Cells are the central role players in the study of biology. Everything on our earth, which is organic, has come from cells. This is true for the fossil fuels, oil, coal, and natural gas to the fabrics, wood, leather, feathers, cotton, silk, and wool to bone and the two components of teeth: enamel and dentin. The ability of cells to produce such a wide array of products is a measure of just how specialized they can become, often in the same organism. It is also a measure of the level of ordered and coordinated interaction between cells, which allows complex structures to be made. A chick embryo develops a beating heart only 48 hours after a single cell is fertilized. This chapter will be about how cells in the same body become specialized or differentiated, and then how they interact to form complex structures like teeth. There are many similarities in cell interactions, between embryology and wound healing, so this chapter will also describe the replay of embryology that we find during repair of tissues.
5.1 Cell Interactions in Differentiation
5.1.1 Cell Differentiation
We can recognize four basic types of tissue in mammals. They are epithelium, muscle, nerve, and connective tissue. Each tissue group has a wide diversity of cell types, for example, among the epithelial types of cells are many subtypes such as skin, salivary gland secretory cells, and ameloblasts, the cells which form tooth enamel. So, there are many more than just four different types of cell; in fact, there are over 250 cell types in humans. The information that determines a cell’s type is contained in a manual of genetic instructions, the genome, kept in chromosomes inside the nucleus. Every cell of a single species has the same genome; cell types vary due to the expression of different genes within the genome. This expression is regulated by many moderating factors collectively called epigenetic moderators. Many of these moderators are themselves the products of gene expression from within the cell which enhance or inhibit the expression of other genes. These moderators sometimes act alone but more often operate as a network of influences between different moderators. Other moderators reach the cell from promoters in the surrounding matrix or as messengers from other cells. Some of these cell messengers (cytokines) are commonly found during development and in the adult, such as nerve growth factor (NGF) and bone morphogenic protein (BMP). Other cytokines, such as sonic hedgehog (SHH) may be more specifically involved in the differentiation of tooth-forming cells and in determining the morphology of the different tooth types (incisor, canine, and molar).
Cells become differentiated when they express specific genes which will serve a particular cell function. Some of these functions may be quite specialized. For example, when the preodontoblast differentiates to form a functioning odontoblast, it begins to lay down collagen fibers in a specific orientation within an extracellular matrix and then it mineralizes the matrix. Cell differentiation occurs frequently during embryology and healing.
5.1.2 Cell Differentiation during Embryology
The first events during embryology are the division of primitive stem cells, firstly, to form the cells of the endoderm, ectoderm, and mesoderm and then at many subsequent stages of further specialization, as cells follow different pathways to maturity. The 250 cell types can be represented as a branching tree (▶ Fig. 5.1).
Fig. 5.1 A diagrammatic representation of the pathways of differentiation of some cell lines associated with tooth formation. Each tooth is formed from cells which have taken a different developmental route. The odontoblast has its origins from the neural crest, whereas the ameloblast is derived from the oral epithelium.
The differentiation of cells which will form the pulp−dentin, alveolar bone, cementum, and periodontal ligament all have their origins in the neural crest. During the early development of the embryo, the neural plate folds up and closes to form the neural tube which becomes the brain and spinal cord. Cells from the crest of the neural folds detach from the epithelium and enter the mesoderm layer. They are known from this point on as cells of the ectomesenchyme. They migrate far and wide. The migration includes two types of cells. Some of them are committed to their future identities before their migration starts. Others, who travel with them, remain multipotential. The departure and migration of neural crest cells take place during just a few days. On reaching their destination, they differentiate into a variety of cell types, but primarily, the cells of the peripheral nervous system. These include cells of the sensory, sympathetic and parasympathetic ganglia, and the adrenal medulla. They also become pigment cells of the skin and the cells of the branchial arches (▶ Fig. 5.1). The neural crest cells which migrate to colonize the branchial arches have the capacity to begin tooth germ formation. They are collectively known as dental mesenchymal cells.
On their own, these cells are not capable of forming a tooth. This inability to independently progress toward a fully differentiated cell has been studied in cell cultures. If a clump of identical stem cells is artificially grown in isolation, they do not differentiate, but if they are cultured along with a different cell type, they may progress to a further stage of differentiation. This is the case with the dental mesenchymal cells of the developing tooth germ. They require the proximity of cells from the dental lamina, a downgrowth of the oral epithelium to begin to differentiate into tooth-forming cells.
A similar dynamic applies to the cells of the oral epithelium, which will differentiate into ameloblast. In the case of the ameloblast, these moderators come from cells forming dentin, the odontoblasts. Only when the first dentin has been formed, the cytokines will be released which trigger the ameloblast to differentiate into a cell which is able to form enamel. The process of differentiation of one cell by the cytokines produced by a cell of another type has been called induction. It is a process repeated many times during the various stages of differentiation of the cells of the dental lamina and of the dental mesenchyme as they progress to becoming ameloblasts and odontoblasts. Cytokines are just one of the many epigenetic factors which control the expression of genes (see Appendix E.1 Epigenetic Modulation).
5.1.3 Cell and Matrix Interactions in Tooth Development
We have seen the role of gene regulation in bringing forth a new cell line at the right time, but there is less understanding of the mechanism which control tissue shape. What directs a mass of growing cells into the shape of an eye, or tooth, or limb? This process is called morphogenesis, literally the genesis of shape and structure. In order to fully understand the organization and behavior of cell masses, it is worthwhile being familiar with the types of interactions which occur between a cell, its neighboring cells, and the extracellular matrix (see Appendix E.2 Morphogenesis).
The development of the teeth begins with a condensation of epithelial cells of the oral ectoderm, the dental lamina (▶ Fig. 5.2). This forms by a thickening of the epithelium cells along the ridge of both branchial arches which will form the maxilla and mandible. In response, the dental mesenchyme becomes condensed in an area, where the future tooth will develop into a clump of cells which will eventually form the dentin−pulp complex. The initiative for tooth formation then passes to this condensation of cells, and they induce the epithelium to grow downward as a bud, becoming a cap, and eventually a bell-shaped mass of cells. The cells on the inner lining epithelium of the bell are called the inner enamel epithelium (IEE), and they will become the future ameloblasts. The induction continues to pass from one formative tissue to the other in a series of exchanges almost like a verbal dialogue. The preameloblasts do not mature fully and begin to lay down enamel until the odontoblast has formed dentin. Both ameloblasts and odontoblasts retreat from each other as the hard tissues are formed; the ameloblast migrates toward the surface of the future crown and the odontoblast toward the center of the tooth.
Fig. 5.2 A diagrammatic representation of the early stages in the development of a tooth which illustrate induction at a number of occasions. (a) The oral epithelium induces the mesenchyme to condense in areas of a future tooth. (b) The mesenchyme induces a downgrowth of oral epithelium. (c) The “cap” of epithelium induces differentiation of the nearest mesenchymal cells into preodontoblasts, cells which will later form dentin. (d) Further interactions occur between the preodontoblasts and the inner enamel epithelium, which become preameloblasts.
The cells of the IEE and the cells lining the outer surface of the bell, the outer enamel epithelium fuse together and form a sheath of cells which define the shape of the tooth root. This sheath of cells carries the name of its discoverer, Hertwig. Not only does Hertwig’s sheath define the shape of the root dentin, but it may induce the differentiation of the surrounding mesenchymal cells to form cementoblasts, which lay down cementum against the newly formed root (see Chapter 3.6.2 Origins of Cementum). For a complete description of the embryological development of teeth, a text in dental histology should be consulted.
Embryonic cells have the potential to become specialized so as to form any cell from the entire spectrum of adult tissues. The distinctive cell type is determined by the expression of specific genes. Differentiation is the process whereby a particular set of genes is expressed. It is determined by a network of interacting moderating factors, operating both inside and outside the cell.
5.2 Tooth Morphogenesis
5.2.1 Generic Tooth Forms
During the evolution of mammals, the teeth became more specialized than those of their reptile ancestors. Reptiles’ teeth were, and still are today, rather similar cone-shaped pegs. In contrast, mammals have developed a recognizable group of generic shapes to the teeth. There are some notable variations in the details, but all mammals from the elephant to the armadillo conform to the blueprint for four basic generic tooth shapes. In both these two examples, the elephant and the armadillo, some or all of the tooth types are missing.
Firstly, there are a group of single-rooted teeth in front of both jaws which are usually flat and spade shaped. These are the incisors and they work against the teeth of the opposing jaw like flat pincers. The exceptions are the ruminants whose mandibular incisors work against the tongue; the maxillary incisors are absent. At the corner of the arch of teeth, just below the nostril is the second type of tooth which is spear shaped and often the longest tooth. This is the canine. Then there is often a space in the dentition. This is an important option as in some mammals it separates the process of grazing, browsing, or nibbling, with the process of shredding and grinding. The teeth toward the back of the jaw are larger and have a more complex crown and root system. They are subdivided into premolars of intermediate size and molars which are larger.
It is convenient to describe mammalian dentition using a formula which lists the combination of generic types on one side of the jaw. The convention of the dental formula is to list the number of each tooth type in the maxilla over the number in the mandible (▶ Fig. 5.3). There is a wide range of dentitions, all suited to the dietary and feeding habits of each animal, but they all are constructed using the same theme of four basic tooth shapes. Note that cows, sheep, and antelope do not have any maxillary incisors. They graze by using their tongue to rasp of grass against the lower teeth.
During tooth development four generic shapes of tooth are formed. These four shapes are characterized by increasing complexity of the crown and root form. Incisors have one sharp edge (or cusp) and a tiny second one, the cingulum, on the lingual side. Canines have a pointed sharp edge and a bit more of a second cusp, which makes the tooth rounder. Premolars have a well-defined second cusp and may have two or three roots. Molars have more cusps and many roots. Some generic shapes have been adapted. For example, the elephant tusk is a very large lateral incisor.
The mammalian teeth, in comparison to reptilian teeth, play a primary role in its survival. There can be few other anatomical structures which so clearly define the life span of an animal as the teeth of a mammal. When the teeth are worn out, it dies of starvation. It is not a process which is usually witnessed, as wild animals go away somewhere private to die, but domestic animals reveal the true crisis of being toothless. The mandibular incisors of sheep are crucial to their ability to graze, but it is not uncommon, in some countries, for them to suffer from a type of periodontal disease called broken mouth. The incisor teeth become so mobile that they cease to function and the animal’s life is at an end.
5.2.2 Cusp Morphology
It has been noted in Chapter 1 The Origins of Teeth that for the mammalian tooth to perform its vital function, the cusps must wear away to provide a composite tooth surface in order to become an effective working tool. It is also clear from the brief review above that the structural design of the dentition is specific and crucial to the survival of every mammalian type. It can be therefore be inferred that one of the defining characteristics of this structural design is the conformation of the unworn tooth cusps.
In comparison with the dentition of modern humans, whose tooth cusps have not been revealed by reliable evidence to serve a significant function, the cusp design of quadrupedal mammals is of significant importance. It is therefore not surprising to discover that during mammalian tooth development, it is the cusp formation which defines the structure and function of the dentition.
The cusps and roots of teeth are formed during development by folds in the inner enamel epithelium, the layer of cells which will lay down enamel (▶ Fig. A.2). The shape of the inner enamel epithelium and the alignment of the ameloblasts will determine the future shape of the crown (▶ Fig. 5.4). In a review of this process by Simmer and coworkers, it is suggested that the shape of this layer of ameloblasts is determined by several influences, the most significant of which is the gradient of the rate of division of preameloblasts.1 The rate of division is expressed as the mitotic index, which is the ratio of dividing cells to those cells not dividing. The mitotic index of the inner enamel epithelium (preameloblasts) is more rapid in some areas than others. There is thus a gradient between the fastest and slowest dividing cells. Where the mitotic index is highest, the sheet of cells expands rapidly, and a bulge (cusp slope) appears. Where it is slower, the curve of the cell sheet does not expand as rapidly and remains more tightly curved leading to a relatively pointed cusp tip. The cusp tips are formed at points, where the cell division is slowest. When cell division stops, the ameloblasts start to form enamel and that part of the sheet of ameloblasts becomes rigid.
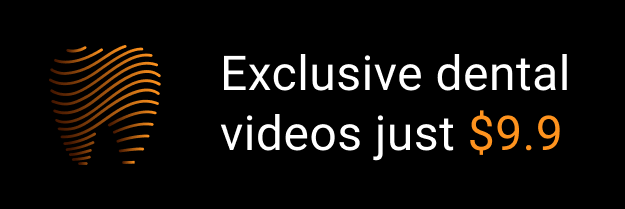