Periimplant Anatomy, Biology, and Function
Joseph Fiorellini, Keisuke Wada, Panagiota Stathopoulou and Perry R. Klokkevold
Attempts to replace lost dentition by means of implanted materials can be traced back to the ancient Egyptians, who hammered shaped seashells directly into the jaws for the purpose of replacing teeth.41 Over the last few centuries, a variety of materials have been implanted into jaws in an attempt replace missing teeth. The success of these early implants was extremely poor primarily because they never achieved a stable state of integration with the supporting tissues.89 The typical outcome, regardless of material or design, was healing with a soft tissue layer interposed between the implant and bone (i.e., fibrous encapsulation). Consequently, implants became mobile, infected, and painful, which led to failure.
The history of modern implant dentistry began with the introduction of titanium implants.41 In the 1950s, Per-Ingvar Brånemark, a Swedish professor of anatomy, had a serendipitous finding while studying blood circulation in bone that became a historical breakthrough in medicine. He discovered an intimate bone-to-implant apposition with titanium that offered sufficient strength to cope with load transfer. He called the phenomenon “osseointegration” and developed an implant system with a specific protocol to predictably achieve it. The implants were used to anchor prosthetic replacement teeth in the edentulous jaw,30 and the first patient was successfully treated in 1965.29,70 Subsequent clinical studies proved that commercially pure (CP) titanium implants, placed with a strict protocol, including an unloaded healing period, could predictably achieve osseointegration and retain a full-arch prosthesis in function with long-term success (15 years).9 Since that time, millions of patients worldwide have had missing teeth replaced with implants based on this original concept. Over the years, implant systems with variations in design (geometric and surface characteristics) and modified protocols have been developed and used with equal or better long-term success.
Implant Geometry (Macrodesign)
Numerous implant systems with various geometric (macrodesign) designs have been developed and used before the current implant systems in use today. Previous implant designs included blade vents (narrow, flat shape; tapped into boney trough prepared with rotary burs),67 press-fit cylindrical (bullet shape; pressed or tapped into prepared hole),98 subperiosteal (custom-made framework; adapted to the surface of jawbone),40 and transmandibular (long rods or posts; placed through the anterior mandible).103 Some of these implant systems were initially stable and appeared to be successful over short-term periods (e.g., 5 years) but failed to remain stable, became symptomatic and/or loose, and failed over longer periods.96,120 Lacking predictability, these implant systems are no longer used.
Since the time of the Brånemark studies, millions of patients have been treated worldwide using variations of these techniques with implants of different geometries and surface characteristics. Similar research including that of André Schroeder in Switzerland in the mid-1970s contributed to the success of endosseous dental implants. The serendipitous finding of Brånemark was that when a hole is prepared into bone without overheating or otherwise traumatizing the tissues, an inserted biocompatible implantable device would predictably achieve an intimate bone apposition, as long as micromovements at the interface were prevented during the early healing period. The history of the research endeavors in Sweden provides a better understanding of the relevant biologic parameters involved.70
The macroscopic configuration of implants has varied widely; the most common types are listed in Box 71-1. Currently, most endosseous implants have a cylindrical or tapered, screw-shaped/threaded design. The disastrous results with other implant configurations were largely responsible for the evolution toward the current popular designs.14
Endosseous Implants
Blade Implants.
Blade implants were designed and developed by Linkow67 and used clinically in 1960s and 1970s. Blade implants were inserted into the jawbone after mucoperiosteal flap elevation and preparation of a channel with a high-speed rotary bur. They were tapped into the narrow trench. One or several posts pierced through the mucoperiosteum after suturing of the flaps. After a few weeks of healing, a fixed prosthesis was fabricated by a classic method and cemented on top of it.
Because the high-speed drilling leads to extensive bone necrosis at the histologic level, fibrous scar tissue formation occurs. This allows downgrowth of the epithelium, which leads to marsupialization of the blade implants (Figure 71-1).57 If a bacterial infection occurs, it can lead to an intractable periimplantitis with ample bone loss. More importantly, removal of such implants after complications implies sacrificing surrounding jawbone. Because of its retentive geometry, the blade implant cannot simply be extracted or removed by a trephine, as with a cylindrical or screw-shaped implant.
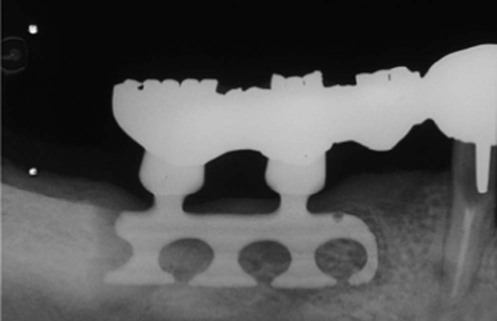
The interface between blade vent implant and bone was called fibroosseous integration, which was defined as tissue-to-implant contact: interposition of healthy dense collagen fibers at the interface between the implant and bone.1 At that time, the fibrous tissue was thought to be the replacement of the periodontal ligament;115 however, it was fibrous scar tissue following the bone necrosis initiated by high-speed drilling. This allows epithelial downgrowth around the post caused by the bacterial infection, which leads to encapsulation of blade implant with the fibrous scar tissue.
Most of the studies reported less than 50% success rate for 5-year duration with the complication of pocket formation exceeding 6 mm, followed by the significant alveolar bone loss around the implant.36,106 It was also more complicated and invasive surgery to remove the failed implant, which was sacrificing significant surrounding jawbone. As a result of the poor long-term success, as well as the high complication rate, the use of blade vent implants has been significantly reduced. Removal of failed blade implants often requires significant cutting and bone removal despite being mobile.
Disk Implants.
Disk implants are rarely used at present. The concept developed by Scortecci is based on the lateral introduction into the jawbone of a pin with a disk on top.100 Once introduced into the bone, the implant has strong retention against vertical extraction forces. Implants have been used with one, two, and even three disks. Unfortunately, as mentioned previously for blade implants, the cutting of the bone by means of high-speed drills leads to a fibrous scar tissue surrounding the implant, as revealed frequently by periimplant radiolucencies. Data on the clinical success of disk implants are mostly anecdotal.
Root Form (Cylindrical) Implants
The first implant in this category, designed and developed by Schroeder and colleagues between 1974 and 1985, was called ITI (International Team for Implantology) hollow-cylinder, plasma-sprayed, one-stage implant98 (Figure 71-2). It was thought that the hollow geometry should provide large bone-to-implant contact and holes would favorable for the additional fixation of the implant. However, the survival rates were less favorable compared to the other system.8 Thus this system was withdrawn from clinical use.
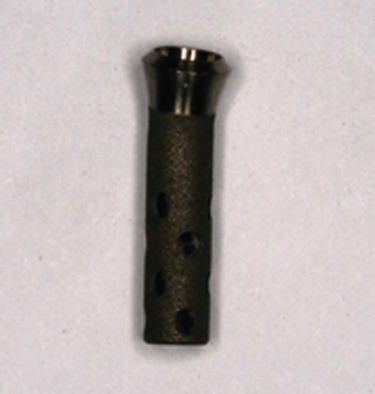
When discussing cylindrical implants, it is important to distinguish between hollow and full cylindrical implants. Straumann and coworkers introduced hollow cylinders in the mid-1970s with the ITI system.107 The idea was that implant stability would benefit from the large bone-to-implant surface provided by means of the hollow geometry. It was also thought that the holes (vents) would favor the ingrowth of bone to offer additional fixation. The same concept was used in the Core-Vent system developed by Niznick.78 Although it was not clarified whether the cause was geometry or the associated surface characteristics (titanium plasma–sprayed surface, titanium alloy), survival statistics were disappointing for hollow cylinder implants.111
Solid cylindrical implants were used by Kirsch and became available under the name IMZ, referring to the internal mobile shock absorber.63 The IMZ system, developed by Kirsch, prevailed in the market (Figure 71-3). The characteristic function of this system was the internal mobile element (IME) shock absorber, which was able to connect with the adjacent natural tooth. The system presented successful results in the short term, but long-term success rates were unacceptable (38% in 10 years), leading to the limited use of this implant design.49 For this reason, this implant is rarely seen in the market.
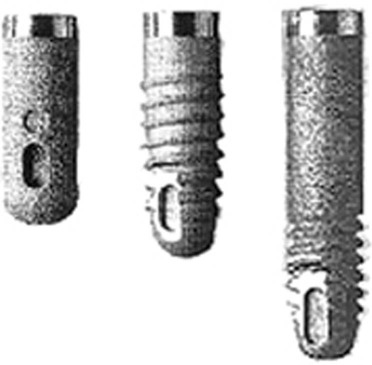
Even when an intimate bone apposition is achieved, extraction forces on such cylindrical implants lead to strong shear forces at the bone-to-implant interface. Only the microscopic surface irregularities offer some mechanical retention by interdigitation of bone growing onto the implant surface. With a screw-based geometry, forces acting parallel to the long axis of the implants are dispersed in many directions.102
Transmandibular Implants
Transmandibular implants were developed to retain dentures in the edentulous lower jaw. They were indicated for use in the extremely resorbed edentulous mandible with a minimal alveolar ridge height less than 10 mm. The implant was inserted through a submandibular skin incision and required general anesthesia. Two models were available in the 1960s. The first, called the staple-bone implant, was developed by Small. It consisted of a splint adapted to the lower border of the mandible, to which it is fixed by stabilizing pins.104 Two transmandibular screws were driven transgingivally into the mouth. The reported implant survival rate was 93% after 5 years and continued to exceed 90% after 15 years.103,105 The other model, introduced by Bosker, has two metal splints, one below the lower border of the mandible and one intraorally to connect the four posts piercing through the soft tissues.24 The Bosker implant seemed less reliable than the staple-bone implant, achieving only 70% survival after 5 years in the mandibular symphyseal area.111 Both transmandibular implants presented with the gingival hyperplasia or infection with the incidence of 10% to 15% of all cases.103 Despite the good long-term survival data reported (especially for the staple implant), the high incidence of complications and the necessity of using general anesthesia for implant placement, the transmandibular implant design is rarely used today.
Subperiosteal Implants
Subperiosteal implants are customized according to a plaster model derived from an impression of the exposed jawbone, before the surgery planned for implant insertion. The implant was designed with several posts, typically four or more for an edentulous jaw, which passed through the gingival tissues.12
The subperiosteal implant was used in the treatment of atrophic mandibles. The implant is custom designed to fit the mandibular jaw bone using a plaster model obtained from an impression of the exposed mandible. It consisted of a cast metal framework with a subperiosteal component in contact with the bone and a trans-gingival component (posts), which were used to retain the dental prosthesis. The number of transgingival posts on the implants depend on the number of missing teeth, averaging four to six for the totally edentulous jaw. Several clinical studies reported poor survival rates of less than 50% for 5 to 10 years, with the frequent complication of gingival inflammation, involving tissue reaction around the post caused by the lack of soft tissue attachment to the metal post. These complications led to the development of sinus tract resorption of the cortical bone and exposure of framework.79,121 As a result of this higher rate of failure, as well as the significant complications and surgical invasion, subperiosteal implants are rarely used.
The most common implant design being used today is the screw-shaped or threaded cylindrical implant (Figure 71-4, A). A threaded implant design is preferred because it engages bone well and is able to achieve good primary stabilization. Even systems that started with cylindrical press-fit (nonthreaded) designs progressively evolved to a threaded geometry. The (longitudinal) shape of implants may be parallel or tapered (Figure 71-4, B). Although a vast majority of all implants have been parallel walled, the use of a tapered implant design has recently been advocated because it requires less space in the apical region (i.e., better for placement between roots or in narrow anatomic areas with labial concavities). Tapered implants have also been advocated for use in extraction sockets (Figure 71-5).
Implant Surface Characteristics (Microdesign)
Implant surface characteristics (microtopography) have been shown to positively influence the healing process.31,61,74,85 Accordingly, modification of implant surface characteristics has been a major area of research interest and development over the past 15 to 20 years. Modifications in surface energy, chemical composition, and surface topography are known to influence cellular activity and tissue responses leading to enhanced osteogenesis.26,32,72,114 At the molecular level, modified implant surfaces increase adsorption of serum proteins, mineral ions, and cytokines, which subsequently promote cellular migration and attachment.62,85,100 Implant surface characteristics can also aid in the retention of a fibrin clot, thus providing a migratory pathway for the differentiating osteogenic cells to reach the implant surface.37,38,87 Today, implants are treated with a variety of technologies to modify surface characteristics (microscale or nanoscale) to enhance bone formation.
Additive Processes
The additive process modifies the microstructure/macrostructure and chemical nature of the implant surface by adding materials or chemicals to the existing surface. There are several methods used to add materials and/or chemicals to the implant surface, such as inorganic mineral coatings, plasma spraying, biocoating with growth factors, fluoride, and particulates or cements containing calcium phosphates, sulfates, or carbonates. The addition of materials, such as hydroxyapatite (Figure 71-6), to the implant surface has been shown to enhance or accelerate the initial bone cells adaptation or proliferation.42,43,53,118 In general, additive surface modifications tend to increase the surface texture greater than subtractive surface modifications, which results in topographically “rougher” implant surfaces (Figure 71-7). Surface roughness can also be increased by oxidizing or adding an oxide layer (Figure 71-8).
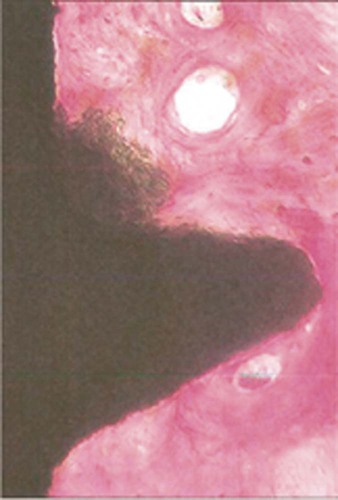
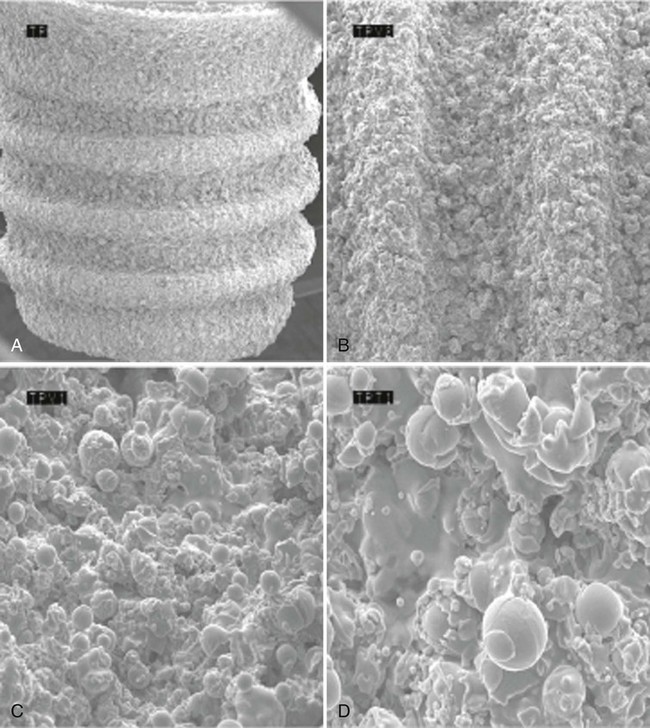
Subtractive Processes
The subtractive process modifies the microstructure and chemical nature of the implant surface by removing or altering the existing surface. The roughness of implant surface can be modified by machining, acid etching, blasting, or a combination of these processes to enhance the amount or speed of osseointegration.36,43,90 The changes are most notable at the microscopic level (Figures 71-9 and 71-10). Implant surfaces that are modified at the microscopic level with techniques such as acid-etching are thought to promote favorable cellular responses and increased bone formation in close proximity to the surface16,55 (Figure 71-11).
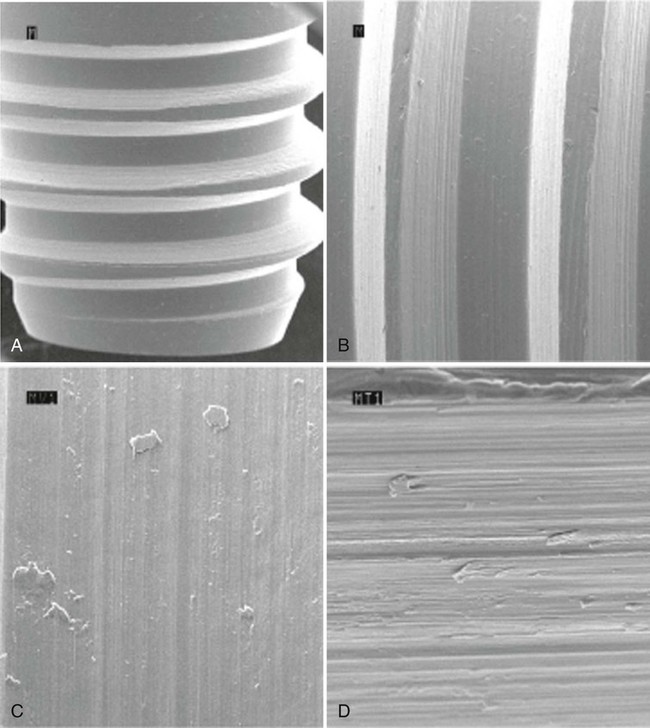
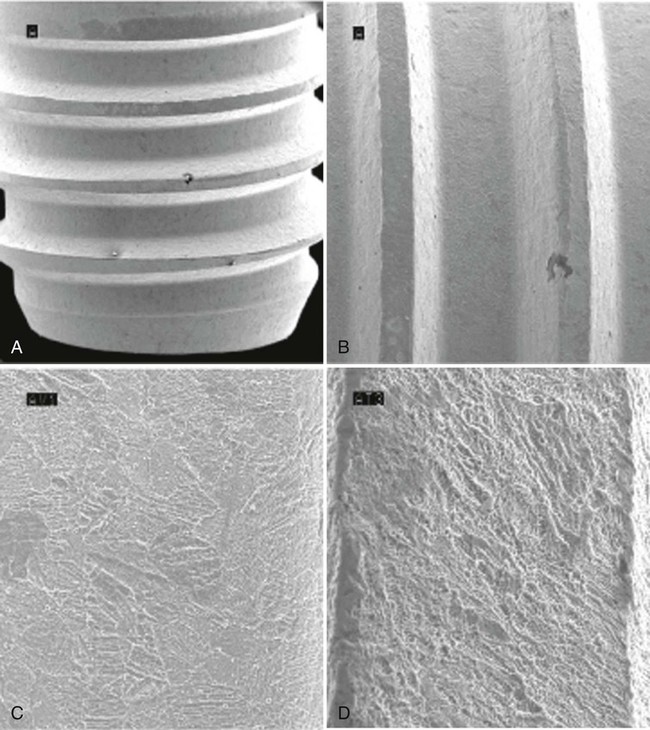
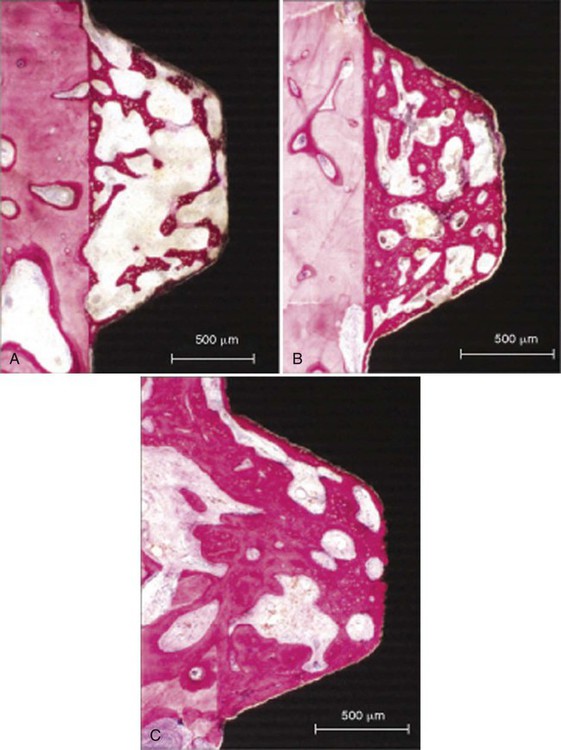
Implant Surface Chemical Composition
All titanium oxides have dielectric constants, which are higher than most other metal oxides. This factor may explain titanium’s tendency to adsorb biomolecules, as seen during surgery when the blood creeps up the surface during implant insertion. The biomolecules normally appear as folded-up structures to hide their insoluble parts, while putting water-soluble radicals on their surface. Thus they will adhere to the TiO2 surface after displacing the original water molecules sitting on its surface. Although initially attracted by weak van der Waals forces, the high dielectric constant of titanium oxides and the polarizability of the molecules after adsorption will lead to high bond strengths, which are considered irreversible when they surpass 30 kcal/mol.59 In fact, because of its propensity for being covered by an uninterrupted oxide layer, which has ceramic-like properties similar to other metal oxides (e.g., aluminum oxides), titanium makes the coating of implants superfluous. This should be stressed because many authors hope for even better osseointegration potential with calcium phosphate (CaP)–coated surfaces and strongly advocate their use. To date, clinical results with CaP-coated implants have not been encouraging in a long-term perspective.22
Thus the overall view of potential advantages for different implant surface characteristics is complex, and only clinical observations can determine their validity. For good-quality bone, after 15 years of follow-up, clinical success rates of 99% have been reported for implants with a turned surface.65 Enhanced implant surface characteristics are likely to be most beneficial for the more challenging situations, such as poor quality bone and early and immediate loading.
Implant Surface Free Energy and Micro-Roughness
When an implant is brought into contact with bodily tissues and fluids, in this case mostly bone, it faces a “bioliquid,” an aqueous environment. Within milliseconds, water, ions, and small biomolecules are absorbed. One could imagine that this absorbed layer renders all surfaces equal. However, the large molecules and the cells that will subsequently adhere to this surface are influenced by the surface characteristics of this pellicle layer. The composition and structure of the initial layer are largely determined by the underlying surface.93 Thus, the three-dimensional shape of the molecules will be modified during their adherence to this pellicle layer and will unveil different radicals, depending on this metamorphosis.
Surface topography at the cellular and molecular level means microscopic roughness. A surface roughness can be measured with a profilometer, a stylus that follows the surface and measures the peak-to-valley dimensions (expressed as Ra values) or the spacing between irregularities (expressed as Scx values). Wennerberg and Albrektsson116 provide guideline/>
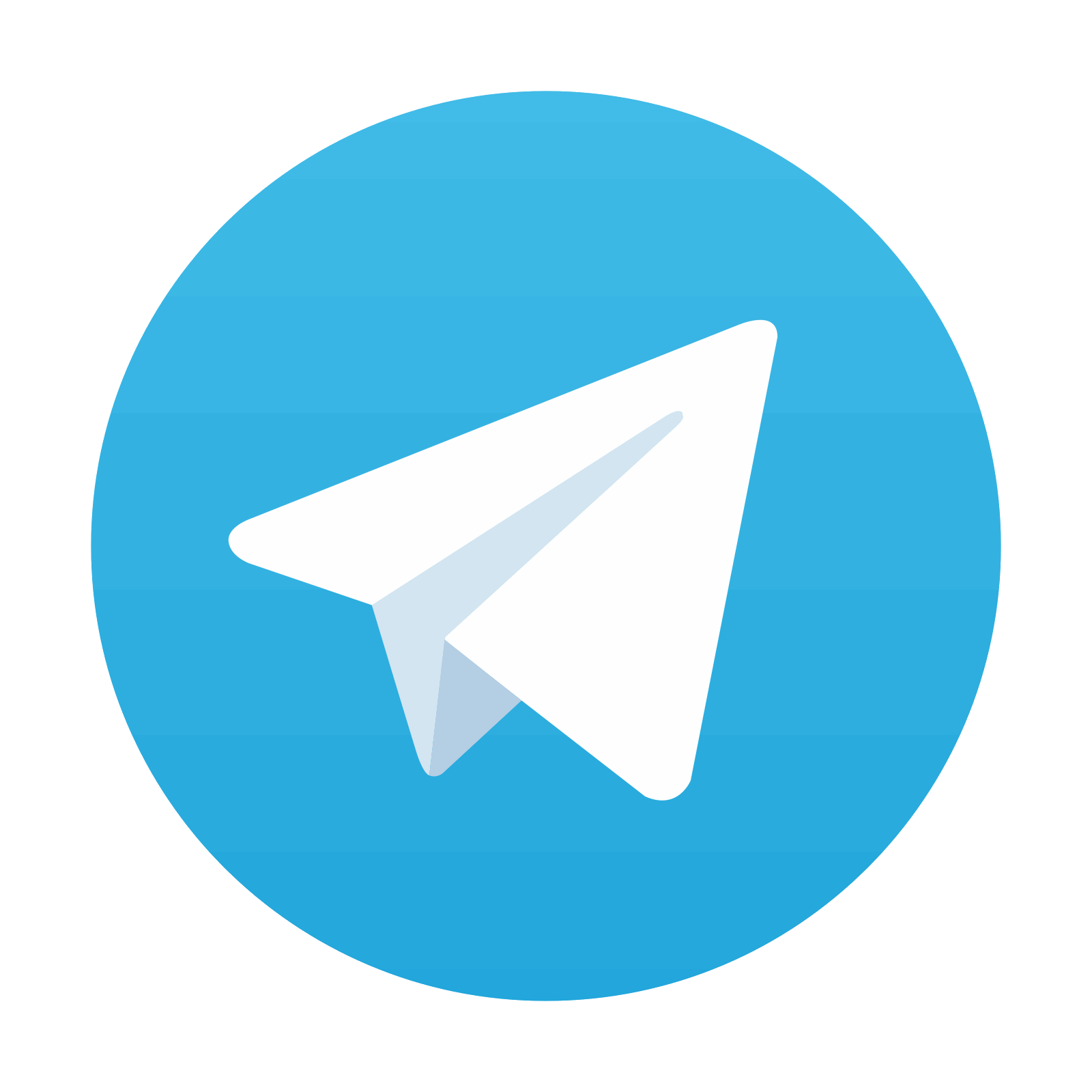
Stay updated, free dental videos. Join our Telegram channel

VIDEdental - Online dental courses
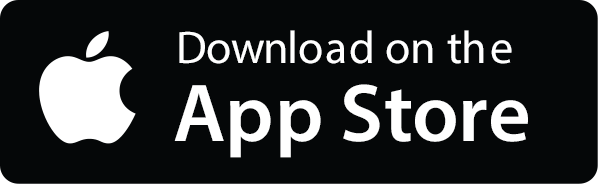
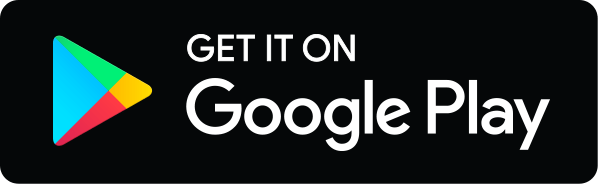