General Classes of Biomaterials
Dental biomaterials are generally categorized into four classes: metals, polymers, ceramics and composites. The four classes are distinctly different from each other in terms of density, stiffness, translucency, processing method, application, and cost. One class, composites, is a combination of two or more classes that produces materials that can be engineered for specific applications. For some applications, such as intracoronal restorations, several classes offer suitable materials. For other applications, such as endosseus implants, only one class is appropriate. Requirements such as esthetics, stiffness, and osseointegration dictate the choice of material class. Restoration design and material class are always coordinated to achieve the best patient outcome.
This chapter is organized into four sections by the class of material. Fundamental concepts of each class are presented here. Additional detail is provided in other chapters when specific applications are discussed.
Metals and Alloys
Metals and alloys are used in almost all aspects of dental practice, including the dental laboratory, direct and indirect dental restorations, implants, and instruments used to prepare teeth. Metals and alloys have optical, physical, chemical, thermal, and electrical properties that are unique among the basic types of materials and suitable for many dental applications. Although tooth-colored materials are often desired for restorations, metals provide strength, stiffness, and longevity for long-term dental applications that are often not achievable with other classes of materials. Evidence in the scientific literature of clinical performance is the most extensive for this material class.
As a class, metals are ductile and malleable and therefore exhibit elastic and plastic behavior; they are good electrical and thermal conductors, higher in density than other classes, exhibit good toughness, are opaque, and can be polished to a luster. Metals may be cast, drawn, or machined to create dental restorations and instruments.
Chemical and Atomic Structure of Metals
A metal is any element that ionizes positively in solution. As a group, metals constitute nearly two thirds of the periodic table (Figure 7-1). During ionization, metals release electrons. This ability to exist as free, positively charged, stable ions is a key factor in the behavior of metals and is responsible for many metallic properties that are important in dentistry. Another important group of elements shown in Figure 7-1 are the metalloids, including carbon, silicon, and boron. Although metalloids do not always form free positive ions, their conductive and electronic properties make them important components of many dental alloys.
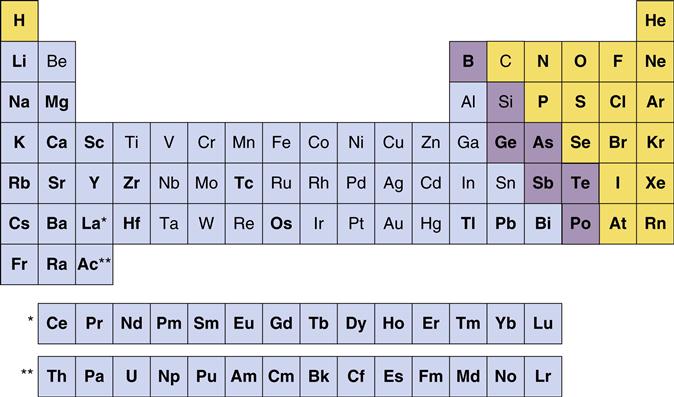
The periodic table of the elements can be subdivided into metals (blue backgrounds), metalloids (purple backgrounds), and nonmetals (yellow backgrounds). Elements in non-bolded type are used in dental alloys or as pure metals. The metals are elements that ionize positively in solution and comprise the majority of elements in the periodic table. Note that not all elements are shown. Hydrogen is often included in Group IA (left column) because it forms compounds with oxidation numbers of both +1 and −1. Also, under very high pressures, hydrogen exhibits the properties of a metal. The single asterisk indicates the insertion point in the table for the lanthanide series of elements, whereas the double asterisk indicates the insertion point for the actinide series of elements. Reference: The American Chemical Society.
Atomic Structure
At the atomic level, pure metals exist as crystalline arrays (Figure 7-2) that are continuous in three dimensions. In these arrays, the nuclei and core electrons occupy the atomic center with the ionizable electrons floating freely among the atomic positions. The mobility of the valence electrons is responsible for many properties of metals, such as electrical conductivity. It is important to note that the positively charged atomic centers are held together by the electrons and their positive charge is simultaneously neutralized by the negative electrons. Thus pure metals have no net charge.
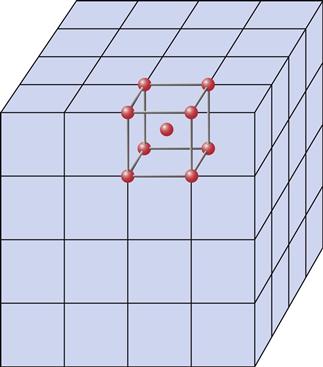
Every lattice has a unit cell (shown in bold) that extends (repeats) in three dimensions for large distances. Electrons are only relatively loosely bound to atomic nuclei and core electrons. The nuclei occupy specific sites (shown as dots in the unit cell) in the lattice, whereas the electrons are relatively free to move about the lattice. In reality, the metal atoms are large enough to touch each other.
The relationships between the atomic centers in a metallic crystalline array are not always uniform in all directions. The distances in the x, y (horizontal), and z (vertical) axes may be the same or different, and the angles between these axes may or may not be 90 degrees. In all, six crystal systems occur (Figure 7-3), and they can be further divided into 14 crystalline arrays. Metallic nuclei may occur at the center of faces or vertices of the crystal. Within each array, the smallest repeating unit that captures all the relationships among atomic centers is called a unit cell (see Figure 7-2). The unit cells for the most common arrays in dental metals are shown in Figure 7-4. In the body-centered cubic (BCC) array, all angles are 90 degrees and all atoms are equidistant from one another in the horizontal and vertical directions. Metallic atoms are located at the corners of the unit cell, and one atom is at the center of the unit cell (hence the name body-centered cubic). This is the crystal structure of iron and is common for many iron alloys. The face-centered cubic (FCC) array has 90-degree angles and atomic centers that are equidistant horizontally and vertically (as does the BCC), but atoms are located in the centers of the faces with no atom in the center of the unit cell (hence the name face-centered cubic). Most pure metals and alloys of gold, palladium, cobalt, and nickel exhibit the FCC array. Titanium exhibits the more complex hexagonal close-pack array. In this array, the atoms are equidistant from each other in the horizontal plane, but not in the vertical direction.
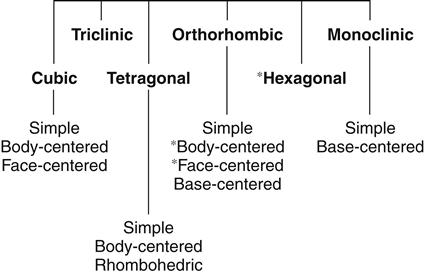
All metals occur in one of the lattice structures shown. There are six families of lattices, four of which can be subdivided. Each family is defined by the distances between vertices and the angles at the vertices. The body-centered cubic, face-centered cubic, and hexagonal lattices (asterisks) are the most common in dental alloys and pure metals.
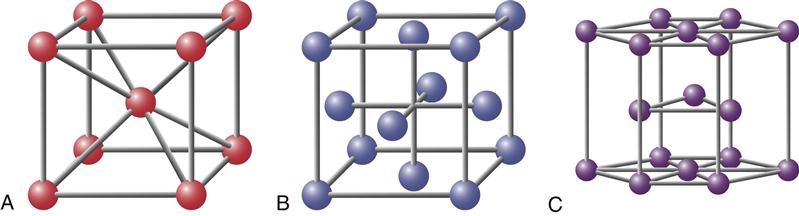
A, Body-centered cubic cell; B, face-centered cubic cell; and C, hexagonal close-packed cell. The atoms (circles) in all three cases would be larger and touching each other. They were drawn smaller to make the structures easier to visualize.
In a metallic crystal, the atomic centers are positively charged because the free valence electrons float in the crystal. Although we might expect the atomic centers to repel each other, the freely floating electrons bind the centers together and create a strong force between the atomic centers. This is known as the metallic bond and is a fundamentally important type of primary bond. The metallic bond is fundamentally different from other primary bonds, such as covalent bonds that occur in organic compounds, and ionic bonds that occur in ceramics.
Physical Properties of Metals
All properties of metals result from the metallic crystal structure and metallic bonds. In general, metals have high densities that result from the efficient packing of atomic centers in the crystal lattice. Metals are electrically and thermally conductive because of the mobility of the valence electrons in the crystal lattice. The opacity and reflective nature of metals result from the ability of the valence electrons to absorb and emit light. Melting occurs as the metallic bond energy is overcome by the applied heat. Interestingly, the number of valence electrons per atomic center influences the melting point somewhat. As the number of valence electrons increases, the metallic bond develops some covalent character that contributes to higher melting points. This phenomenon occurs for iron (Fe3+) and nickel (Ni2+).
The corrosion properties of metals depend on the ability of atomic centers and electrons to be released in exchange for energy. The amount of energy required depends on the strength of the metallic force, which is related to the freedom of the valence electron, and the energy that the released ion can gain by solvating in solution. For metals such as sodium and potassium, the metallic bond is weaker because the valence electrons are loosely held, and the energy of solvation is high. Thus these metals corrode into water with explosive energy release. For metals such as gold and platinum, the metallic bond is stronger; valence electrons are more tightly held, and solvation energies are relatively low. Thus gold and platinum are far less likely to corrode. The corrosion of metals always involves oxidation and reduction. The released ion is oxidized because the electrons are given up, and the electrons (that cannot exist alone) are gained by some molecules in the solution (that are therefore reduced).
Because the distances between metal atoms in a crystal lattice may be different in the horizontal and vertical directions (see Figure 7-4), properties such as conductivity of electricity and heat, magnetism, and strength may also vary by direction if a single crystal is observed. These directional properties of metals and metalloids have been exploited in the semiconductor industry to fabricate microchips for computers. However, in dentistry, a single crystal is rarely observed. Rather, a collection of randomly oriented crystals, each called a grain, generally make up a dental alloy. In this case, the directional properties are averaged across the material. In general, a fine-grained structure is desirable to encourage alloys with uniform properties in any direction. Nonuniformity of directional properties is termed anisotropy.
Like the physical properties, the mechanical properties of metals are also a result of the metallic crystal structure and metallic bonds. Metals generally have good ductility (ability to be drawn into a wire) and malleability (ability to be hammered into a thin sheet) relative to polymers and ceramics. To a large extent, these properties result from the ability of the atomic centers to slide against each other into new positions within the same crystal lattice. Because the metallic bonds are essentially nondirectional, such sliding is possible.
If the metallic crystals were perfect, calculations have shown that the force required to slide the atoms in the lattice would be hundreds of times greater than experiments indicate. Less force is necessary because the crystals are not perfect; they have flaws called dislocations. Dislocations allow the atomic centers to slide past each other one plane at a time (Figure 7-5). Because movement can occur one plane at a time, the force required is much less than if the forces of all the planes have to be overcome simultaneously. An analogy is moving a large heavy rug by forming a small fold or kink in the rug and pushing the fold from one end of the rug to the other. Dislocations are of several types, but all serve to allow the relatively easy deformation of metals. All methods for increasing the strength of metals act by impeding the movement of dislocations.
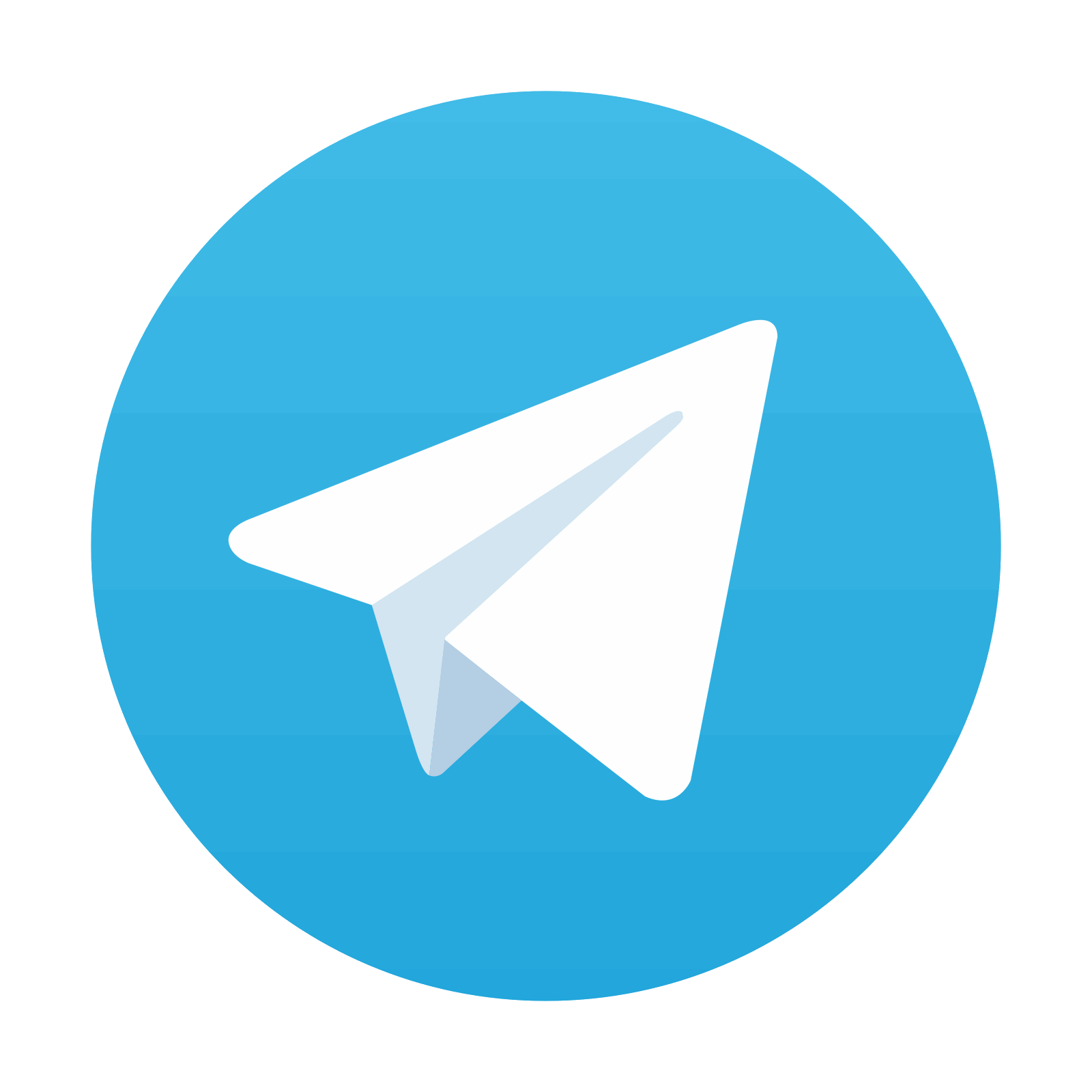
Stay updated, free dental videos. Join our Telegram channel

VIDEdental - Online dental courses
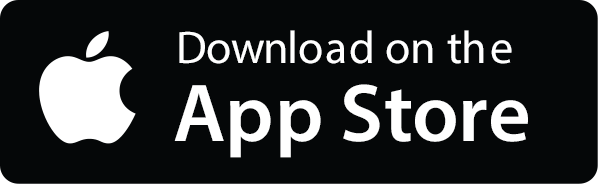
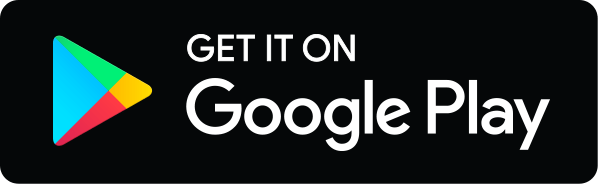