Initial Assessment and Intensive Care of the Trauma Patient
Patient Transport
History of Medical Transportation
Prior to the 1600s, several methods of moving the debilitated were in use around the world. In what would become the United States, Native Americans used the travois (Fig. 4-1), which was essentially a stretcher affixed at one end to a horse or large dog to pull as a conveyance for material or disabled people. In Egypt, camel stretchers called panniers, which were used in many regions around the world with other beasts of burden such as mules, were the method of choice, even through the Napoleonic period, to move the nonambulatory.
The next significant development of U.S. emergency medical services (EMS) and medical transport took place during the Civil War (1861-1865). The Union Medical Department implemented the use of committed, customized horse-drawn wagons as ambulances (Fig. 4-2A) as well as stretcher litters and pack animal cacolets (see Fig. 4-2B). In addition, a dedicated group of stretcher bearers and ambulance wagon attendants and drivers was formed. They received specialized training by the medical department and a tiered transport system was developed. On March 11, 1864, President Lincoln signed a law that was passed by Congress, “An Act to Establish a Uniform System of Ambulances in the Armies of the United States.” This legislation established a standardized system of ambulance service throughout the military. The law also mandated the use of special uniforms for the ambulance corps and special signs for the ambulances. Regulations issued during the war by both sides, and incorporated into this law, also conventionalized specific insignia and signage for the recognition of ambulances and hospitals.
Types of Transport
Helicopter
Helicopters offer several advantages over other transport vehicles (Fig. 4-3). They can travel at speeds of 120 to 180 mph, allowing for transport times to be up to 75% shorter when compared with ground transport. They can avoid traffic delays and ground obstacles and fly into locations inaccessible by other modes of patient transport. Helicopters have landing requirements, which is a disadvantage when compared with ground transport, but they can access more regions than fixed-wing transport. Helicopter cabins are not pressurized and, as a result, patients being transported are at some risk for barotrauma. Another disadvantage is that in most transport programs, helicopters are only permitted to fly under visual flight rules. Hence, weather conditions can limit this operation. However, some programs are now implementing instrument flight rules, which give greater flexibility to flying in less than ideal weather.5
The widespread use of helicopters for patient transport has led to increased accident rates (three times higher) when compared with general helicopter aviation.6 Another study has demonstrated a lower accident rate among busier flight programs and programs that implement instrument flight rules.7 Helicopter ambulances are best used when hospital ground transport time is expected to be longer than 35 minutes or when ground transport is not a viable option. Helicopter transport of critically injured patients from remote areas may be lifesaving; however, there is a large potential for its misuse. The annual cost of helicopter transport service can be at least $1 million for an institution. For effective use of this transport modality, it is generally recommended that helicopter transport should be integrated into the regional EMS system, staffed to provide advanced life support, and used based on medical need.
Initial Assessment
The accurate and systematic assessment of injury is essential to establish the extent of injury to vital structures. This forms the basis of Advanced Trauma Life Support (ATLS) protocols. It is estimated that approximately 25% to 30% of deaths caused by trauma can be prevented when a systematic and organized approach is used.8 Trauma patients should undergo an initial assessment and treatment that is prioritized and corresponds to their injuries and stability of their vital signs. When critical injuries are present, lifesaving measures necessitate that a logical and sequential treatment priority be established, based on the overall assessment of the patient.
Trauma injuries can generally be divided into three categories—severe, urgent, and nonurgent.
• Severe injuries are those that are an immediate threat to life because they interfere with vital physiologic functions. These severe injuries make up approximately 5% of all patient injuries, but represent more than 50% of all trauma deaths.
• Urgent injuries constitute 10% to 15% of all injuries and are not an immediate threat to life. These patients will usually require surgical intervention, but they have stable vital signs.
• Nonurgent injuries account for the remaining 80% of all trauma cases. These do not constitute an immediate threat to life. These patients will generally require medical or surgical intervention after significant evaluation and/or observation.
Assessment Principles
These principles are involved in the initial assessment of a patient with major trauma and have been outlined by the American College of Surgeons (ACS) in their guidelines regarding ATLS protocols.43 These principles are as follows:
2. Primary survey and resuscitation, including monitoring and radiography
3. Secondary survey, including special investigations, such as CT scanning or angiography
Preparation and Communication
A variety of EMS systems exist based on the level of need of a community or jurisdiction. Prehospital medicine exists in two levels of care, basic life support and advanced life support services. Services are provided by emergency medical technicians (EMTs) who are trained at these two different levels of care.9
Trauma Scoring
Glasgow Coma Scale.
This scale is commonly used and was developed by Teasdale and Jennet. The Glasgow Coma Scale (GCS) was the first system to attempt to quantify the severity of a head injury.10 There are three variables used with the scale—best motor response, best verbal response, and eye opening. The key to calculating the GCS is to determine the best response in each category. The motor response is used to assess the level of central nervous system (CNS) function, whereas a verbal response shows the ability of the CNS to integrate information. Eye opening will demonstrate brainstem activity. Each category is given a score, as outlined in Table 4-1, with total scores ranging from 3 to 15. Higher scores represent increased levels of consciousness. The letter T is used to designate that the patient was intubated at the time of examination. A GCS of 8 or less is generally accepted as a definition of coma. At this level, there will often be a monitor placed to measure intracranial pressure (ICP) because it is difficult to monitor these patients for neurologic deterioration as compared with a patient with a higher GCS. Of patients with a moderate head injury (GCS, 9 to 13), approximately 10% to 20% will deteriorate and lapse into a coma. This can influence the timing of the management of maxillofacial injuries. Unless the maxillofacial injures compromise the airway, these patients are not routinely intubated as long as the airway can be protected. For patients with a severe head injury (GCS, 3 to 8), urgent management is critical. These patients are at the greatest risk for mortality and morbidity. They will generally have an endotracheal tube placed to protect their airway. Airway protection can be complicated in the head-injured patient because there are often concomitant maxillofacial injuries.
Trauma Score and Revised Trauma Score.
The Trauma Score was initially developed in 1981 by Champion et al. The purpose of the score was to assess the extent of injury to vital systems and the severity of injury quickly to aid with triage and the treatment of the trauma patient.11 In 1989, Champion et al revised this scoring system, which became the Revised Trauma Score.12 The Trauma Score and Revised Trauma Score quantify the physiologic status of the patient’s respiratory, cardiovascular, and neurologic systems. The revised trauma score has a coded value for three variables—respiratory rate, systolic blood pressure, and GCS. Each variable is coded from 0 to 4, with a total score ranging from 0 to 12. Lower scores represent an increased severity of injury.
Injury Severity Score.
The Injury Severity Score (ISS) was developed to deal with multiple traumatic injuries to multiple organ systems. Scoring is based on the severity of injury to the three most injured organ systems, including respiratory cardiovascular systems, CNS, abdomen, extremities, and skin. Each of the three most injured organ systems is graded from 1 (minor) to 6 (fatal). The grades are then squared and added to come up with a total score from 3 (12 + 12 + 12) to 108 (62 + 62 + 62). The ISS has a practical range from 1 to 75; the risk of death increases with a higher score. ISS rates have been used to predict mortality rates by comparing ISS with mortality by age group. The National Trauma Data Bank (NTDB) uses this scoring system and categorizes an ISS from 1 to 9 as minor, 10 to 15 as moderate, 16 to 24 as severe, and more than 24 as very severe. Figures 4-4 and 4-5 show NTDB data as they relate to the ISS. The average length of stay increases approximately 3 days for each level of severity, as outlined in Figure 4-6.
Mechanism of Injury
The mechanism of injury can provide insight into other possible injuries that have not yet resulted in significant changes in vital signs or physiologic function. The mechanism of injury is usually one of the first issues communicated by EMS to the trauma team as a patient enters the trauma bay. Factors with a high correlation with life-threatening injuries are outlined in Box 4-1.
Other Factors
Various anatomic factors that correlate with high mortality include those listed in Box 4-2. Other factors that have been shown to worsen prognosis of a trauma patient with only a moderately severe injury include concurrent disease, age younger than 5 years or older than 55 years, and cardiac or respiratory disease.
Primary Survey
Airway maintenance with cervical spine protection
Circulation with hemorrhage control
Exposure, environmental control: Undressing the patient but preventing hypothermia
Airway Maintenance with Cervical Spine Control
The airway is especially critical in the head and/or maxillofacial trauma patient. The causes of upper airway compromise in the trauma patient may be tongue position, aspiration of foreign bodies, regurgitation of stomach contents, or facial, mandibular, tracheal and/or laryngeal fractures, bleeding, a retropharyngeal hematoma resulting from cervical spine fractures. or traumatic brain injury.13 Any laceration to the neck is classified according to the anatomic level and aggressively explored. Placement of a surgical airway may be necessary if endotracheal intubation is not possible.
Endotracheal Intubation
The mnemonic LEMON (Table 4-2) is useful when assessing the difficulty for intubation:
Airway Evaluation
1. Observation. Agitation, labored breathing, using accessory muscles indicates hypoxia; obtundation indicates accumulation of carbon dioxide or hypercarbia. Cyanosis, a late sign, will indicate inadequate oxygenation. The use of pulse oxymetry is an adjunct for blood oxygen saturation.
2. Listen for abnormal sounds. Stridor can be associated with partial obstruction of the airway. Hoarseness implies functional laryngeal obstruction.
3. Palpate the trachea and determine whether it is in the midline
Breathing
A patient’s respiratory status should be monitored constantly. Signs of ventilator deterioration warrant placement of a secured airway via an endotracheal tube or the initiation of assisted ventilation. At this point, a patient can be artificially ventilated by a bag-valve mask or a bag attached to an endotracheal tube. Patients who require assisted positive-pressure ventilation with an Ambu-Bag or mechanical ventilator should be closely monitored if their chest status has not been completely evaluated. A simple pneumothorax can be converted into a tension pneumothorax when the intrathoracic pressure increases (Fig. 4-7). In the presence of a pneumothorax, especially a tension pneumothorax, immediate treatment with a regular chest tube or needle insertion is necessary.
Circulation Management
In the primary survey, circulation becomes the priority after airway and breathing have been definitively managed. Delivery of oxygen to the tissues is dependent on adequate circulation. The main cause of deaths that can be prevented is caused by hemorrhage. It is estimated that hemorrhage accounts for 30% to 40% of trauma mortality, with 35% to 65% of deaths occurring in the prehospital period; 50% of deaths secondary to hemorrhage occur within the first 24 hours after the initial trauma.14 In general, blood volume is 7% of body weight; in children, it is considered to be 8% to 9%. Shock in a trauma patient is primarily hypovolemic secondary to trauma, although the patient may present with cardiogenic, neurogenic, or even septic shock. There are conditions that will contribute to a shocklike tension pneumothorax by reducing venous return. Extensive damage to the CNS or spinal cord may result in a neurogenic shock. In very unusual situations, septic shock may be present if the treatment of the patient was initiated several hours after the initial trauma.
Response to Shock
Poor perfusion will affect aerobic metabolism, with nutrients and oxygen being deprived, and consequently result in anaerobic metabolism, with the formation of lactic acid. This will ultimately lead to metabolic acidosis. If this situation continues, the cell membrane will lose its integrity and there will be progressive cellular damage. Continuous hypoxia will result in cellular death. Research has shown that the lethal triad of acidosis, hypothermia, and coagulopathy initially cause the first problems in the polytrauma patient, associated with high mortality.15
The initial damage to soft tissues and organs, and fractures in the trauma patient, represents a major challenge. The local tissue damage resulting from contusions or lacerations, hypoxia, and hypotension result in further damage from local and systemic responses. These processes are activated to preserve immune system integrity and stimulate reparative mechanisms. This systemic inflammation is known as SIRS (systemic inflammatory response syndrome). In addition, the initial inflammatory response is augmented by a second hit, such as ischemia and reperfusion injuries and surgical interventions or infections (two-hit theory).16
Effects of circulatory compromise include the following:
• Decreased or complete loss of consciousness
• Respiratory distress, failure
• Hypovolemia—reduced cardiac output leads to inadequate blood flow to all body organs (hypovolemic shock)
• Tissue hypoxia, metabolic acidosis, increased respiratory rate
• Ischemic injury to the brain, heart, kidneys, liver, and bowel, with cell death and inadequate function of these organs
Assessment
The surgeon should be aware of the simultaneous compensatory mechanisms in a trauma patient and also the individual situation. In the older patient, for example, aging will affect general and specific organ systems that are predictive of failing health. Medically compromising conditions and the use of different medications are frequently present in this group and can contribute to high morbidity and mortality rates with relative minor injuries.17 According to the ABCs of trauma, once the airway is patent and ventilation is reestablished, the hemodynamic status (blood volume and cardiac output) should be assessed, as follows
• Level of consciousness: Cerebral perfusion indicates an adequate circulating volume of blood, although a conscious patient may have a significant amount of blood loss.
• Pulse: The pulse should be checked in central arteries such as the femoral and carotid arteries.
• Respiratory rate: According to the degree of hemorrhage present, patients may become tachypneic as a physiologic response to the need for more oxygen to be delivered to the tissues.
• Blood pressure: If the blood loss is significant (>30% of blood volume), there will be changes in blood pressure.
• Skin color: A gray, pale ashen tone may indicate hypovolemia; pink skin is an indication of good perfusion.
• Urinary output: Urinary output is considered to be in normal limits with approximately 0.5 mL/kg/hr for the adult and 1 mL/kg/hr for children. A decrease of urinary output to less than 30 mL/hr in an adult may indicate hypovolemia in the absence of other medical conditions (e.g., renal damage).
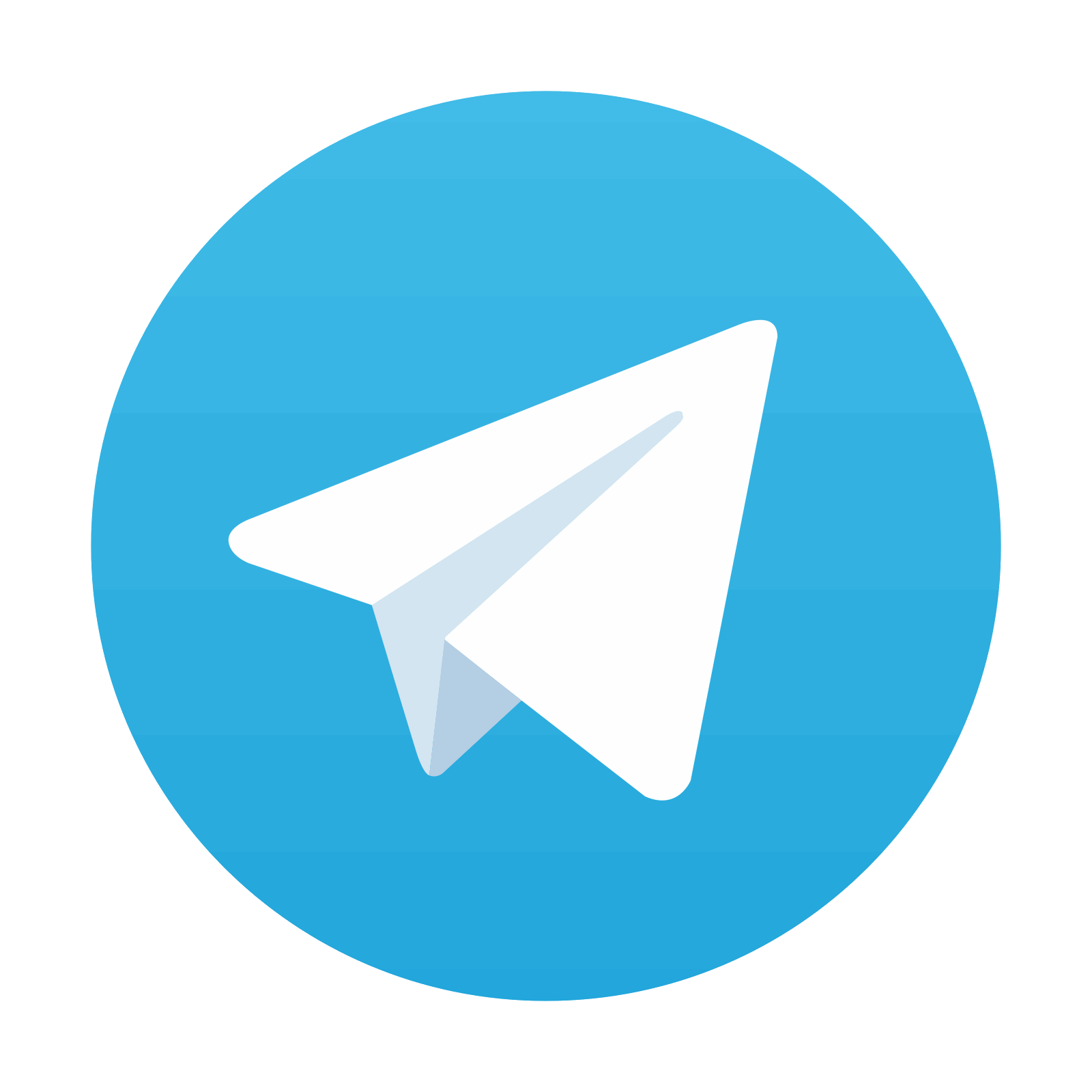
Stay updated, free dental videos. Join our Telegram channel

VIDEdental - Online dental courses
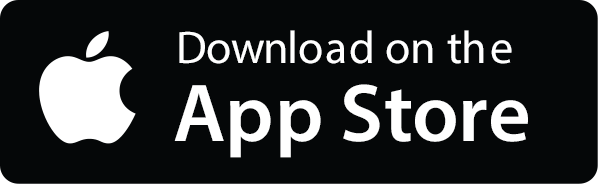
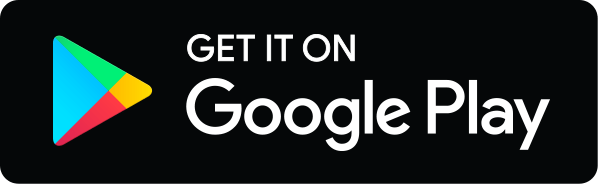