Principles of Fixation for Maxillofacial Trauma
History of Fracture Treatment and Development of Modern Osteosynthesis
Bone fractures have been treated with various conservative techniques for centuries and it was not until the eighteenth century that internal fixation was first documented. Icart, a French surgeon in Castres, performed ligature fixation with brass wire on a young man with a humeral fracture. Since then, many surgeons developed several methods for internal fixation, but one of the greatest leaps in bone trauma surgery was in 1886, when Hansmann of Hamburg published a technique using retrievable metal bone plates with transcutaneous screws. Soon after, a Belgian surgeon, Albin Lambotte, improved these techniques and coined the term internal fixation. Lambotte developed and manufactured a variety of bone plates and screws and much of his armamentarium remained in use until the 1950s.1
Biology of Bone and Bone Healing
Bone’s organized structure is illustrated in cross section revealing the haversian system, or osteon. Each osteon contains concentric layers of compact bone surrounding a central haversian canal, which harbors the neurovascular bundle supplying the unit. Cells suspended in this highly calcified, highly vascular structure are perfused via small capillary-containing cylindrical cavities called canaliculi.2
Bone healing can be broadly categorized in two ways, primary and secondary. Primary, or direct bone healing, requires rigid fixation and immobility of fracture segments with a minimal gap between them (less than 100 µm). Osteoclasts migrate to the fracture site and widen adjacent haversian systems, allowing osteoblasts to deposit bone matrix, or osteoid, eventually to calcify into organized mature lamellar bone.3
Secondary, or indirect bone healing, is more complex and occurs when a significant gap or interfragmentary motion is present. Secondary bone healing involves the formation of a fibrocartilaginous intermediary bone callous (Fig. 33-1) There are four distinct stages of indirect bone healing but the end product is the same as mature bone formed in primary healing. The initial insult propagates the inflammatory stage. A hematoma between and around the fracture develops and stabilizes, drawing inflammatory cells to the site. Necrotic and nonviable bone near the fracture is cellularly débrided and repair is initiated by angiogenesis and the activation of osteoprogenitor cells and fibroblasts. The second, or soft callus, stage is characterized by conversion of the hematoma to a fibrocartilaginous mass to bridge the fracture. Fibroblasts and mesenchymal elements are highly active in laying down new collagen to create the substrate into which the third phase, or hard callus stage, develops. During this period, osteoid is calcified and periosteal and endosteal bone ingrowth starts to replace the soft callus as a result of endochondral bone formation. Finally, in the remodeling stage, the woven bone of the hard callus matures and organizes to a trabecular structure to re-create the native preinjury structure.4
Although distinct, both types of bone healing may occur simultaneously in the same fracture. As three-dimensional structures, bones may have varying levels of contact and fracture reduction in the same general site, resulting in endochondral and lamellar elements in different areas at the same point in time (Fig. 33-2).
Biophysics of the Facial Skeleton
It is imperative to understand the physics and forces applied to facial bones to fixate fractures properly and use biomechanics to our advantage. Due to its dynamic nature, the mandible bears most of the forces applied by facial musculature to the skeleton. Beam mechanics dictates that the mandible is a class III lever, with the condyle being the fulcrum, the muscles of mastication acting as the applied force, and bite load acting as the resistance (Fig. 33-3). This rationale applies to one side of the mandible at a time, but as a horseshoe-shaped bone, the mandible is more than a simple class III lever. Vectors from bilateral muscle contracture on a unilateral fracture produce rotation and torques that cannot be easily described by simple beam mechanics.5,6 Varying bone thickness, occlusal arrangements, and multiple vectors of muscle contraction also cannot be accounted for in this model. For the purposes of understanding rigid fixation across fractures, beam mechanics applies.
FIGURE 33-3 The mandible as a class III lever.
When loaded, the mandible exhibits maximum tension at the superior border and maximum compression at the inferior border (Fig. 33-4). This is a gradient and, between the zones of tension and compression, lies a neutral zone in which opposite forces total zero. In this model, it would be mechanically advantageous to apply rigid fixation hardware along the zone of tension, or superior border.7 Biologically, this is complicated by the presence of teeth, thin cortical bone, and thin overlying soft tissue. The neutral zone is dynamic and depends on the moment of the bite force applied, but generally correlates to the plane in which the inferior alveolar neurovasculature lies, and therefore not acceptable to place hardware. The inferior mandibular border is a biologically sound location for hardware placement. Bicortical screw fixation here is extremely stable, there is adequate vascular soft tissue to prevent dehiscence or hardware palpability, and dental structures usually do not extend to this area. To compensate for lack of stability at the zone of tension, a tension band can be applied. This could be a monocortical low profile miniplate, lag screw, or arch bar, all of which resist tensile forces without violating biologic principles of surgery.
In vivo, the mandible is more complicated than the model just described. Because of the three- dimensional nature of the mandible, multiple muscular insertion sites, and bilateral nature of the muscles, zones of compression and tension are not always static around a fracture. For example, in the mandibular corpus or parasymphysis, forces may be inverted if bite force is applied posterior to the fracture line secondary to forces applied from the contralateral musculature.8 This creates compression at the alveolus and tension at the inferior border. Torsional forces also act on segments due to the bilateral nature of the forces applied. This is easily demonstrated by rotation of segments around the neutral zone when only one point of fixation is implemented or widening of the mandible at the condyles due to alveolar process torquing.9
Methods of Fixation
Traumatic injuries of the facial skeleton can be properly managed and treated in numerous ways. AO-ASIF guidelines of rigid fixation follow four basic principles to ensure adequate treatment of fractures: bony segment reduction, stable fixation and immobilization of fragments, maintaining blood supply, and early function.10 As noted, primitive methods of internal fixation developed from the 1800s. Advancements in techniques and biomaterials and an understanding of biophysics have greatly changed how we currently apply fixation to facial fractures. Internal fixation with titanium hardware is still the most commonly used method of treating facial skeletal injuries and a plethora of systems are available to carry this out. Various sizes and shapes of plates and screws exist to meet the needs of the surgeon and to tailor treatment to each individual patient and fracture type.11
Cases in which surgical exposure of fracture sites may interrupt blood supply, such as severely comminuted fractures or contaminated wounds, pose a risk for hardware infection and may be an indication for skeletal pin external fixation.12 This technique works especially well in comminuted and contaminated mandibular fractures requiring control of non–tooth-bearing proximal segments. Transcutaneous access to the mandible with stab incisions and blunt dissection is achieved. The mandible is drilled using a drill guide to protect the soft tissue and skeletal pins are inserted into the fractured segments. Ideally, two pins with a large distance between them should be placed into each large segment to prevent rotation. To ensure optimum biomechanics, each pin should be as large as possible and placed close to fracture lines (but not closer than 1 cm to prevent the risk of segment shearing). The external components of the skeletal pins are stabilized by being fastened to a rigid external bow or linking segments with the use of other, smaller subunit bars. Smaller shards of comminuted bone generally do not require fixation because they can be immobilized as they are sandwiched between larger externally fixated segments. Care is taken not to devitalize these small bone segments by avoiding aggressive débridement. Maintaining blood supply is essential to the healing of these fractures. In cases in which internal fixation cannot be applied without devitalizing bone segments, such as a grossly contaminated open mandibular fracture, external fixation may serve as a temporary measure to immobilize and stabilize the segments while the wound is washed out and sterilized over a period of days to weeks. This can be ultimately converted to internal plate fixation to avoid prolonged skeletal pin hardware application.13
Maxillomandibular fixation (MMF) or interdental fixation is widely used in the management of almost all injuries affecting the jaws. As described, this is an excellent method for achieving fracture reduction. Prior to the development of modern internal fixation, MMF was the mainstay of facial fracture treatment. By stabilizing the dentition in its known pretraumatic occlusion, bone segments will assume an anatomically acceptable configuration. Because MMF compresses fractures at the alveolus, the inferior border of the mandible may still demonstrate a gap. By combining this method with compression of the inferior border with bone reduction forceps and application of internal fixation methods, an ideal reduction can be achieved. Several hardware designs and techniques are available for MMF including arch bars, Ivy loops, Stout interdental wires, and MMF screws. The advantage of MMF with the application of arch bars is that they provide a tension band at the alveolar component of maxillomandibular fractures. This aids in resisting tensile forces of the fracture near the teeth. Also, arch bars have numerous fixation points (lugs), allowing more precise control of fracture segments and application of force vectors when placing the patient into MMF. In fractures not involving dentate portions of bone—that is,. mandibular angle, ramus, or condyle fractures—arch bar application may not be necessary to obtain MMF. If a stable and reproducible occlusion can be obtained, the use of MMF screws or Ivy loops can provide reduction and stabilization of the fracture in a much shorter, less technique-sensitive manner.14 Hand articulation of the jaws has also been described and deemed successful by some authors. These methods are also more hygienic than the use of standard arch bars, prevent excessive biofilm accumulation, are less traumatic to the periodontium, and can be removed relatively quickly.15 Although not as rigid and mechanically stable as arch bar MMF, with appropriate patient selection, screw and/or interdental wire MMF can provide excellent results for the nonoperative management of mandibular fractures.16 A retrospective cohort of 75 patients with 83 mandibular angle fractures treated by Bell and Wilson17 has described three different methods of intraoperative intermaxillary fixation prior to the application of a single, 2.0- mm miniplate via the Champy technique. There were no significant differences in outcomes, reoperation rates, and complications among the Erich arch bar, Stout interdental wire, and manual reduction groups.
MMF is still used as a primary modality of fracture treatment in patients for whom internal fixation may not be indicated. Minimally or nondisplaced biomechanically favorable fractures in patients with a sufficient complement of teeth to provide a stable premorbid occlusion, severely comminuted fractures, or intracapsular condylar fractures in which occlusion can be reestablished are some common scenarios for which 2 to 8 weeks of MMF without surgery may be indicated. MMF is considerably less invasive and more cost-effective and reduces complications associated with open surgery; however, it poses its own unique set of risks and complications. MMF invokes an aspiration hazard in patients such as those with severe gastroesophageal reflux, nausea, seizure disorder, alcoholism and dysphagia. Patient compliance is essential to prevent loosening or cutting of wires and mobilizing the forming callus. There is a great deal of evidence from orthopedic literature demonstrating ill effects on the skeletal system from prolonged immobilization and disuse of long bones. These effects are demonstrable not only on the immobilized bone itself, but on the associated muscles, vasculature, and joints. Bone immobilization results in hypomineralized osteoporotic cortices and trabeculae and an overall decreased oxygen tension and pH of the nutrient vessels. Muscles inserting on this bone also atrophy with disuse and usually require extensive physical therapy for the patient to return to full strength and mobility.18,19 Decreased muscle demand inevitably results in hypovascularity and protein loss over time. Surrounding immobilized joints demonstrate pericapsular contractures and synovial hyperplasia, which decrease range of motion. Over time, the formation of intracapsular adhesions can result in pain, inflammation, and derangements in joint function. The dreaded complication of TMJ ankylosis may follow prolonged immobilization of displaced or comminuted condylar fractures.20,21
Condylar fractures are surrounded by controversy in respect to closed versus open treatment. Internal fixation tends to produce superior results in regards to anatomic reduction, but studies have shown variability in postoperative mandibular function, symmetry, and joint pain. Variability in fracture patterns of the condyle, concomitant mandibular fractures, occlusal stability, patient compliance, postoperative physiotherapy, and operative technique have significant effects on study outcomes of closed and open fracture treatment and have led to opposing viewpoints. A meta-analysis of several studies has failed to show any overwhelming overall benefit of closed versus open treatment due to lack of consistency in reporting the variables described.22,23
Rigid Versus Functionally Stable Fixation
Internal fixation can be subclassified in several ways, but perhaps the most appropriate would be delineating between rigid and nonrigid. Rigid fixation can be defined as any type of directly applied bone fixation that prevents interfragmentary movement between fracture segments when that bone is under active load.24 Examples of rigid fixation of a fracture include application of a reconstruction plate, two bone plates, two lag screws, or a compression plate and arch bar across a fracture. With the exception of the use of a load-bearing reconstruction plate, rigid fixation techniques rely on two point fixation—a stabilizing unit, such as a bone plate at the inferior border, and a tension band, such as a miniplate or arch bar superior to that.25,26 On a histologic level, the benefit of rigid internal fixation with minimal gap between the bone segments allows for primary bone healing via haversian remodeling. The fracture gap is traversed by osteoclasts and then undergoes angiogenesis, followed by deposition of osteoid by adjacent osteoblasts. The bone remodels over time to create mature haversian bone. This is in contrast to fractures with a significant gap or interfragmentary motion, which heal by secondary intention and formation of an intermediary hematoma and bone callus.
Nonrigid fixation is just that—fixation that allows for movement between the bone fragments across a fracture line. Many older techniques, such as interosseous wiring or interdental bridal wiring, stabilize fractures to approximate segments but do not prevent interfragmentary movement. Depending on the magnitude of movement across the fracture, nonrigid fixation may result in nonunion or malunion. With the methods and materials available today for maxillofacial trauma surgery, many types of nonrigid fixation are no longer in use. Perhaps the most important nonrigid technique in mandibular trauma is the Champy method for the fixation of angle fractures. In 1978, Champy described the use of a single miniplate adapted to the superior border of mandibular angle fractures, with excellent results. This technique has been termed functionally stable because it allows for activation of the mandible during healing, even with interfragmentary motion.27 Due to the rostral force vectors of the pterygomasseteric sling and caudal pull of the suprahyoid muscles, the superior border of the mandibular angle is under tension and the inferior border is under compression. Because the monocortical miniplate is applied to the superior border, the mechanical advantage favors stabilization of the fracture under active forces. Although functionally stable fixation of the mandibular angle reduces operative time, risk of dental injury, and cost, it is not ideal in all situations. Concomitant fractures of the mandible must be treated rigidly to prevent motion at multiple sites. The Champy method relies on the contralateral condyle being seated correctly in the glenoid fossa, without disruption of the temporomandibular relationship. If a contralateral fracture is present and not treated rigidly, bite forces across the angle can transmit to the distal segment, causing rotation around the opposite fracture line. This may result in widening of the mandible and subsequent malocclusion and facial width alteration. By treating the other fracture site rigidly, the angle can essentially be treated as an isolated injury.
Compression Plate Osteosynthesis
The goal of compression osteosynthesis, as described by AO, is establishing absolute stability across a fracture. This is defined as zero movement occurring between bones across the fracture, as well as complete immobility of the hardware against the bone. This creates an ideal environment for primary bone healing by generating friction between the bone segments in compression and minimizing the gap between them.28 Linear compression between the segments counteracts torsional forces produced by the masticatory apparatus during function and prevents interfragmentary motion. Traction perpendicular to the fracture line is maintained by the plate itself, which shares the load under function and maintains compression of the segments.29
Today, most mandibular plating modules include dynamic compression plates for surgeons who wish to use compression osteosynthesis (Fig. 33-5). Although efficient in creating absolute stability in mandibular fractures, compressive plating techniques, even with the advent of the dynamic compression plate, are extremely technique-sensitive and prone to operator error. If the holes are not drilled in the proper location and screws are not placed in the correct sequence, the hardware can distract the fracture, creating gaps in bone, malocclusion, and excessive torsional forces across the plate.30
FIGURE 33-5 Dynamic compression plate.
Dynamic compression plates are designed with eccentric holes with inclined planes. On either side of the midline of the plate, the plate holes are elongated, with the lateral side having the highest portion of the inclined plane and the medial with the lowest portion, or closest to the bone, of the inclined plane (Fig. 33-6). The plate should be adapted so that one eccentric hole is on each side of the fracture, closest to the fracture line. The outer planes of each hole are the active, or compression, sites. As screws are drilled and fastened into this high point of the inclined plane, they follow the plane down toward the bone as friction is created between the screw head and plane surface. When completely tightened, they lie on the innermost portion of the hole closest to the bone. Because this is completed on either side of the fracture, the bone segments are compressed toward each other while the plate remains static, minimizing the bone gap and achieving compression. The remainder of the holes distal to the fracture line are then drilled and secured with bone screws in a passive position so as to not compress or distract the bones and hardware further/>
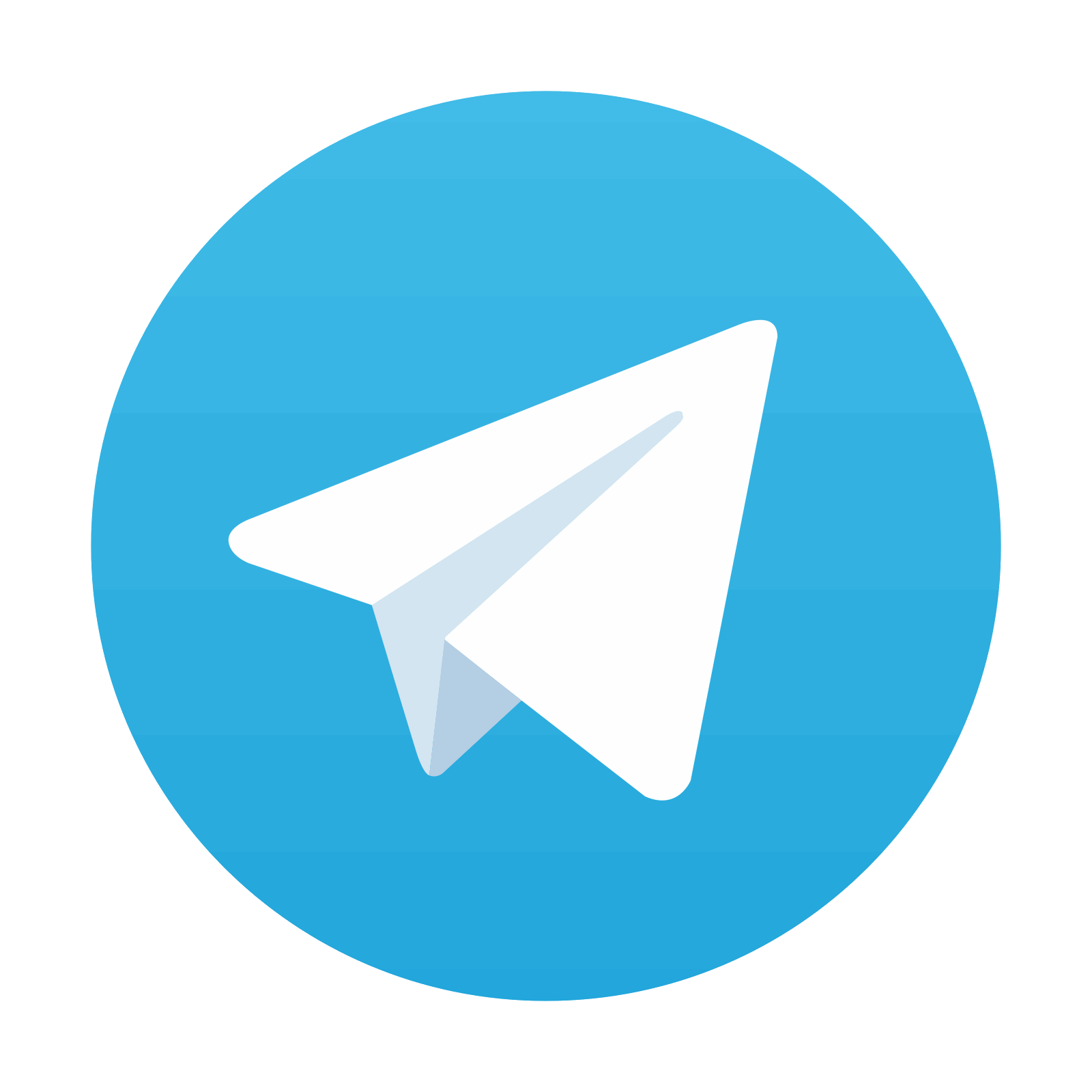
Stay updated, free dental videos. Join our Telegram channel

VIDEdental - Online dental courses
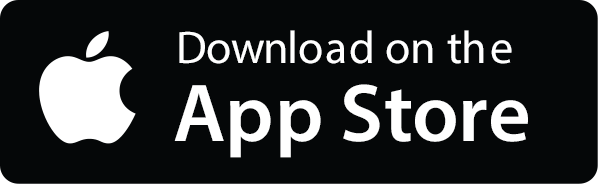
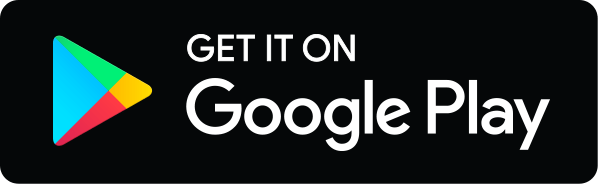