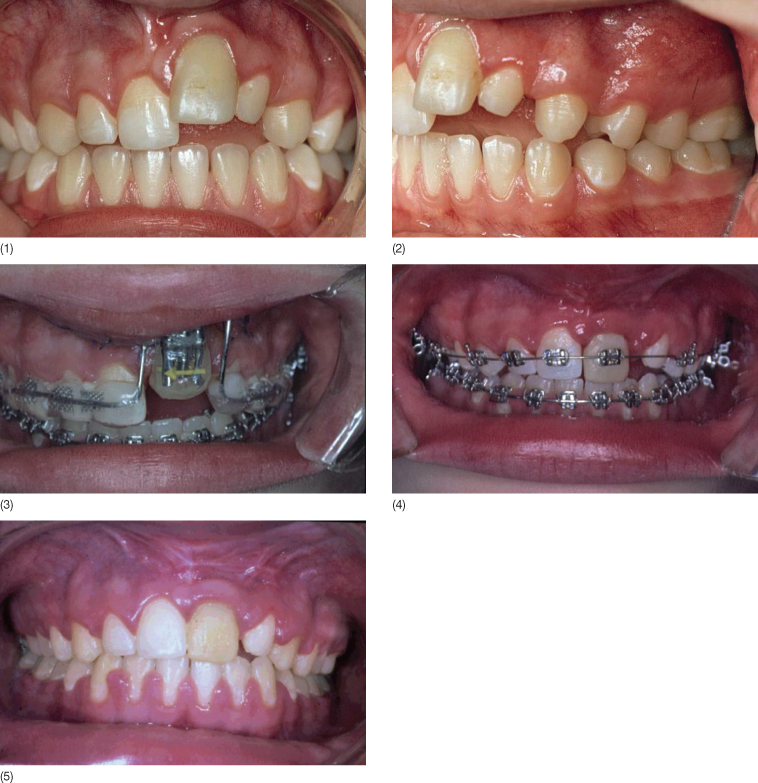
Intermaxillary Anchorage
Intermaxillary elastics were introduced in 1904 by Baker and are widely used today in association with fixed appliances. Their use for anchorage purposes (Kanter 1956) derives from the need to move the teeth in one jaw in one direction while moving the teeth in the other jaw in the opposite direction. The side effect generated by intermaxillary traction are due to the vertical force components, causing extrusion and changes to the inclination of the occlusal plane (Fig. 8.15). Other disadvantages are the intermittent nature of the forces, the dependence on the patient’s compliance and the unavoidable changes in the inclination of the incisors.
Fig. 8.15 (1,2) Forces generated when Class II or Class III traction is used.
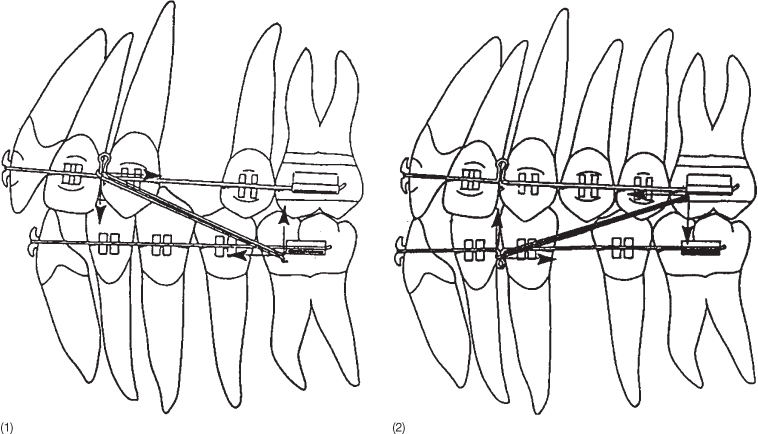
Bite-jumping devices, such as the Herbst appliance and Jasper jumpers, are compliance free and are often used to support type A anchorage in the treatment of class II malocclusions but they can also be used to support the treatment of difficult situations such as type C anchorage (Fig. 8.16) (Fiorentino and Melsen 1996). As in the case of intermaxillary elastics, vertical forces are developed with bite-jumping devices, but there are intrusive force components acting on the upper molars and on the lower anterior region.
Fig. 8.16 (1) Patient with an increased overjet and agenesis of 35, and 75 was retained. (2) A Herbst appliance was used in order to correct the sagittal discrepancy; since the patient had agenesis of 35, the Herbst appliance was retained in the mouth on the left side and used as anchorage for the forward displacement of the molars to close the spaces. (3) Panoramic radiograph demonstrating the mesial movement of the lower left molars. (4) Post-treatment view.
(With permission of Fiorentino and Melsen (1996).)
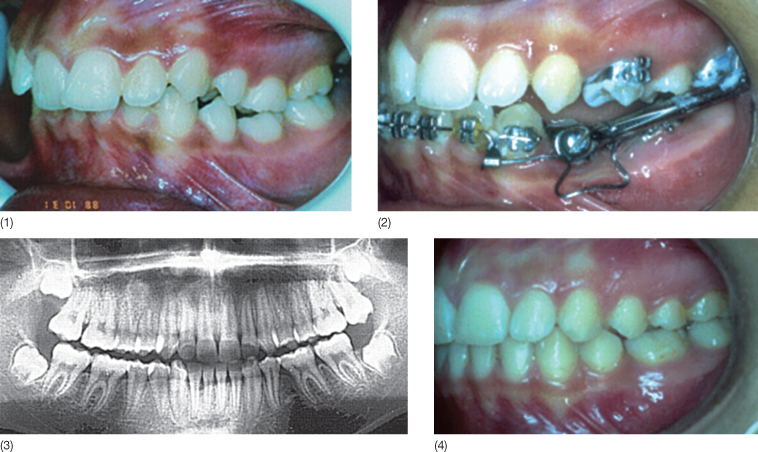
Occlusion
Among the intermaxillary anchorage approaches, occlusion probably plays the most important role. In order to maintain normal function, it is crucial that the proprioceptive input from the periodontium via the occlusion remains undisturbed. Only limited attention has been paid to the role of occlusal contacts in the stability of anchorage units. The proprioceptive input from occlusion is constantly changing during orthodontic treatment. Fewer occlusal contacts might be responsible for a reduction in the occlusal forces (Bakke et al. 1992). Since it has been argued that masseter muscle activity is reduced during orthodontic treatment (Miyamoto et al. 1996), chewing exercises can be considered an important factor to enhance maximal intercuspation and occlusal forces, especially in non-growing individuals (Miyamoto et al. 1999). Moreover, the masticatory muscle function seems to increase the density of cortical bone (Bresin et al. 1999).
Occlusal forces can be maintained by consolidation of the posterior teeth into ‘one big tooth’ with a full size stiff archwire and can further be enhanced by addition of a conventional transpalatal arch or an iron cross in the maxilla and a lingual arch in the lower arch. In addition to the consolidation, whether achieved with wires or fibre-reinforced composites, the stimulus from the occlusion can be increased by an occlusal build-up to maximize the occlusal contact (Fig. 8.12).
In adult patients, consolidation of the posterior segments is sometimes already present when the patient is first seen, e.g. if a repositioning splint has been cemented for the maintenance of the mandibular position in patients with TMD (Melsen and Verna 1999). The advantages of occlusal coverage is that the splints can also include edentulous areas and thereby enhance the occlusal feedback even from the teeth without contacts in the opposing arch. In some adult patients, the occlusal splint is the only possible solution, such as in patients with several missing teeth or satisfactory restorative dentistry consolidating the posterior teeth (Fig. 8.17).
Fig. 8.17 (1–3) Study casts of a patient who had undergone major prosthetic rehabilitation with two bridges on both sides of the mandibular arch. Two thin fully balanced splints connected with a lingual wire were used as anchorage for intrusion and proclination of the lower incisors. (4,5) The anchorage unit consists of two lateral splints covering the posterior bridges. The incisors were proclined, but instead of using brackets, the incisors were consolidated with a wire bonded lingually. The retroclined incisors were proclined and intruded. (6) The space created between the bridges and the incisors was closed by a mesial tipping of the canines. (7–9) Post-treatment photographs.
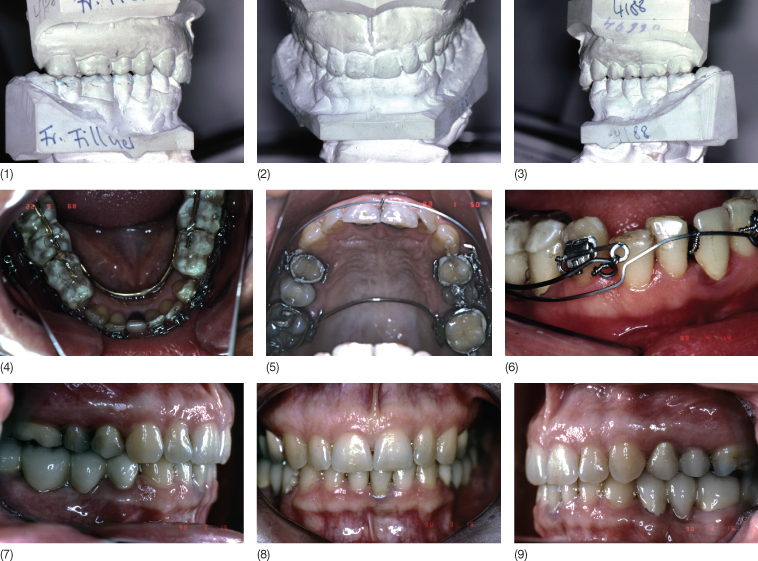
The inclination of the occlusal plane seems to play an important role. When the inclination of the occlusal plane is steep, the occlusal forces are reduced and loss of anchorage occurs more easily (Mavreas 1991). Maintenance of occlusion also prevents vertical loss of anchorage and no sagittal anchorage loss can occur without being accompanied by posterior rotation of the mandible. The first to perceive changes in the occlusion is the patient, sensing even minor occlusal changes. When a patient reports an altered sensation, it may be time to change the force system or lower the force level. Patients should be encouraged to chew and exercise to stimulate and thereby enhance the proprioceptive input from occlusion (Spyropoulos 1985).
Differential Timing of Force Application
In addition to the above-mentioned principles, the timing of the force delivery has been mentioned among the principles underlying use of anchorage. Proffit and Fields (2000) wrote ‘Clinical experience suggests that there is a threshold for force duration in humans in the 4–8 hours range, and that increasingly effective tooth movement is produced if the force is maintained for longer durations’.
In case of type A anchorage, the anchorage would be assured by the alternation of the use of inter- and intramaxillary elastics, i/>
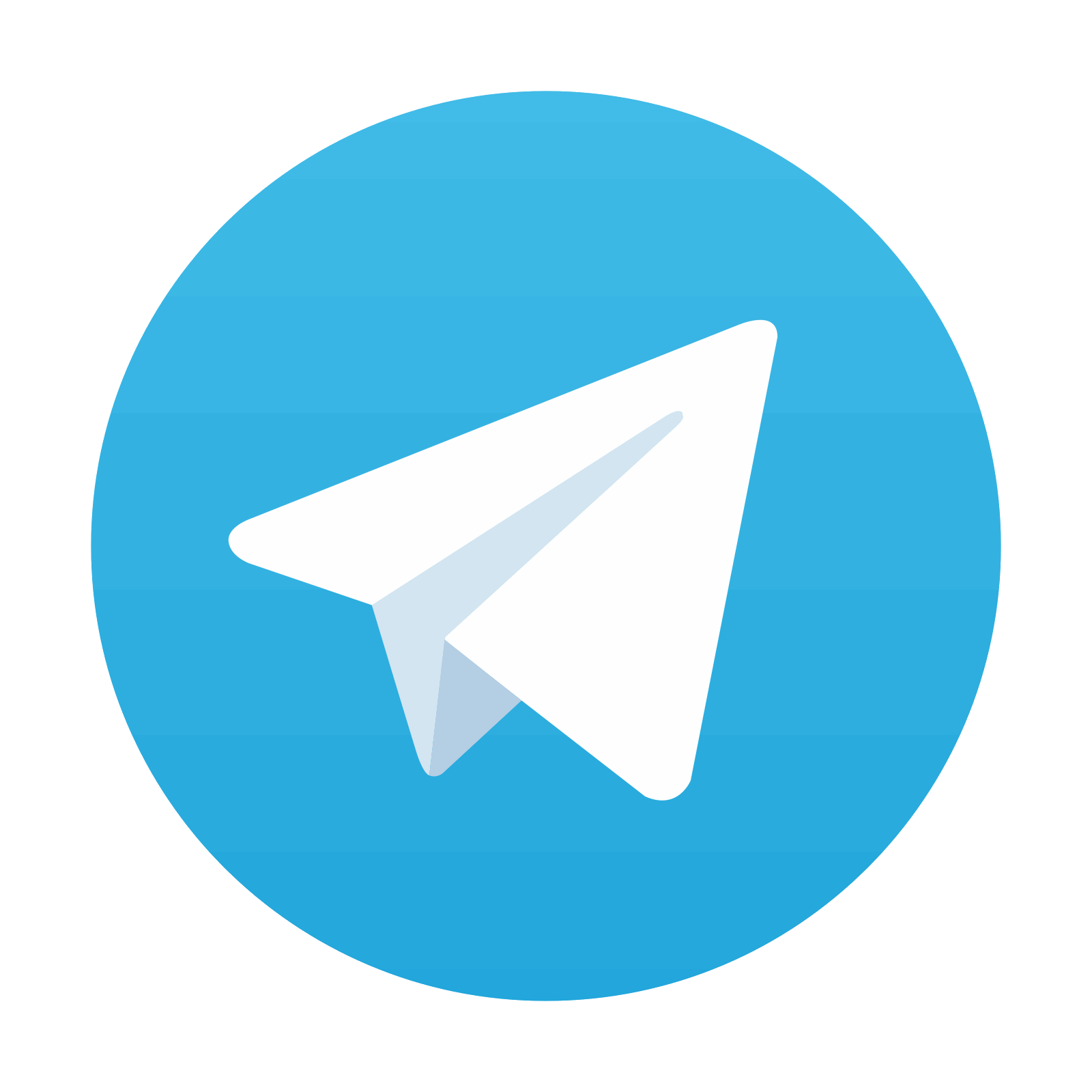
Stay updated, free dental videos. Join our Telegram channel

VIDEdental - Online dental courses
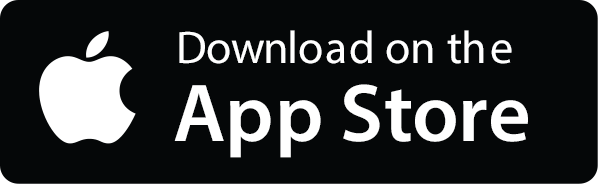
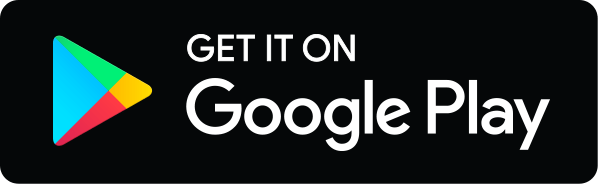