CHAPTER 26 A NEW CONCEPT OF TAPERED DENTAL IMPLANTS
PHYSIOLOGY, ENGINEERING, AND DESIGN
Biological Background
The NobelActive implant design is based on the physiology of bone surrounding natural teeth and implants. A clinically successful dental implant must create and maintain integration with hard and soft tissue.1 However, some authors have alluded to the presence of remodeling after implant placement, which manifests in diminished bone dimensions, vertically and horizontally.2 Implant placement also has been unable to preserve the hard tissue dimension after tooth extraction, and the buccal and lingual walls are often resorbed.3,4 The theories surrounding critical bone volume (CBV) and well-known factors about bone physiology are the main concepts that aid in understanding the process involved in maintaining bone dimension.
Critical Bone Volume Theory
The CBV theory supports the idea that bone tissue can survive and maintain its original dimensions, providing sufficient volume and/or blood supply to maintain the surrounding bone. Data support the concept of a positive relationship between the densities of blood vessels and the formation of bone.5,6 Consequently, even if the blood supply has been altered in the supporting structures of the natural teeth or dental implants the bone can survive when minimal volume remains.
Bone Physiology
Bone is a living, growing tissue. It is a porous, mineralized structure made up of cells, vessels, calcium salts, and crystals of calcium compounds (hydroxyapatite), the proportion of which varies according to bone types and regions. The human skeleton is made up of two types of bone: cortical bone and trabecular bone.7
Cortical bone, also called compact bone, represents nearly 80% of the skeletal mass. It is called compact bone because it forms a protective outer shell around every bone in the body. Cortical bone has a high resistance to bending and torsion and it provides strength where bending would be undesirable. It has a specialized vascular system, called the Haversian system, with an inside net of vertical and horizontal canals and blood vessels to overcome limited diffusion capability. Cortical bone has a slow turnover rate, progressing approximately 0.6 micron a day. It can be estimated that an injury to the cortical bone can take up to 5 months to repair.7
Trabecular bone comprises only 20% of the skeletal mass but 80% of the bone surface. Trabecular bone is less dense and more elastic than cortical bone, and forms the interior scaffolding that helps the bone to maintain its shape despite compressive forces. Trabecular bone is rigid but appears spongy. It is composed of bundles of short, parallel strands of bone fused together. The center of the bone contains red and yellow marrow bone cells, and other tissues. Trabecular bone has a higher turnover rate than cortical bone, and as a result has a quicker healing capacity, progressing about 20-50 microns a day.6
The outer surfaces of cortical bone are covered with the periosteum membrane, which is composed of an irregular type of dense connective tissue. Periosteum is divided into an outer fibrous layer and an inner cambium layer. The fibrous layer contains fibroblasts; the cambium layer contains progenitor cells that develop into osteoblasts. These osteoblasts are responsible for increasing the width of long bones and the overall size of the other bone types. Unlike osseous tissue, periosteum has nociceptor nerve endings that make it very sensitive to manipulation. It also provides nourishment by providing the bone’s blood supply.8 Trauma to the periosteum and cortical bone must be minimized because the most superficial bone layer gets all of its blood supply from the periosteal tissue and 30% of the blood supply to the bone comes from and returns to the periosteum.
Periosteum is attached to bone by strong collagenous fibers called Sharpey’s fibers, which are the ends of principal fibers that insert into the cementum. Sharpey’s fibers intensify the continuity between the periodontal ligament fiber and the alveolar bone (tooth socket), and act as a buffer medium against stress. Sharpey’s fibers in the primary acellular cementum are mineralized fully; those in cellular cementum and bone are mineralized only partially at their periphery,9 which extends to the outer circumferential and interstitial lamellae. They also provide attachments for muscles and tendons.8
It is important to understand the vascular supply to the soft and hard tissue of the oral cavity. The blood supply to the periodontium has three main sources: the blood vessels of the alveolar bone, the blood vessels of the periodontal ligament, and the supraperiosteal blood vessels.10 The teeth are surrounded by the periodontal ligament, gingival tissue (circumferential fibers), and a lamina dura. The periodontal ligament comprises a group of specialized connective tissue fibers that essentially attach each tooth to the alveolar bone within which it sits. These fibers help the tooth withstand the naturally substantial compressive forces that occur during chewing and remain embedded in the bone11 (Figure 26-1).
The buccal bone adjacent to natural teeth is usually only 0.5-1 mm thick. This bone gets its blood supply from the periosteal layer and the periodontal ligament. The gingival side of the outer periosteum of bone anastomoses with the periodontal ligament intra-alveolar blood vessels.12 Why is the buccal wall adjacent to natural teeth so thin? It has been observed that buccal bone remains thin even after orthodontic movement, and that it follows the movement of the teeth.4 One evolutionary explanation for buccal bone thinness might be protection against dental infections. An infection usually leads to buccal bone perforation with secretion throughout the buccal side (as opposed to the lingual side), which prevents potentially life-threatening infection of other vital structures such as the floor of the mouth or the palate.
Flap elevation can lead to increased bone loss.13 In particular, trauma from drilling procedures affects the vascularity of the bone, which, in turn, leads to resorption.14 The cortical bone is especially vulnerable to resorption because the blood supply comes from the periosteal tissue and the Haversian system, which are damaged during the surgical procedure. Even more drill-related trauma may occur as the drill becomes wider and the flap becomes larger and is exposed for a longer period of time.
Transmission of Forces to Natural Teeth and Bone
According to Wolff’s law, bone in a healthy person adapts to the load placed on it. If loading on a particular bone increases, the bone remodels itself over time to become strong enough to resist that sort of loading. As a result, the external cortical portion of the bone becomes thicker and trabecular bone becomes denser and oriented toward the direction of the forces exerted on it. The converse is also true; if the loading on a bone decreases the bone will become weaker due to turnover. Weaker bone is less metabolically costly to maintain and without increased loading there is no stimulus for continued remodeling required to maintain bone mass.15,16
Occlusal forces are first transmitted to the coronal part of the teeth and then are partially transmitted horizontally to the adjacent teeth through the contact points of the crowns. The rest of the forces are transmitted to the bone through the periodontal ligament. The periodontal ligament structure allows for a special tridimensional architecture of fibers that absorb some of these forces and transmit some to a dense bone layer around the roots (lamina dura), which are connected to the cortical bone. In turn, the lamina dura transmits some forces to the trabecular bone and cortical bone of the jaws. All of these mechanisms of force absorption and transmission also enable orthodontic movements, in which forces reorganize and remodel the periodontal ligament to facilitate tooth movement.17
The internal structure of the surrounding bone changes after implant placement. The bone around the implant is replaced through bone healing and remodeling.18 The dense lamina dura that was present in natural teeth disappears. As a consequence, occlusal forces are transmitted mainly to the cortical bone. This is true for most implants on the market due to their structural design, which lacks features to absorb and transmit occlusal forces and maintain the blood supply from the periodontal ligament. The reduced blood supply and concentration of forces on the crestal cortical bone can lead to resorption of this vulnerable tissue.
Clinical Observations Supporting the CBV Theory
Buccal Wall Resorption After Extraction
Buccal bone is resorbed after tooth extraction. The bone-to-implant contact established during the early phase of socket healing following implant installation is in part lost when the buccal bone wall undergoes continued resorption. After tooth extraction the buccal bone resorbs over several months.1,3,19 It is therefore recommended that the postextraction socket should be filled with a bone augmenting material.20,21,22
The goal of ridge preservation procedures is preservation of the hard and soft tissue volume and architecture and maximization of the supply of osteoprogenitor cells and their ability to invade the area occupied by the osteoconductive scaffold.21 The key factor in ridge preservation is prevention of postextraction bone resorption. There often is extensive resorption of the buccal wall and minimal resorption of the palatal/lingual wall because the palatal wall has a better blood supply from the thick palatal gingiva and presents a volume above the CBV. In most cases, the buccal wall volume decreases to below the CBV. According to the CBV theory, there is a high probability of maintaining bone dimensions without bone augmentation procedures if there is sufficient buccal wall volume of bone and good blood supply.
Interdental Bone and Papilla
Between two adjacent teeth there is a thin layer of bone supporting the interdental papilla. The interdental bone and papilla get most of their blood supply from the periodontal ligament of the adjacent teeth. The papilla is configured in a tridimensional orientation of fibers to maintain its architecture. When teeth are extracted the blood supply to interdental bone and the papilla is altered, resulting in bone resorption and papillary dimension decreases.23,24 If only one of the adjacent teeth is extracted, adequate blood supply will still come from the periodontal ligament of the remaining tooth. This leads to more predictable and aesthetic results in single-tooth implants between two natural teeth. It is more difficult to create a good papilla between two adjacent implants.25
When every tooth is replaced with a standard implant with a conical design and a wide coronal neck, the crestal bone volume between two adjacent implants is very small. This inter-implant crestal bone is usually below the CBV and as a consequence it will resorb. This becomes even more evident as the implants are placed closer to each other. If the distance between two adjacent implants is less than 3 mm,26 which is below the CBV, the resorption of the interdental bone increases.
The use of a pontic has been advocated to overcome the problem of inadequate bone volume between implants.27,28 It is possible to restore a three-unit bridge on two implants using an ovoid pontic. The papilla can be maintained underneath the pontic because the bone has an adequate blood supply provided by the adjacent periodontal ligament and periosteal tissue in three directions: buccal, lingual, and crestal. The use of an ovoid pontic instead of an implant allows more space for preserving the dental architecture and the underlying bone and soft tissues. A pseudo-papilla can be created by modeling the gingival soft tissue.
Bone Walls Around Standard Implants
A certain amount of bone resorption occurs around implants when they are in contact with the oral cavity. The distance between an implant and the adjacent tooth, the distance between two implants, is as important to maintaining bone as the bone volume around the buccal side of the implant head and in the papillary area. This is true especially for a successful long-term result.29 It is a well-known fact that 1.5-2 mm of bone must surround the implant buccally and lingually.29,30
In summary, the bone has more blood supply when a tooth is present or absent than when a tooth has been replaced with a dental implant. As an example, the bone around a wider diameter implant is at higher risk for crestal bone resorption because a greater amount of bone is needed to surround the implant.31 It is recommended that the width of the buccal and lingual bone plates around 5- to 6-mm diameter implants must be at least 3-4 mm to achieve the CBV.
Distraction Osteogenesis
Both bone grafting and distraction osteogenesis are predictable methods for restoring missing tissue. The main cause of bone resorption after distraction osteogenesis is the trauma of laying a flap involving the periosteum, drilling through the bone, and/or the insertion of the fixating screws. When performing a distraction osteogenesis procedure, the bone segments must be wide enough to comply with the CBV theory and maintain bone volume.32,33
Augmentation Prevents Bone Resorption
Augmentation is necessary to prevent resorption. It can occur at or after tooth extraction. There are many techniques for alveolar ridge augmentation, such as guided bone regeneration (GBR); onlay/veneer grafting (OVG); combinations of onlay, veneer, interpositional inlay grafting (COG); distraction osteogenesis (DO); ridge splitting (RS); free and vascularized autografts for discontinuity defects (DD); mandibular interpositional grafting (MI); and socket preservation (SP).34 The main idea behind augmentation is to maintain adequate volume to comply with the CVB theory and avoid bone resorption.
Implant Exposure
Immediately after insertion of an implant in a two-stage procedure, there are no significant changes in the bone structure around the implant or at the crestal level. Healing after the surgical implant placement procedure results in defect fill and clinically healthier situations.35 When the implant is submerged, it is protected from occlusal loads and bacterial infection. Also, it obtains its vascularity from the crestal periosteum in the adjacent bone.
After exposure to the oral environment, marginal bone loss of 1-2 mm occurs during the first year.36 Possible reasons for this bone loss include micromovement, microgaps, and bacteria infiltration at the implant and abutment interface. Another possible explanation is the damage to the thin crestal bone during placement of implants with wide coronal diameters. After 3-6 months the bone heals by resorption and apposition, but it is not functional bone. Changes start to occur once the implant is exposed to the oral cavity, where greater occlusal forces36,37 are introduced to the crestal bone than to the trabecular bone.
Implant Requirements
The understanding of bone behavior around implants has aided the development of an implant that minimizes trauma to the cortical bone and condenses the trabecular bone. The reduction of trauma to the bone will influence the preservation of bone and soft tissue, including the papilla. It is important to consider the support system around an implant. The implant supports the abutment and the abutments support the restoration. However, this whole implant system is held in place by the most important element, which is the bone. The bone is the foundation for and the sustenance for the soft tissue. Box 26-1 lists the requirements for the NobelActive implant.
BOX 26-1 Requirements for a third-generation implant
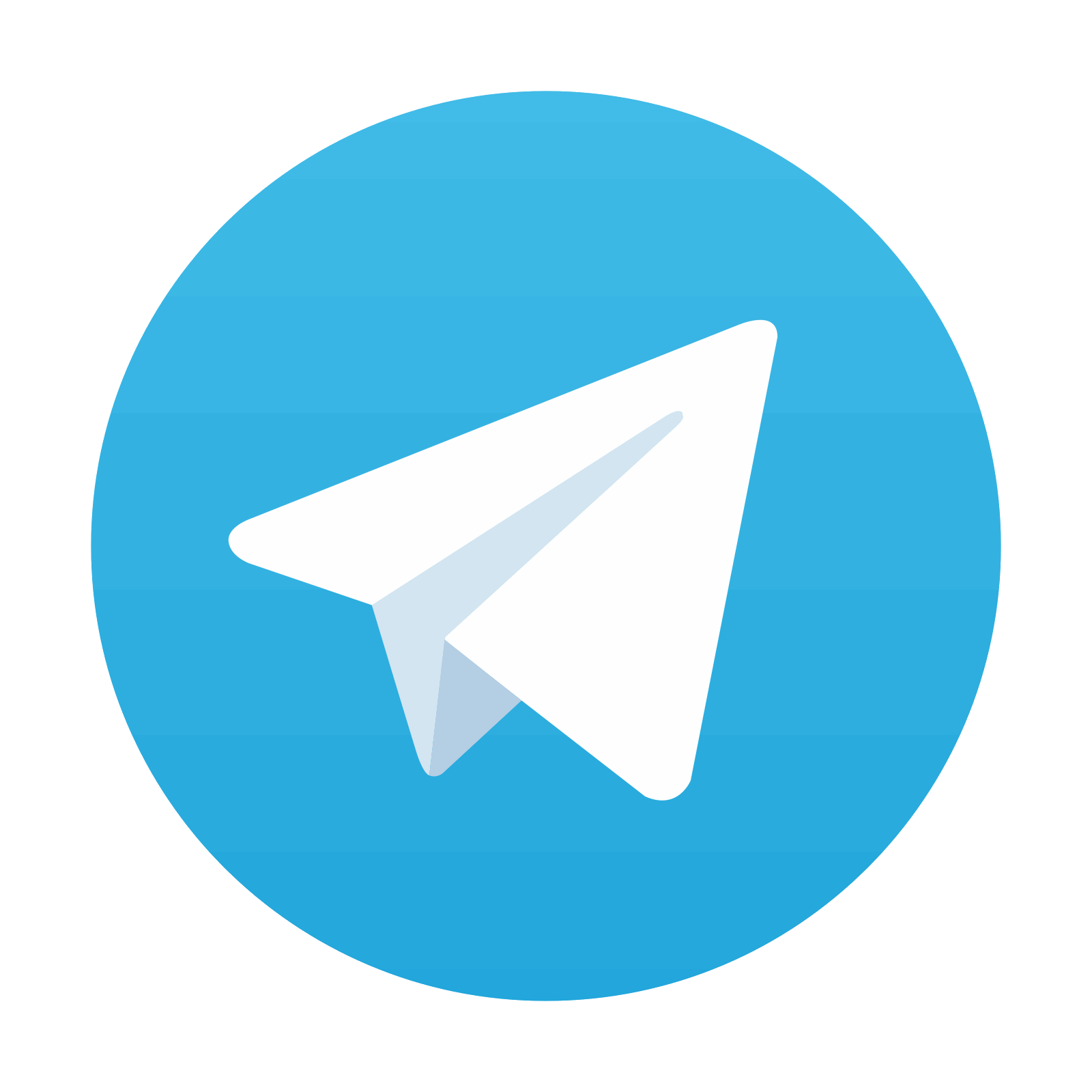
Stay updated, free dental videos. Join our Telegram channel

VIDEdental - Online dental courses
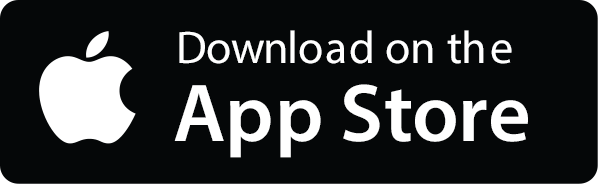
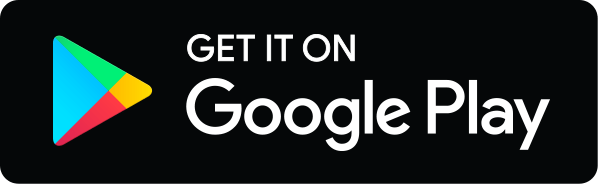