2
Accelerated tooth movement following alveolar corticotomy: Laboratory canine model evidence
Donald J. Ferguson
European University College, Dubai Healthcare City, UAE
Accelerated tooth movement
One of the biological constraints in orthodontics is the amount of time it takes to resolve malocclusion. A reduction in active treatment time would logically mean that teeth would need to move faster. Treatment times for nonextraction and extraction orthodontic treatment strategies respectively average 23.8 years and 28.1 years, according to Buschang et al. (2012), and corticotomies increase the rates of tooth movement and decrease treatment duration. Long et al. (2013) evaluated the effectiveness of five interventions on accelerating orthodontic tooth movement, including low-level laser therapy, corticotomy, electrical current, pulsed electromagnetic fields, and dentoalveolar or periodontal distraction; corticotomy was judged as safe and able to accelerate orthodontic tooth movement.
It is well established that tooth movement rate is, in part, a function of alveolar bone density (Verna et al., 2000), and that tooth movement is accelerated under conditions of low bone density. Bone densities change regularly as bone renews itself. Roberts et al. (2004) contrasted the process of bone renewal for cortical and trabecular bone; cortical bone requires an activation that initiates resorption (cutting cones) followed by formation (filling cones) in a couple sequence; that is, remodeling, otherwise known as secondary osteon formation. Trabecular bone is thin, which sustains a simpler process; that is, modeling, wherein stimulus activation can result in either apposition or resorption. The milieu of orthodontic tooth movement is trabecular bone modeling with the exception of the thin cortical lamina dura surrounding each tooth root.
Orthodontic clinicians understand that tooth movement results from alveolar bone resorption and formation, and that application of a biomechanical force results in a shift in cell population dynamics within the periodontal ligament (PDL). Until sufficient osteoclasts and osteoblasts have accumulated within the PDL, tooth movement after initial force application is limited to the width of the PDL space; this 3–5-week “lag” phase dissipates as PDL cell populations supportive of tooth movement have accumulated and hyalinization has diminished (Von Böhl and Kuijpers-Jagtman, 2009).
Orthodontic researchers understand “bone turnover” as a phrase describing the dynamics of a living osseous tissue that by nature is compensatory and adaptive. The research methods for the study of bone turnover include histomorphometry; that is, quantitative analysis of the physical size and shape (form) of bone. “Bone turnover” is a histomorphometric expression that includes anabolic (apposition) and catabolic (resorption) bone changes that occur and an appraisal of the varying degrees and relative amounts of mineral salts, namely calcium, present or absent.
Alveolar demineralization or decreased alveolar bone density leads to increased tooth movement rate (Verna et al., 2000). Reduction of the availability of calcium metabolite (i.e., calcium manipulation either pharmacologically or through diet), results in greater tooth movement rate and scope (Midgett et al., 1981; Goldie and King, 1984; Engström et al., 1988; Verna and Melsen, 2003).
Corticotomy
Corticotomy is the intentional cutting of only cortical bone leaving intact the medullary vessels and endosteum and was first cited in the professional dental literature in the late 1800s to correct malocclusion. Alveolar decortication has evolved during the past decade, gathering noteworthy attention of orthodontic clinicians and academicians. After lying fallow for the previous half century, interest in the corticotomy concept was rekindled by Köle’s (1959) journal publication describing interproximal corticotomy combined with subapical through-and-through osteotomy in the rapid treatment of an openbite patient. Seminal technique changes since Köle include corticotomy-only (without subapical osteotomy) in the orthodontic treatment of two apertognathia patients (Generson et al., 1978), and blending augmentation bone grafting with corticotomy-only in combination with orthodontics treatment (Wilcko et al., 2001).
For 5 years following the Wilcko et al. (2001) publication, only a few corticotomy articles appeared in the professional literature (Chung, 2001; Chung et al., 2001; Ferguson et al., 2001; Hwang and Lee, 2001; Owen, 2001; Ferguson, 2002; Wilcko et al., 2003; Iseri et al., 2005; Germec et al., 2006; Iino et al., 2006).
Anecdotal accounts of accelerated tooth movement following corticotomy abound in the literature. But it was not until 2007 that evidence based upon controlled experimentation became available describing post-corticotomy rapid tooth movement. Corticotomy experiments in laboratory animals prior to 2007 focused on tissue responses (Düker, 1975), but tooth movement rate or magnitude were not study variables until after 2006.
Since 2007, interest in alveolar decortication as a technique to enhance the rate of tooth movement increased notably (Fischer, 2007; Kanno et al., 2007; Lee JK et al., 2007; Moon et al., 2007; Wilcko MT et al., 2007, 2009; Lee W et al., 2008; Nowzari et al., 2008; Oliveira et al., 2008; Park et al., 2008; Wilcko WM et al., 2008; Akay et al., 2009; Chung et al., 2009; Dibart et al., 2009, 2010; Ferguson, 2009; Kim SH et al., 2009; Kim S-J et al., 2009a,b; Murphy et al., 2009; Roblee et al., 2009; Spena et al., 2007; Wang et al., 2009; Hassan et al., 2010b; Choo et al., 2011; Keser and Dibart, 2011; Kim et al., 2011). Moreover, two alveolar corticotomy literature reviews were published in regional journals (AlGhamdi 2010; Hassan et al., 2010a) and one in an international journal (Long et al., 2013). Ten of the more recent experimental publications have included data on tooth movement as a study variable in human and/or animal subjects with additional information on the biology of alveolar bone change dynamics (Cho et al., 2007; Iino et al., 2007; Kim SH et al., 2009; Mostafa et al., 2009; Cohen et al., 2010; Sanjideh et al., 2010; Aboul-Ela et al., 2011; Baloul et al., 2011; Iglesias-Linares et al., 2011; Safavi et al., 2012).
Accelerated tooth movement in laboratory animals
The validity of bone research in laboratory animals depends upon the choice of the animal model (Buschang et al., 2012). Rogers (1993) points out that there are at least three characteristics of an animal model: (1) convenience, (2) relevance (comparability to the human condition), and (3) appropriateness (a complex of other factors that make a given species the best for studying a particular phenomenon). As long as the limitations of a specific animal are candidly addressed by the investigator, experimental data can lead to a valid understanding of the effects on corticotomy-induced bone loss on the human skeleton.
It is clear that bone composition in some species more closely resembles human bone composition than others (Jee and Yao, 2001). Aerssens et al. (1998) compared cortical bone composition, density, and quality in bone samples derived from seven vertebrates that are commonly used in bone research: human, dog, pig, cow, sheep, chicken, and rat. Large interspecies differences were observed in all analyses of cortical bone; rat bone was most different, whereas canine bone best resembled human bone. Trabecular bone density and mechanical testing analyses also demonstrated large interspecies variations; human samples showed the lowest bone density and fracture stress values, and porcine and canine bone best resembled the human samples. In summary, of all species examined by Aerssens et al., the bone composition of the dog most resembles that of human bone.
Ten refereed journal articles have been published after 2006 with precision measurement of experimental and control tooth movement following alveolar corticotomy from which amount and rate of tooth movement could be ascertained (Table 2.1).
Table 2.1 Tooth movement following alveolar decortication injury is represented by 10 evidence-based, refereed, professional journal articles through to May 2013. All investigations include measurements of accumulated and/or rate of tooth movement and provide experimental and control data typically in a split-mouth design. One study is in humans (Aboul-Ela et al., 2011) and the remainder used either laboratory dogs or rats.
First author | Subjects | Article title | Journal details | Injury type | Tooth movement type | Data type |
Cho | Dog | The effect of cortical activation on orthodontic tooth movement | Oral Dis 13: 314–319, 2007 | Bur buc-ling with flap Split mouth |
Tipping Up3 after ext Up2 Translate Lp3 after ext Lp2 |
Longitudinal |
Iino | Dog | Acceleration of orthodontic tooth movement by alveolar corticotomy in the dog | AJODO 131: 448e1–8, 2007 | Bur buc-ling with flap Split mouth |
Translate Lp3 after ext Lp2 | Cross-sectional |
Mostafa | Dog | Comparison of corticotomy-facilitated vs standard tooth-movement techniques in dogs with miniscrews as anchor units | AJODO 136: 570–577, 2009 | Bur buccal with flap Split mouth |
Tipping Up1 after ext Up2 | Cross-sectional |
Kim | Dog | Effects of low-level laser therapy after corticision on tooth movement and paradental remodeling | Lasers Surg Med 41: 524–533, 2009 | Scalpel buc-ling No flap Split mouth |
Translate Up2 after ext Up1 | Longitudinal |
Sanjideh | Dog | Tooth movements in foxhounds after one or two alveolar corticotomies | EJO 32: 106–113, 2010 | Bur buc-ling with flap Split mouth |
Translate Up3 after ext Up2 Translate Lp2 after ext Lp3 |
Longitudinal |
Cohen | Dog | Effects of increased surgical trauma on rates of tooth movement and apical root resorption in foxhound dogs | Orth Craniofac Res 13: 179–190, 2010 | Bur buccal with flap Remove labial cortex Split mouth |
Distract Up2 after ext Up1 | Longitudinal |
Baloul | Rat | Mechanism of action and morphologic changes in the alveolar bone in response to selective alveolar decortication-facilitated tooth movement | AJODO 139: S83–101, 2011 | Bur buc-ling with flap Split mouth |
Tipping – non-extract | Cross-sectional |
Aboul-Ela | Human | Miniscrew implant-supported maxillary canine retraction with and without corticotomy-facilitated orthodontics | AJODO 139: 252–259, 2011 | Bur buccal with flap Split mouth |
Translate Up2 after ext Up1 | Longitudinal |
Iglesias-Linares | Rat | The use of gene therapy vs. corticotomy surgery in accelerating orthodontic tooth movement | Orth Craniofac Res 14: 138–148, 2011 | Bur buc-ling with flap Split mouth |
Tipping – non-extract | Cross-sectional |
Safavi | Dog | Effects of flapless bur decortications on movement velocity of dogs’ teeth | Dent Res J 9: 783–789, 2012 | Bur buccal no flap Corticotomy monthly split mouth |
Translate Up2 after ext Up1 | Longitudinal |
Seven of the 10 investigations used dog as the laboratory model, two studies used the rat (Baloul et al., 2011; Iglesias-Linares et al., 2011), and there was one human study (Aboul-Ela et al., 2011). The type of alveolar cortical bone injury was by surgical bur in all but one project, which used a scalpel (Kim SH et al., 2009) and which was excluded from further consideration. Translation-type tooth movement following extraction was the prospective design of all studies except three; one was buccal tipping (Iglesias-Linares et al., 2011) in rats, a second was mesial tipping (Baloul et al., 2011) in rats, and a third was dental distraction (Cohen et al., 2010) in dogs. Dental distraction or distraction osteogenesis articles were not considered.
Accelerated tooth movement in the canine laboratory model
Canine study description and design
Given the fact that bone of the canine laboratory animal best resembles the human condition, five dog investigations utilizing bur injury for corticotomy were isolated for further consideration and data pooling (Table 2.2).
Table 2.2 Five investigations of tooth movement following alveolar decortication utilizing the canine laboratory model are summarized by author and year, dog type, sample size and data type, time after extraction and tooth movement (TM) force used, corticotomy surgery details, tooth movement type, anchorage and appliance used, measurement, location and frequency.
First author Year | Type dog (sample = n) Data type | Extraction healing TM force | Corticotomy surgery details | TM type | Anchorage + appl | Measurement Location Frequency |
Cho (2007) |
Beagle (n = 2) Longitudinal |
4 weeks healing Up3 mesial – 150 g Lp3 mesial – 150 g |
Flap + non-perf cortex “dots” 12 holes injury – #2 rd bur Buccal–lingual |
Tipping Up3 after ext Up2 Translate Lp3 after ext Lp2 |
U canine L canine + slot guide |
Digital vernier caliper Gingival margins Weekly for 8 weeks |
Iino (2007) |
Beagle (n = 12) Cross-sectional |
16 weeks healing Lp3 mesial – 51 g |
Flap + vert & horizontal cuts 1 mm wide – #009 fissure bur Buccal–lingual |
Translate Lp3 after ext Lp2 | L canine Bands + fixed tube |
Caliper & radiograph Protocone tip Lp3 Superimposed tracing Weeks 1, 2, 4, 8 |
Mostafa (2009) |
Non-purpose-bred (n = 6) Cross-sectional |
Immediate Up1 distal – 400 g |
Flaps + vert & horizontal cuts and 8–10 holes – #2 rd bur Extract site buccal cortex |
Tipping Lp1 after ext Lp2 | Miniscrew Lp1 cervical lig. tie |
Boley gauge Notched cervical crown Weekly for 5 weeks |
Sanjideh (2010) |
Foxhound (n = 5) Longitudinal |
Immediate Up3 mesial – 200 g Lp2 distal – 200 g |
Flaps + cuts around root #702 tapered fissure bur Buccal to Up3 Buccal–lingual to Mn ext site |
Translate Up3 after ext Up2 Translate Lp2 after ext Lp3 |
Teeth Bonds + fixed tube |
Digital caliper & radiograph Implant bone markers Lp2 mesial tube to Lc tip Up3 mesial tube to Uc tip Days 10, 14, 28, 42, 56 |
Safavi (2012) |
German (n = 5) Longitudinal |
Immediate Up2 mesial – 150 g Lp2 mesial – 150 g |
Flapless + holes thru attached Pointed tungsten carbide bur 25 penetrations 2 mm deep Buccal to p2 and extract site |
Tipping Up2 after ext Up1 Tipping Lp2 after ext Lp1 |
Miniscrew Up2 cervical lig tie Lp2 cervical lig tie |
Digital caliper Marks on p2s & canines Months 1, 2, 3 |
Cho et al. (2007) utilized two beagles in the longitudinal examination of mesial movement of the upper and lower third premolars after extraction of all second premolars followed by 4 weeks of post-extraction healing. Corticotomy technique included upper and lower full-thickness flaps and 12 cortex non-perforating “dots” with a no. 2 round bur into the buccal and lingual cortical plates in the right maxillary and mandibular quadrants in a split-mouth design. The third premolars were protracted into the extraction site with 150 g nickel–titanium closed coil springs using adjacent teeth as anchorage; upper third premolars were tipped and lower third premolars were tipped-translated with a “guide-wire and slot” device (Figure 2.1). Measurements were made weekly with a vernier caliper at the cervical–gingival margins of teeth adjacent to extraction site.
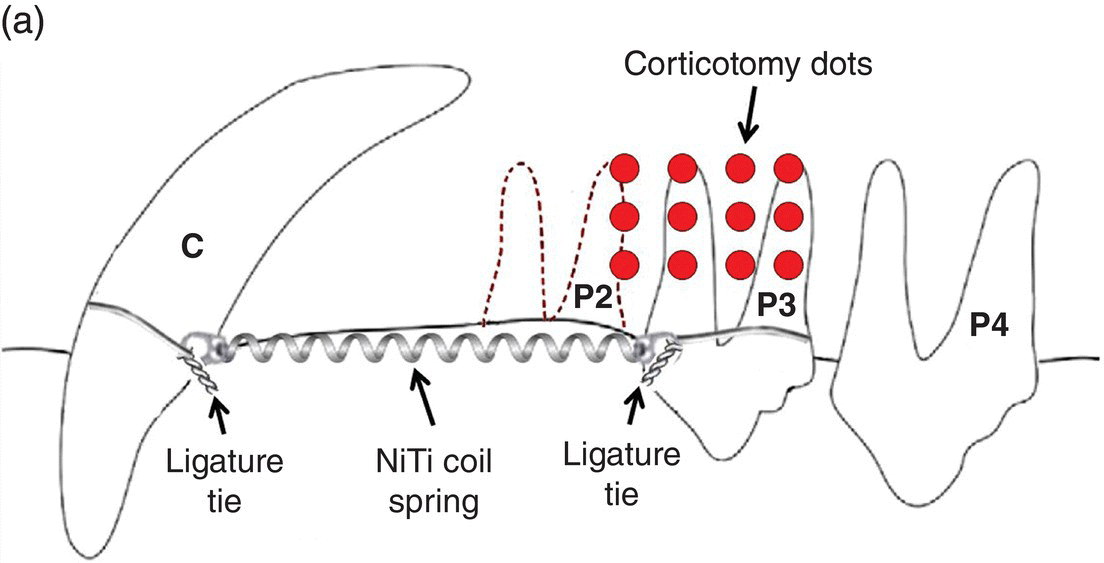
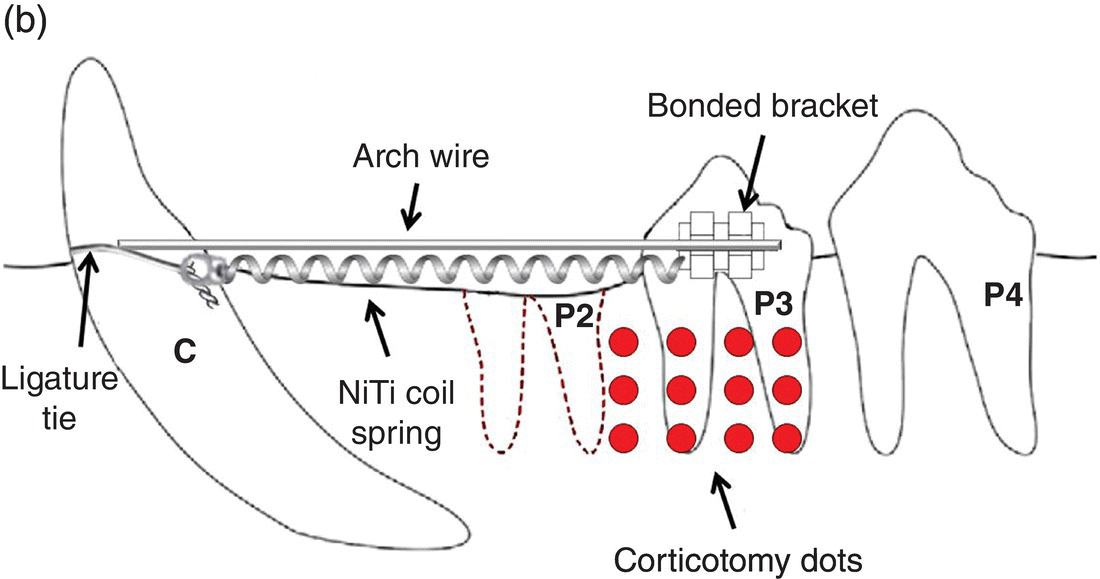
Figure 2.1 Cho et al. (2007) used 150 g nickel–titanium closed coil springs placed 4 weeks after extraction of upper and lower second premolar (P2) and affixed to maxillary third premolars and canines with ligature ties around the cervical crowns. Corticotomy technique consisted of 12 dots buccal and lingual to the third premolars (P3) using a no. 2 round bur. The mandibular orthodontic appliance differed from the upper and was comprised of a 0.017 × 0.025 mm2 stainless steel guide wire from mandibular canine (C) sliding through an orthodontic bracket bonded to the mandibular third premolar (P3).
Iino et al. (2007) used 12 adult beagle dogs in the cross-sectional evaluation of mesial movement of lower third premolars after extraction of the mandibular second premolars and 16 weeks of post-extraction healing. Corticotomy technique included lower full-thickness flaps and no. 009 fissure bur to make horizontal subapical and vertical interproximal cuts perforating the alveolar cortex buccal and lingual to the lower third premolar in a split-mouth design. The third premolars were protracted into the extraction site with 0.5 N (51 g) nickel–titanium closed coil springs using adjacent teeth as anchorage; lower third premolars were translated mesially with a fixed band-and-tube cemented appliance (Figure 2.2). Measurements were made at 1, 2, 4 and 8 weeks from radiographs taken using a standardized format. Radiographs were superimposed on the fourth premolar and measured between the tips of protocone of the third premolar on the tracing; error of method was 0.02 mm.
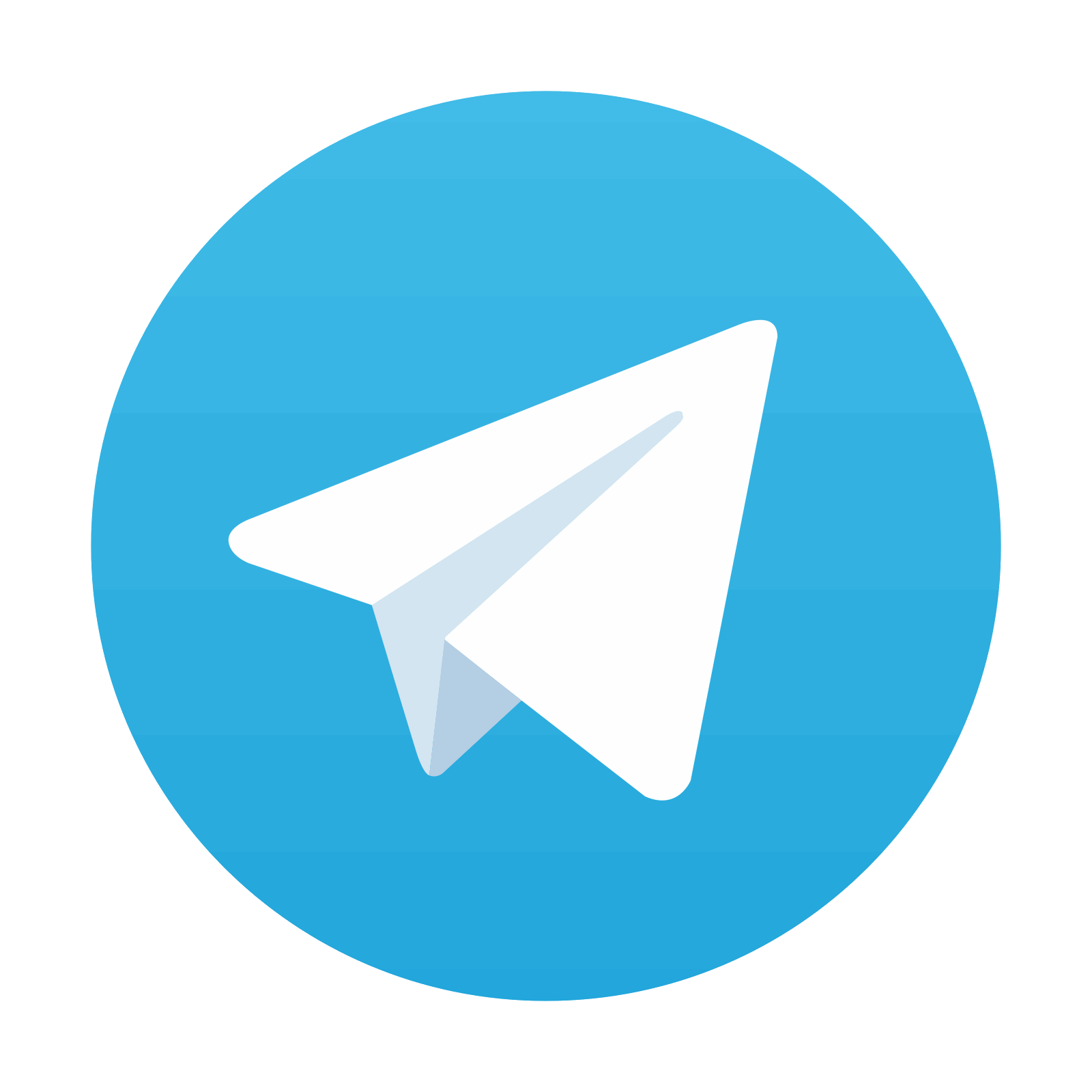
Stay updated, free dental videos. Join our Telegram channel

VIDEdental - Online dental courses
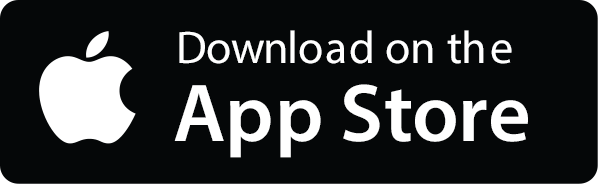
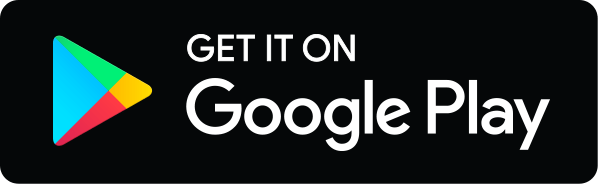