Biomechanics, Prosthetic Considerations, and Treatment Planning
Clinical research has demonstrated excellent long-term success rates for implant-supported restorations in fully and partially edentulous patients. The replacement of missing teeth with an implant-retained prosthesis restores function and esthetics, improves confidence and self-esteem, and enhances quality of life. However, it is important to recognize that implant success is achieved by following certain principles. Proper treatment planning is essential for predictability and success. One of the most critical factors in planning is the control and distribution of stress for preservation of the “biologic” connection between the implants and bone as well as the prevention of “mechanical” breakage of implants and restorative components. The quantity and quality of bone available to support implants play an important role in stress distribution,2 maintenance of bone apposition, and support under functional loading.
Biomechanical Considerations
Osseointegrated dental implants provide a predictable means of replacing missing teeth and restoring dental function. A thorough understanding of implant biomechanics is essential if implant-retained restorations are to be employed predictably. The load-bearing capacity of implants supporting the restoration must be greater than the anticipated loads during function. If applied loads exceed load-bearing capacity, it may lead to mechanical and/or biologic failure. In the case of mechanical failure, screws that secure the restoration may bend, loosen, or fracture. The most devastating type of mechanical failure is fracture of the implant. In the case of biologic failure, a resorptive–remodeling response of the bone around the implant(s) is provoked, leading to progressive bone loss.13,25 If bone loss around the implant progresses until the implant is no longer supported, osseointegration may be lost resulting in implant failure.
The function and support of implant-retained restorations is quite different from tooth-retained restorations. Teeth are suspended within the supporting alveolar bone by a periodontal ligament, which allows slight physiologic “movement” of teeth in function. If forces are excessive, teeth have the capacity to adjust or move in response to the applied forces. In the absence of inflammatory periodontal disease, teeth will adapt to these forces without appreciable bone loss. Orthodontic movement of teeth through alveolar bone is the ultimate example of the capacity of teeth to adapt to excessive applied forces. As orthodontic forces move a tooth, bone resorbs in response to pressure and forms in response to tension applied by the “attached” periodontal ligament fibers. Osseointegrated dental implants, by definition, are in direct contact with the alveolar bone without intervening soft tissues; there is no periodontal ligament and should movement occur, it would indicate a loss of osseointegration (e.g., fibrous encapsulation).12 Because excessive forces are destructive, it is crucial to have a good understanding of the biomechanical properties and the limitations of dental implants to ensure proper treatment planning that will sustain the anticipated occlusal forces.
Load-Bearing Capacity
The load-bearing capacity of implants is influenced by several factors, including the number and size of implants, the angulation and arrangement of implant positions, and the quality of the bone-to-implant interface (Box 74-1).
The number of implants used will influence the load-bearing capacity. In the 1980s and early 1990s, many posterior quadrants of the maxilla were restored with one or two implants, and in some patients, two implants were used to support restorations with three or four dental units. In many cases, a distinct pattern of bone loss was observed soon after loading that led to bone loss and failure of the implants (see Figure 82-16). Additional implants significantly improved the biomechanics of these implant-supported fixed partial dentures.
Historically, the use of longer (>7 mm) implants has been advocated as the result of reported higher rates of failure for shorter (≤7 mm) implants.24,27,38,51 Logic suggests that biomechanical stress around “short” implants leads to greater bone loss relative to the implant length and a higher rate of implant failure. However, Pierrisnard et al47 showed that the maximum implant stress somewhat increased with implant length and bicortical anchorage. They also stated that the low anchorage stiffness of shorter implants might reduce the mechanical stress to the implant because of the flexibility of the bone. Using the finite element analysis (FEA), Pierrisnard and colleagues46 showed that greater implant length did not positively affect transfer of stresses to the implant but that increasing implant diameter did reduce the intensity of stress along the length of the implant. Other studies also observed that wider rather than longer implants registered lower stress values to the whole system, suggesting that the use of short, wide implants could increase the load-bearing capacity of implants and implant prostheses7,26,45 (Box 74-2). In a recent systematic review of the literature, Atieh et al concluded that the survival of short (≤8.5 mm) implants used in the posterior partially edentulous jaw is high and not related to implant surface, design or width.4
Angulation and Arrangement
The angulation and arrangement of implants used to retain and support a prosthesis influence the load-bearing capacity of the system (i.e., implants, components, prosthesis, and supporting bone). In the 1980s, conventional dogma stated that once an implant became “osseointegrated,” it did not matter whether occlusal loads were applied axially. However, as more clinical follow-up and animal research data became available, the adverse effect of nonaxial loads on implants became increasingly more apparent. The angulation of implants in relation to the plane of occlusion and the direction of the occlusal load is an important factor in optimizing the transfer of occlusal forces to implants and surrounding bone. Axial loads are tolerated well. Minor discrepancies in angulation are probably not clinically significant, but if loads are applied at an angle of 20 degrees or more to the long axis of the implant, load magnification can result, provoking a resorptive remodeling response of the adjacent bone. This concept is supported by numerous FEA studies, which clearly demonstrate that nonaxial forces significantly increase the stress concentration to the cortical bone around the neck of the implant (Figure 74-1).3,14,42,56 Nonaxial loads lead to implant overload through load magnification at the bone-to-implant interface, which, in turn, precipitates a resorptive remodeling response of the bone around the neck of the implants.
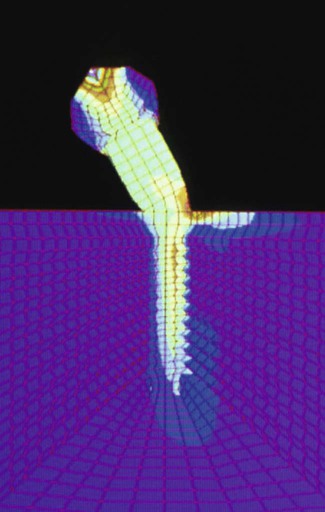
Using FEA, Cho and coworkers demonstrated that nonaxial loads concentrated stresses around the neck of the implant. (Courtesy Dr. In Ho Cho.)
When excessive loads persist, bone loss continues and may progress to implant failure. Brunski et al13 proposed that excessive occlusal loads lead to microdamage (fractures, cracks, delamination) of the bone adjacent to the implant, which provokes a resorptive remodeling response. The bone remodeling at the implant surface results in less bone density adjacent to the implant, especially around the coronal aspect of the implant. This bone has poor capacity for repair that is the result in part to the absence of a periodontal ligament fiber attachment (i.e., no bone-forming tension exists). Thus a vicious cycle ensues in which continued excessive loading leads to more microdamage and progressive bone loss until the implant fails (Box 74-3).
Before the widespread use of bone augmentation procedures in the posterior maxilla (i.e., sinus augmentation), many implants were positioned with excessive buccal angulations or were restored with buccal and/or distal cantilevers. Implant-supported restorations with a cantilevered pontic transfer nonaxial occlusal forces to the adjacent implant and surrounding bone resulting in bone loss. This load magnification causes bone loss around the neck of the implant closest to the cantilever (Figure 74-2). Excessive distal angulations, which creates nonaxial loading is another recognized problem that leads to bone loss. These implants often exhibit signs of overload (i.e., progressive and irreversible bone loss around neck of implant) when nonaxial occlusal loads are applied to the prosthesis (Figure 74-3).
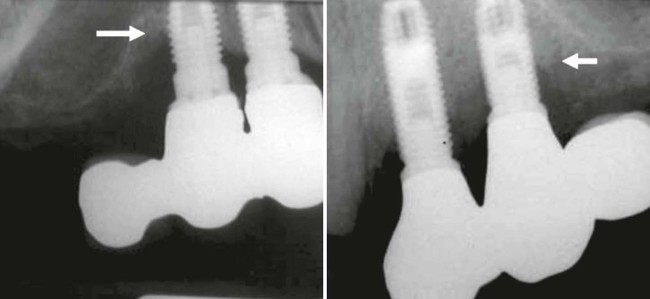
These radiographs demonstrate two cases of bone loss (arrows) around the neck of short implants (closest to the cantilever) in which load magnification occurs.
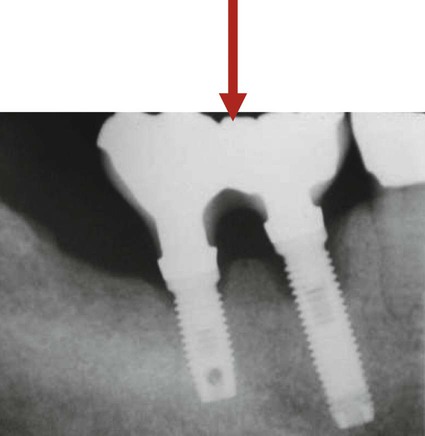
The bone appositional index (percentage of bone-to-implant contact) may be the most important factor to consider when evaluating the load-bearing capacity of any given implant(s). Less bone density and lower bone-to-implant contact provide less support and resistance to occlusal loading. As an example, consider the bone support of implants in the posterior maxilla; the bone quality is particularly poor as compared with the anterior mandible. The trabecular bone is less dense and the cortical bone layer is thin in the posterior maxilla. As a result, the bone appositional index in the posterior maxilla is significantly less than what can be achieved in the anterior mandible, where the trabecular bone is typically dense with a thick cortical bone layer. The bone appositional index for implants in the posterior maxilla typically ranges from 30% to 60%, whereas the bone appositional index for implants placed in the anterior mandible typically ranges from 65% to 90% (Figure 74-4).
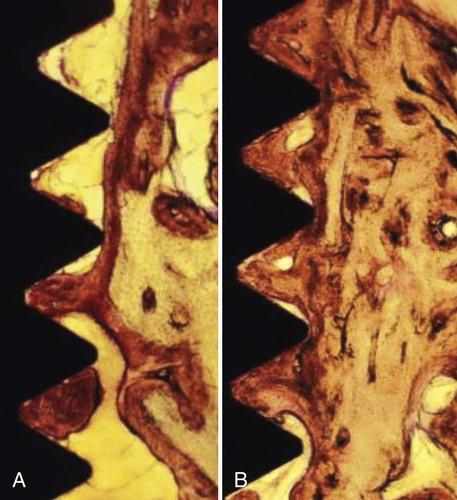
In these histologic images obtained with light microscopy, note the difference in bone appositional index achieved in sparse “poor-quality” bone (A), often found in the posterior maxilla, versus dense bone (B), typical of the anterior mandible.
Technologic advances in surface modification (as compared to the original machined Brånemark surface) may enhance bone apposition and support for implants placed in poor quality/quantity bone, thereby gaining anchorage and improving the biomechanics.5,32,33,54 These “rougher” implant surfaces appear to have a significant effect on bone anchorage.32,33,43 Surfaces with an altered microtopography achieve higher bone appositional indices than the machined surfaces and appear to facilitate the biologic processes of bone formation through greater deposition of bone onto the surface of the implant. In addition, bone deposited on the surface of acid-etched implants appears to be harder and denser and may be more resistant to resorptive remodeling.52
A lack of bone height limits the amount of available bone for placement of implants and reduces the potential for bone-to-implant contact. In the posterior mandible the inferior alveolar nerve and vessels travel through the body of the mandible until exiting the mental foramen in the premolar region (see Chapter 54). The height of bone available for placement of implants in the posterior mandible is determined by the location of the neurovascular bundle and the resorption of the alveolar ridge. Lateral nerve repositioning is possible but has a moderately high morbidity rate. In the posterior maxilla, pneumatization of the maxillary sinus combined with loss of alveolar bone results in a decreased total height of available bone in the posterior maxilla, which limits the length of implants that can be used. Sinus floor elevation and bone augmentation procedures have enabled clinicians to increase the height of bone available in the posterior maxilla, allowing for the placement of longer implants with improved success (see Chapter 77). Vertical ridge augmentation is challenging and less predictable for all sites.
Treatment Planning With Dental Implants
The success of implant-retained restorations is measured not only by successful osseointegration but also by the lack of problems associated them in function over time. Implant complications and failures have taught clinicians that the “engineering” of implant support for prosthetic appliances is an essential part of treatment planning and the key to success for implant-retained restorations (see Chapter 82 for a description of implant complications and failures).
Edentulous Maxilla
1. Poor ridge form with a marginally stable conventional maxillary denture. Two or four implants provide greater stability and security of a maxillary denture in function when the maxillary ridge is severely resorbed and lacks resistance to lateral forces.
2. Lack of posterior support with an intact mandibular anterior dentition. Implants in the maxilla can offset the potentially destructive effects on the premaxillary region when a mandible with natural anterior teeth and missing posterior teeth opposes an edentulous maxilla. In this situation, the lack of posterior support leads to a condition often referred to as combination syndrome in which overclosure of the anterior teeth causes a “hammer-and-anvil” type of destruction of the edentulous anterior maxilla (Figure 74-5).
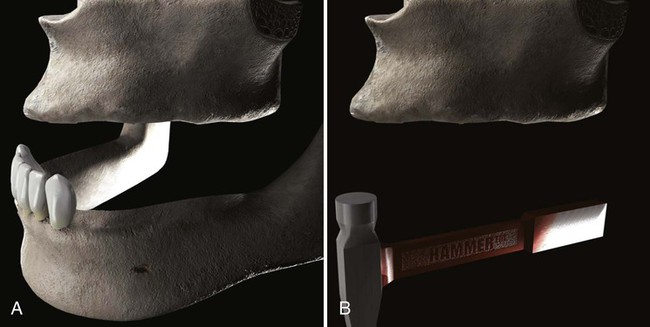
3. Palatal coverage is not tolerated. Some patients prefer a palateless denture, which may enhance their sensations of taste and texture or may simply provide a psychologic advantage. Some patients prefer a palateless denture because the proximity of the denture with the soft palate induces/>
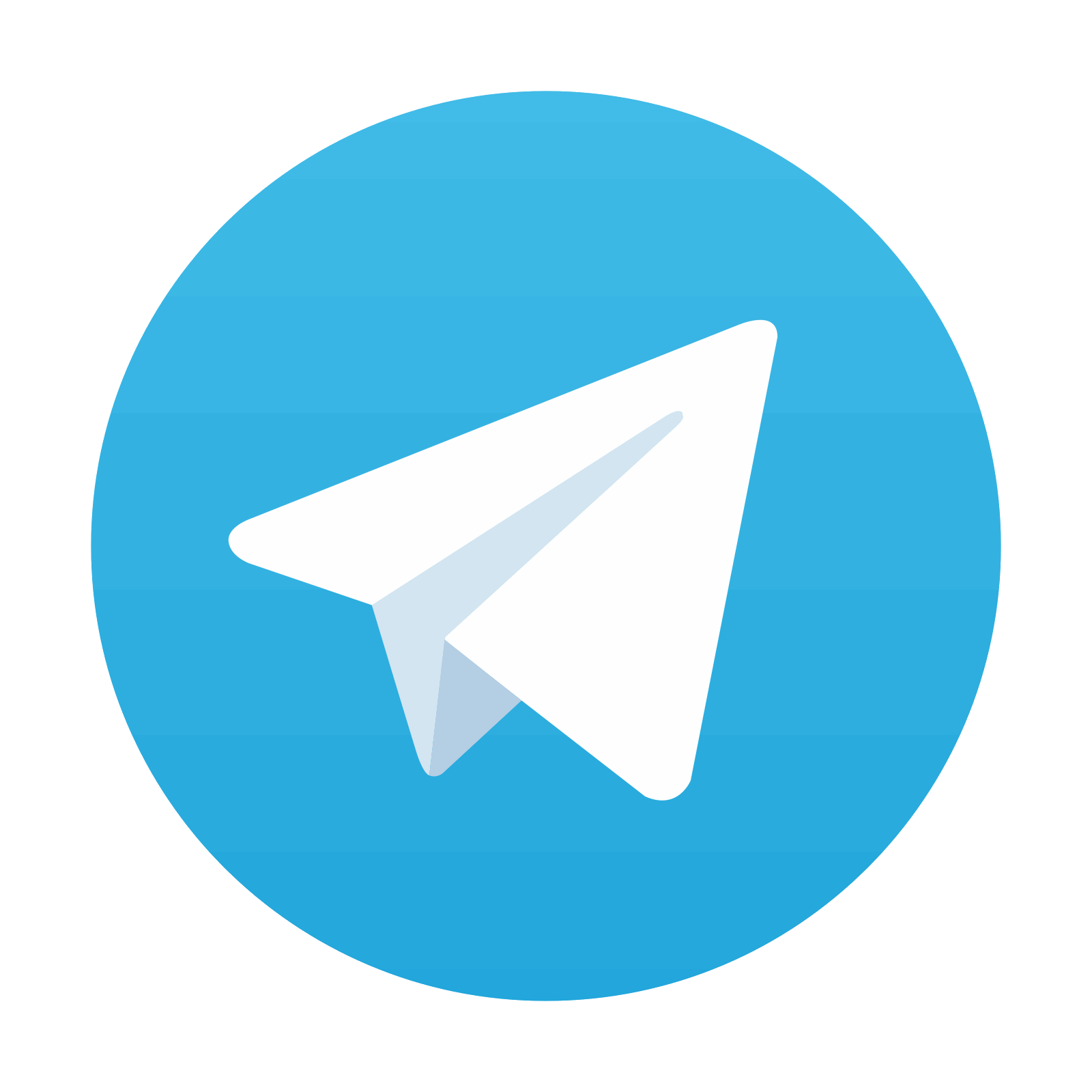
Stay updated, free dental videos. Join our Telegram channel

VIDEdental - Online dental courses
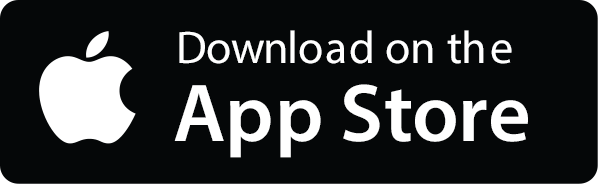
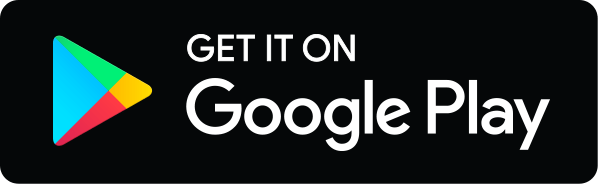