Chapter 7
Regenerative Osseous Surgery: The Use of Growth Factor–Enhanced Bone Grafts
Growth factors are proteins secreted by cells that act on the appropriate target cell or mediator cells to carry out a specific action. Their function involves a vast cellular communications network that influences such critical processes as cell division, matrix synthesis, and tissue differentiation. The results of experimental studies have established that growth factors play an important role in bone and cartilage formation, osseous healing, and regeneration. With the advent of recombinant proteins, there has been considerable interest in the use of growth factors as therapeutic agents in the treatment of skeletal injuries and in augmentation techniques. As growth factors become available as therapeutic agents, it is essential for surgeons to understand their biological characteristics and clinical potential.
BIOLOGY BEHIND GROWTH FACTOR ACTION
Growth Factors and their specific Profile of Action
Growth factors can cause a cellular response in three different ways: autocrine, paracrine and endocrine.
1. Autocrine: A growth factor acts on the same cell that produced it or on cells of the same phenotype (e.g., a growth factor produced by an osteoblast influences the activity of another osteoblast).
2. Paracrine: A growth factor acts on an adjacent or neighboring cell that is different from its cell of origin (e.g., a growth factor produced by an osteoblast stimulates differentiation of an undifferentiated nonrelated cell).
3. Endocrine: A growth factor influences a cell that is different from its cell of origin and located at a remote anatomical site (e.g., a growth factor produced by neural tissue in the central nervous system stimulates osteoblast activity).
Thus growth factors might have effects on multiple cell types and induce an array of cellular functions in a variety of tissues (Lieberman, Daluiski, and Einhorn 2002; Barnes et al. 1999).
Mechanisms Behind Cellular Responses Induced by Growth Factors
Growth factors act through binding to specific receptors on the target cell. This binding is referred to as ligand-receptor interaction. Once a growth factor binds to a target cell receptor, this receptor is activated by a change in its conformation. Receptors usually have an extracellular domain that bind to the ligand and an intracellular domain that activates the signal transduction system. Generally, activation of the transduction system results in modulation of transcription factors, which are intracellular proteins that are part of the overall signaling pathway. The activated transcription factor travels to the cell nucleus where it binds to nuclear DNA and induces transcription of specific mRNA. This results in expression of a new gene or set of genes. It is the expression of these new genes that ultimately changes the characteristics of a particular cell (Lieberman, Daluiski, and Einhorn 2002).
GROWTH FACTORS INVOLVED IN BONE METABOLISM
Signaling molecules, which are important during bone healing, can be categorized into three groups:
1. proinflammatory cytokines such as interleukin-1 (IL-1), interleukin-6 (IL-6), and tumor necrosis factor-α (TNF-α);
2. transforming growth factor (TGF-β) superfamily, especially bone morphogenetic proteins (BMPs), platelet-derived growth factor (PDGF), fibroblast growth factor (FGF), and insulin-like growth factor (IGF); and
3. angiogenic factors such as vascular endothelial growth factor (VEGF), angiopoietins, and matrix metalloproteinases (MMPs) that degrade bone and cartilage and, therefore, enable vessel invasion (Dimitriou, Tsiridis, and Giannoudis 2005).
Cytokines IL-1, IL-6, and TNF-α are involved early in the repair cascade. These cytokines are secreted by macrophages and mesenchymal cells present in the periosteum and respond to injury with a peak in expression during the first 24 hours. However, they are also active in the remodeling phase of healing. In addition, these cytokines exert chemotactic activity on inflammatory cells, enhance cellular matrix synthesis, and stimulate angiogenesis (Dimitriou, Tsiridis, and 2005; Kon et al. 2001).
TGF-β is produced by osteoblasts. It stimulates expression of bone matrix proteins and suppresses the degrading activity of matrix metalloproteinases and other enzymes. TGF-β also induces proliferation or differentiation of osteoblastic cells while inhibiting the formation of osteoclast precursors and, in greater concentrations, may exert an inhibitory effect on mature osteoclasts. A subgroup of the TGF-β superfamily, called BMPs, are involved in cell growth, migration, and differentiation and play a regulatory role in tissue homeostasis and repair in adult organisms (Overall, Wrana and Sodek 1991; Wrana et al. 1988; Kingsley 1994).
PDGF is a potent mitogen for mesenchymal cells. Platelets, monocytes, macrophages, endothelial cells, and osteoblasts produce this growth factor. In the early stages of bone healing, PDGF is a powerful chemotactic agent for inflammatory cells and a stimulus for osteoblasts and macrophages. Another growth factor involved in bone healing is FGF, which is produced by monocytes, macrophages, mesenchymal cells, chondrocytes, and osteoblasts. FGF is important in chondrogenesis and bone resorption. The target cells are mesenchymal and epithelial cells as well as chondrocytes and osteoblasts (Dimitriou, Tsiridis, and Giannoudis 2005; Hollinger et al. 2008).
The role of IGF in bone formation has been disputed. Sources of IGF are bone matrix, endothelial cells, osteoblasts, and chondrocytes. There are two isoforms, IGF-1 and IGF-2. IGF-1 is the more potent and involved in bone matrix formation (Lieberman, Daluiski, and Einhorn 2002; Barnes et al. 1999; Fowlkes et al. 2006).
In the late phases of healing and bone remodeling, cartilage and bone are degraded by MMPs. This allows angiogenic factors to regulate vessels in growth by either the VEGF-dependent pathway or the angiopoietin-dependent pathway. VEGF is found in four isoforms (A, B, C, and D). Several cells, including macrophages, smooth muscle cells, and osteoblasts, produce the protein (Gerstenfeld et al. 2003). See Table 7.1 for growth factor function in the bone and cell.
Table 7.1. Effect of growth factors on bone formation and remodeling.
Growth Factor | Cell Source | Function in Bone |
TGF-β | Platelets, osteoblasts, bone marrow stromal cells, chondrocytes, endothelial cells, fibroblasts, macrophages | 1. Undifferentiated mesenchymal cell proliferation. 2. Osteoblast precursor recruiting. 3. Osteoblast and chondrocyte differentiation. 4. Bone matrix production. 5. Recruitment of osteoclast precursors. |
BMP | Osteoproginator cells, osteoblasts, chondrocytes, endothelial cells | 1. Differentiation of mesenchymal cells into chondrocytes and osteoblasts. 2. Migration and differentiation of osteoprogenitors into osteoblasts. 3. Influences skeletal pattern formation. 4. Induction of matrix synthesis. |
PDGF | Platelets, osteoblasts, endothelial cells, monocytes, macrophages | 1. Osteoprogenator migration 2. Proliferation and differentiation. |
IGF | Osteoblasts, chondrocytes, hepatocytes, endothelial cells | 1. Osteoprogenator proliferation and differentiation. 2. Osteoblast proliferation. 3. Bone matrix synthesis. 4. Bone resorption. |
FGF | Macrophages, monocytes, bone marrow stromal cells, chondrocytes, osteoblasts, endothelial cells | 1. Chondrocyte maturation. 2. Osteoblast proliferation and differentiation 3. Inhibition of apoptosis of immature osteoblasts. 4. Induction of apoptosis of mature osteocytes. 5. Bone resorption. |
This chapter focuses on the group of growth factors that are associated with bone formation and maturation. These include TGF-β, BMPs, PDGF, FGF, and IGF.
Transforming Growth Factor
The TGF-β superfamily of growth factors contains over 30 members, and it is vital for development and homeostasis. The ligands and their downstream pathway components regulate diverse cellular functions, such as growth, adhesion, migration, apoptosis, and differentiation (Wu and Hill 2009).
The TGF-β superfamily ligands are secreted as precursors composed of prodomain and a C-terminal mature polypeptide. The prodomains are required for the formation of dimers via disulfide bonds. The ligands are activated when the prodomains are cleaved by proteases. Ligand dimers bind and activate heteromeric complexes of type I and type II receptors. Activated receptors then phosphorylate the intracellular mediators (Smads), which form complexes with each other and other proteins to modulate transcription of target genes in the nucleus (Wu and Hill 2009).
TGF-β superfamily members require two different serine/threonine kinase receptors to signal, a type I and a type II. There are seven type I receptors (ALKs 1–7) and five type II receptors in the human genome. The ligand brings the receptors together in a heterotetrameric complex in which the type II receptors phosphorylate and activate the type I receptors. In the case of the TGF-β receptors, the ligand–receptor interaction is highly cooperative. The ligand–receptor complexes assemble through the recruitment of the low-affinity type I receptor by the ligand-bound high-affinity type II receptor, facilitated by direct type I–type II interactions at the composite ligand–type II interface (Wu and Hill 2009; Groppe et al. 2008).
The most studied signaling pathway downstream of TGF-β superfamily receptors is the Smad pathway. The Smads are a group of intracellular signaling molecules comprising the receptor-regulated Smads (R-Smads) Smad1, 2, 3, 5, and 8; the co-Smad, Smad-4; and the inhibitory Smads, Smad-6 and -7. Upon ligand stimulation, the R-Smads are phosphorylated by the type I receptors and form both homomeric and heteromeric complexes with Smad-4 that accumulate in the nucleus and directly regulate the transcription of target genes. Although the Smads are the best understood signal transducers downstream of TGF-β superfamily receptors, other signaling pathways can also be activated directly in response to TGF-β, such as ERK MAP kinase (MAPK) signaling pathway, JNK and p38 MAPK signaling, and the kinase PAK2 (Kinglsey 1994; Wu and Hill 2009).
TGF-β has three isoforms, TGF-β1, -β2, and -β3. All three isoforms are detected in bone, but the TGF-β1 isoform is the most abundant at the protein level. In neonatal human bone, all isoforms can be found at sites of endochondral and intramembranous ossification, but the patterns of expression differ. At sites of endochondral bone formation, TGF-β1 and TGF-β3 are detected in proliferative and hypertrophic zone chondrocytes, and TGF-β2 is detected in all zones of the cartilage. During intramembranous bone formation, TGF-β1 and -β2 are present at sites of mineralization, whereas TGF-β3 is more widely distributed. Osteoclasts also express TGF-β, mostly TGF-β1, in high amounts. It is also important to note that expression of all three TGF-β isoforms is upregulated during fracture healing, suggesting that their roles are not restricted to embryonic bone development, but extend to adult bone remodeling (Ai-Aql et al. 2008; Janssens et al. 2005).
TGF-β1 increases bone formation mainly by recruiting osteoblast progenitors and stimulating their proliferation, thus increasing the number of committed osteoblasts, as well as by promoting the early stages of differentiation. On the other hand, it blocks later phases of differentiation and mineralization. These later stages are regulated by other growth factors such as the BMPs. TGF-β1 also blocks apoptosis of osteoblasts and promotes maintenance of survival during differentiation into osteocytes. Interestingly, recruitment of osteoclast precursors to the bone environment, differentiation to the mature osteoclast, bone resorption, and osteoclast apoptosis are also modulated by TGF-β1 either directly or indirectly through osteogenic cells (Janssens et al. 2005).
Bone Morophogenic Proteins
In 1965 Marshall R. Urist recognized the concept that there is a substance in bone that can induce new bone formation when he observed that bone had formed after the implantation of demineralized bone matrix in a muscle pouch in rat model. He termed this phenomenon the bone induction principle and later identified the protein responsible for this effect, which took on the name bone morphogenetic protein. In 1988, Wozney et al. identified the genetic sequence of BMP, which led to the identification of its various isoforms. With this genetic information, it is now possible to produce various BMPs using recombinant gene technology. (Urist 1965; Wozney et al. 1988)
BMPs are members of the TGF-β superfamily, and there are at least 30 individual molecules that have been identified so far. Of these, BMP-2 to BMP-8 show high osteogenic potential. BMP-2, -4, and -7 are known to play a critical role in bone healing by means of their ability to stimulate differentiation of mesenchymal cells to an osteochondroblastic lineage. In vitro studies show that mesenchymal stem cells exhibit a great number of BMP receptors. Mesenchymal stem cells also synthesize the BMP antagonists noggin, gremlin, follistatin, and sclerostin, which are capable of blocking osteogenesis as mesenchymal stem cells differentiate into osteoblasts. Osteoblasts secrete BMPs as well as their antagonists by a delicate regulatory mechanism during bone formation and remodeling. When BMPs are secreted from cells, they have one of several fates: they may immediately exert their actions locally; they may be bound up by extracellular antagonists present at the site of BMP secretion; or, they may interact with extracellular matrix proteins that serve to sequester or enhance BMP activity by anchoring it to make it more available to target cells (Barnes et al. 1999; Abe 2006; Rosen 2006).
BMPs use the same serine/threonine kinase receptor complex to initiate cell signaling. BMP receptors are classified as type I or type II based on sequence homology. Three type I BMP receptors have been identified, Alk3, Alk6, and Alk2, and the BMP type I receptor mainly determines the specificity of the intracellular signals. Type II receptors that exhibit BMP binding are BMP RII and Act RII and IIb. Unlike TGF-β receptors, the BMP receptor complex does not assemble cooperatively, which means that both type I and type II BMP receptors can bind BMPs in the absence of the other receptor. Also in contrast to other TGF-β family members, there is no direct connection between the extracellular domains of the individual receptors. BMP ligand binding to type I receptor triggers the intracellular association of type I and type II receptors, allowing the constitutively phosphorylated type II receptor to phosphorylate the type I receptor. Once acti/>
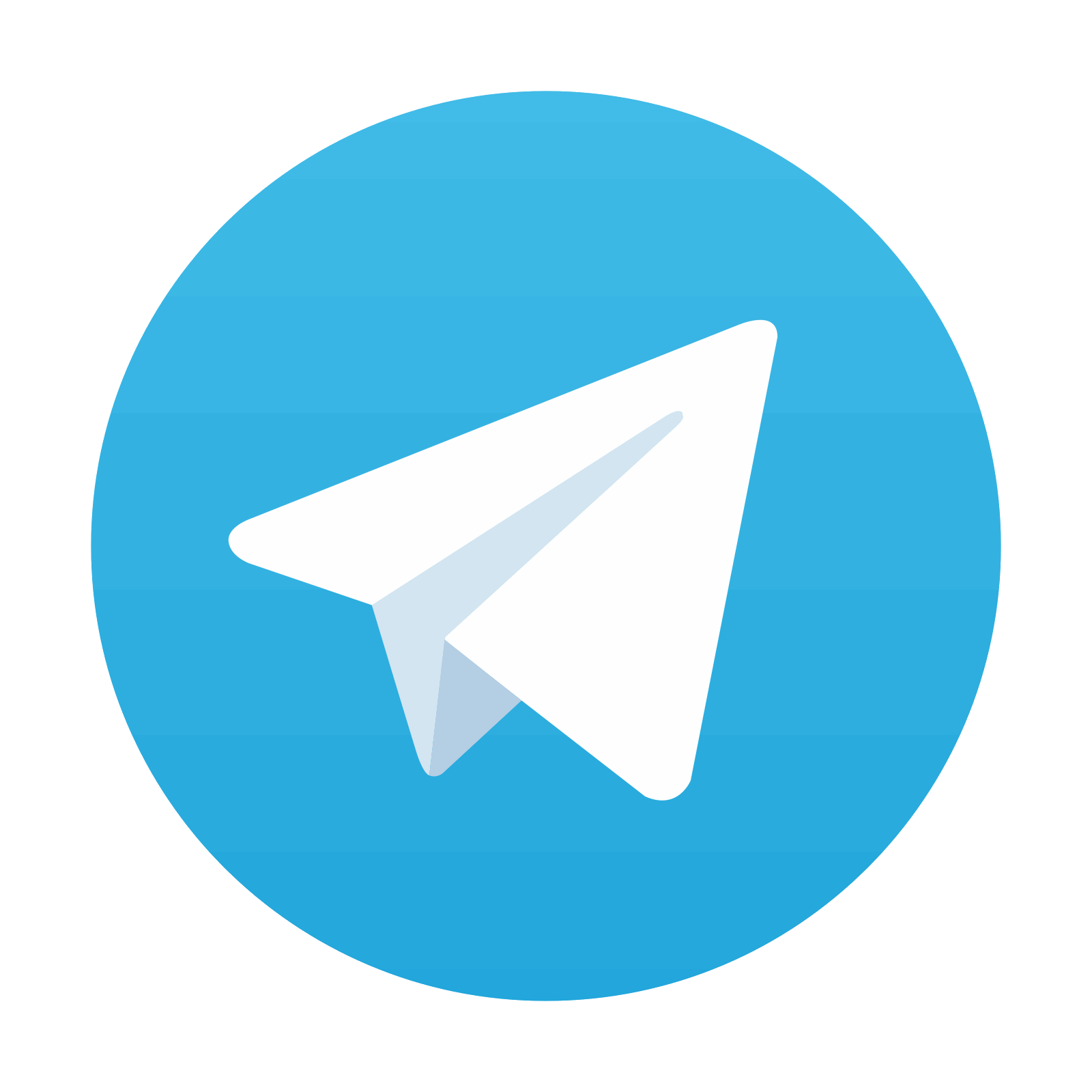
Stay updated, free dental videos. Join our Telegram channel

VIDEdental - Online dental courses
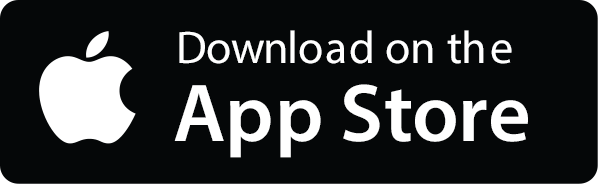
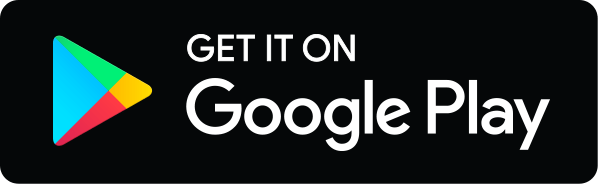