Nanotechnology in dental materials
Introduction
Nanotechnology is concerned with the development and application of matter at the scale of nanometres, or 10−9 m. By convention, a nanomaterial is any material which includes components or features with at least one dimension less than 100 nm. The term is sometimes ‘stretched’ to cover those materials with features of several hundred nanometres, perhaps to cash in on the current vogue for all things ‘nano’! To use features at this scale offers a wide range of advantages and opportunities to the materials engineer, and for this reason nanotechnology continues to have a profound effect on many areas of science, technology, medicine and consumer products.
In this chapter we will discuss the impact of nanotechnology on dental biomaterials. This is a rapidly developing field and what we present here is something of a snapshot of the state of play in late 2012. Doubtless some of the innovations that are in their infancy now will become commonplace in the dental surgery in years to come, while others will prove to be ineffective, or too expensive, or too challenging in scale-up to become widespread.
Nanofilled Dental Composites
Dental composites (Chapter 2.2) are composed predominantly of silica filler particles embedded in a polymer matrix. The size of the filler particles has a profound effect on the properties of the material. To an extent, smaller is better – the composite can be better polished and the translucency and aesthetics controlled if the filler particles are small. The earliest composites, which had large filler particles, suffered from rough and therefore dull, plaque-retentive surfaces. However, reduce the particle size too far and you are likely to run into trouble with wettability. Since specific surface area increases with decreasing particle diameter, there comes a point for a given filler loading where there is insufficient resin to wet the filler particle surfaces and the composite becomes porous, crumbly and dry. This places a limit on the total filler surface area that can be incorporated into a composite, which is a function of both particle size and total loading.
Nevertheless, nanoscale filler particles have found application in dental composites, in combination with larger filler particles. In fact this is nothing new; ‘nanofilled’ composites, composites which contain nanoparticle fillers, were on the market long before the term ‘nanoparticle’ was applied to dental biomaterials! The ‘microfilled’ composites developed in the 1970s contained filler particles as small as 40 nm [1], and modern ‘hybrid’ composites contain filler particles of the size that would be defined as nanoparticles now we have a name to put to them.
So, one could easily be cynical about the array of ‘nanocomposites’ that adorn the catalogues of the dental materials suppliers; dentists have been using products that could be described as exactly that for decades. That would be a hasty judgement, however. Nanocomposite development is certainly a very active area of research and there are some promising early indications. To be a true nanomaterial, a nanocomposite should contain structural features where at least one dimension is less than 100 nm, although the term is sometimes used, both in the scientific literature and in marketing material, to apply to those materials where the features are anything below 1 µm, or those where nanoparticles are arranged in clusters that might themselves form more of a microscale object. It would be beneficial to the dental community if a globally accepted definition of a dental nanocomposite could be developed, and this might quite reasonably insist on a scale of <100 nm. In the absence of such an accepted definition, we will discuss nanocomposites with these and with larger features as well.
It can be difficult to form a thorough and balanced picture of the properties of nanofilled composites since any one study may evaluate only a few materials and the results cannot necessarily be extrapolated to apply in a wider context. In some published studies, the nanofilled composites are compared to non-nanofilled materials that also exhibit other differences in composition or structure, making a genuine evaluation of the effect of the nanofillers impossible. Thus one must interpret research findings with caution and scrutinize studies and claims with a critical eye.
A recent large study compared 72 different commercial brands and models of composite and compomer, of which 7 were classed by the authors as ‘nanohybrid’. It appears that the source of the classification was in the marketing material supplied by the manufacturer rather than a more rigorous criterion such as fraction of filler with diameter below, say, 100 nm, and this is a limitation of the study. It appears from this comparison that the materials classified as nanohybrids exhibited a moderate flexural strength and modulus of elasticity but a high tensile and compressive strength in comparison to other composite classifications [2].
Nanoscale fillers may, of course, be composed of materials other than silica. Nanoparticles of calcium phosphate have been incorporated into experimental composites, and the preliminary indications are that the wear rates of these and conventional composites were comparable while the mechanical properties of the nanocomposites were somewhat superior and a sustained release of calcium and phosphate was observed, which may potentially foster remineralization in the surrounding tissue [3,4]. The calcium and phosphate release was able to elevate the pH in an artificial plaque model, while a conventional composite was not [5]. The same team has investigated calcium fluoride nanofillers which appear to confer fluoride release on the nanocomposite materials and might conceivably offer remineralization benefits [6]. Given that secondary or recurrent caries around the restoration is a common reason for the failure and replacement of composites, this could represent a significant step forward. These are interesting nanocomposites developed by respected researchers and will be watched with anticipation, although they are not yet independently tested or commercially available.
Of course, roughly spherical particles are not the only option for nanoscale fillers. There has been recent interest in developing nanoscale fibres to reinforce dental composites; fibre-reinforced materials are already used extensively and to great effect in civil and aerospace engineering. Composites containing zirconia–silica and zirconia–yttria–silica nanofibres (albeit rather large ones with diameters in the 100–300 nm range) [7] substituted for the regular glass filler had significantly higher flexural strength, flexural modulus and energy at breakage than the same composites with only the silica fillers, and some compositions had increased fracture toughness as well. However, these improvements were at the expense of the degree of conversion of monomer to polymer in the material [8]. Composites containing modest substitutions (5–10%) of hydroxyapatite nanofibres, again with diameters around 100 nm, also exhibited increased flexural strength compared to those containing only silica fillers, but these composites also showed elevated water sorption and solubility [9,10]. The contrast between fibre-like and spherical nanofillers was investigated in a study utilizing smaller hydroxyapatite nanofibres, with diameters of around 50 nm, and comparing these with roughly spherical hydroxyapatite nanoparticles. The nanofibre-reinforced composite was found to have superior flexural strength and fracture toughness when comparing composites with the same mass fraction of substituted spherical nanofillers [11]. This is thought to be because the nanofibres act as crack deflectors and crack bridges as well as being more easily wetted by the resin, and less likely to aggregate, than the spherical nanoparticles. The highly nanofibre-filled composites did, however, display a rather high porosity. Thus there are some interesting developments in this area, but also a number of significant drawbacks which will need to be addressed if these formulations are ever to reach the chairside.
Nanofilled Glass–Ionomer Cements and Related Materials
Nanoscale filler particles have been incorporated into glass–ionomer cements (GICs) and their resin-modified counterparts. For the fundamental chemistry and properties of GICs the reader should consult Chapter 2.3. The reader will recall that one of the significant properties of GICs is that they release fluoride, although there remains some controversy regarding the clinical outcome of this. The primary focus so far on nano-GICs has been the impact of the nanofiller on fluoride release and uptake characteristics. Two independent studies have indicated that a resin-modified GIC containing nanoscale silica fillers as well as conventional (size and composition) fillers exhibited similar fluoride release and recharge behaviour to a regular resin-modified GIC [12,13]. Enhanced fluoride release has been achieved by adding nanoparticulate fillers to resin-modified GICs, not of the conventional silica–alumina–calcium fluoride glass but of fluorapatite or fluorhydroxyapatite, although this was only measured up to 70 days after preparation so it is not clear how long this would be sustained and whether it could be recharged as with conventional fluoride release from GICs [14], or indeed whether it would make any difference to caries in the area surrounding the restoration.
As regards other properties of nanofilled GICs, the data are rather scattered, with only a few properties tested for any one composition, and there is less of a feel for what these fillers might be able to offer this class of material than there is for composites.
Hydroxyapatite and fluorapatite nanofillers have been added to a commercial GIC and have been shown to increase the compressive, tensile and flexural strength [15] and enhance the bond strength to dentine [16]; other physical, chemical or biological properties were not investigated. Titania (titanium dioxide) nanoparticles have been added to GICs with the primary intention of conferring an alternative, or additional, antimicrobial efficacy on the material, since titania exhibits antimicrobial properties when activated by exposure to light of wavelength 380 nm. GICs containing 3% titania nanofillers reduced growth of Streptococcus mutans and also improved some mechanical properties such as fracture toughness and flexural and compressive strength; higher concentrations of nanofillers were also efficacious against the streptococci but resulted in a deterioration of mechanical properties and a reduction in setting time [17]. Of course with these materials one would have to consider what intensity of UV light was necessary to activate the antimicrobial function of the titania – the patient is unlikely to want to spend most of their day with their mouth wide open, gathering the sun’s rays, and is certainly going to resist the suggestion of placing a UV light source in their mouth for therapeutic purposes. Perhaps if the patient had a class V restoration in the anterior region of the mouth this could be used as a motivation for them to put on a broad smile on their way out of the dentist’s surgery.
Other Nanofilled Materials
Nanoscale fillers have been incorporated into many other dental materials, with rather mixed results.
Nanoparticles of montmorillonite (a clay-forming mineral) have been incorporated into poly(methyl methacrylate) (PMMA), a denture base material (Chapter 3.2), and appear to have a good biocompatibility profile as investigated using laboratory and animal tests [18]. The inclusion of these nanoparticles confers a reduction in the polymerization shrinkage of the material [19] so may offer advantages in processing and fit. One might well expect that such inclusions would influence the mechanical and physical properties but this has yet to be established. PMMA has also been modified by addition of nanoscale (4–6 nm) particles of industrial diamond, and it was found that 0.1 wt % substitution of these nanoparticles made the material tougher and more resistant to impact, although higher substitutions caused these properties to deteriorate [20]. This team also noted a change in the colour of the nanofilled PMMA which may represent a limitation to this application.
A modified mineral trioxide aggregate (MTA), used for endodontic applications (Chapter 2.6), has been developed containing nanofillers (US patent http://www.google.com/patents/US20120012030). It seems that the nanofilled material exhibited increased hardness and a shorter setting time compared to a similar material with larger particle size, as well as a reduced porosity (Figure 2.8.1) [21]. The researchers noted that the nanofilled material exhibited a dimensional change on setting which they feared could lead to the material becoming dislodged, but a later comparison of the force needed to push a root filling of the nanofilled MTA out of position with that of conventional MTA materials suggested that the reverse was true and the adhesion of the nano-MTA was superior [22]. Only time will tell whether these properties offer significant clinical benefit, but the initial investigations are quite promising.
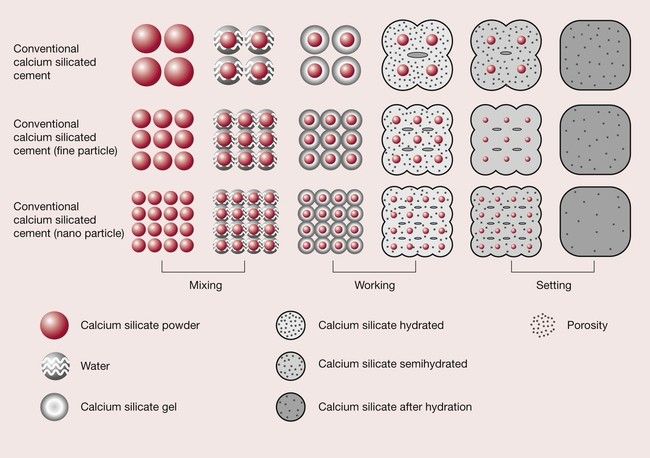
Silica nanofillers have been incorporated into polyvinylsiloxane impression materials (Chapter 2.7), and it was found that by carefully adjusting the ratio of nano- to traditionally sized silica fillers, properties such as the viscosity, tensile strength and working and setting time could be adjusted [23]. A good deal more research will be needed, though, before these materials are fully characterized.
As discussed in Chapter 3.6, zirconia is finding increasing application in dentistry, including in indirect restorations which are then veneered with a more translucent ceramic. These have been very welcome developments but the restorations are sometimes hampered by difficulty in machining the restoration without chipping the veneering ceramic. Using small zirconia particles in the processing phase can result in an increase in the strength of the finished material, but it is of paramount importance to ensure that the particles are well distributed rather than present in clumps and agglomerates, and this has confounded some attempts to use nanoscale zirconia. This has recently been achieved using diatomite (a porous silicate) coated with nanoparticles of zirconia, and the resultant materials had a higher flexural strength and a decreased porosity, although disappointingly there was no improvement in fracture toughness [24].
Thus nanofilled dental materials offer some tantalizing improvements in some properties, particularly the physical and mechanical, but these improvements are sometimes at the expense of other properties. There is room for a good deal of development in this field and this will surely come over the next few years, particularly as manufacturers become more acutely aware of the research being conducted in the academic environment.
Clinical Evaluation of Nanofilled Materials
Owing to the relatively recent development of most of these materials, there is not yet a great deal of clinical data available.
There is one report of non-carious cervical lesions filled using a nanofilled composite, a nanofilled and a traditional RMGIC, observing the restorations at baseline and 6 and 12 months’ recall [25]. The main conclusions of this study were that the nanofilled RMGIC exhibited more marginal staining and discoloration than the other materials. However, the materials were not matched for other properties and were of three different categories, so it is not possible to conclude that it was the nanofillers that were responsible for this difference or if it is simply a facet of the composition and structure of this particular RMGIC.
Another clinical study compared 49 class I and II restorations in 32 patients using a nanohybrid, a microfilled hybrid and a conventional hybrid composite [26]. This suffers from the same problem as the study described above, in that the materials were not matched for other properties and there is an incomplete data set describing the size, distribution and relative proportions of the different filler particles. This study has the benefit that restorations were assessed at regular intervals over 5 years, giving a longer view of the materials’ performance. Although the three materials performed very similarly overall, there was a slight but statistically significant reduction in wear for the nanofilled hybrid.
One further clinical study compared 68 class II restorations in 30 patients over a 6-year period using a nanohybrid and conventional hybrid material [27]. Suffering from the same confounding factors as the previous two studies, this study found no significant difference between the two materials in terms of a range of clinical criteria such as marginal integrity and development of defects. A study comparing indirect restorations created using a nanofilled composite and an ormocer could again detect no differences related to material choice at a 36-month recall [28].
Such clinical data can be confounded by the pace of development of dental biomaterials, which is so rapid that, once sufficient data has been gathered to describe the clinical efficacy of a given material, the material is virtually out of date and new generations of material have superseded it. We must of course continue to strive to establish the efficacy of nanofilled materials in a clinical environment but the reader should be aware that there is inevitably a degree of delay and consequent redundancy in some of this data.
Surface Engineering of Dental Implants
Dental implants are fabricated from titanium or, more rarely, titanium alloys such as Ti–6Al–4V where the titanium is alloyed with 6% aluminium and 4% vanadium. Titanium and its alloys exhibit the unusual property of osseointegration, whereby the interaction of the bone-forming cells, osteoblasts, with the implant surface results in a stable, strong, resilient interface that can withstand the rigours of chewing, eating and talking for a lifetime. Although osseointegration can happen with a smooth titanium surface, it is widely accepted that using an implant surface with moderate roughness accelerates and assists the process.
In years gone by the primary focus has been on the micrometre scale roughness, or microroughness, of implant surfaces. Implants have been developed with many different microrough surface finishes, such as those shown in Figure 2.8.2. Many interesting observations have been made, including that osteoblasts and other cells respond to the specific pattern and separation of features on the surface and will align themselves in grooves or pits of an appropriate size. More recently, the concept of nanoroughness has been developed and the response of osteoblasts to nanoscale features has become a topic of interest to researchers in the field of implantology. It is difficult to quantify the effect of nanoroughness on osseointegration since the methods used to alter the nanoroughness of an experimental fixture often simultaneously change the microroughness and/or the surface chemistry of the titanium, meaning that if the implant generates a different response one does not know whether it was the change in nanoroughness or in one of the other factors which caused it [29]. Very careful study design is necessary if we are to separate out the effects of nanoroughness and random and ordered nanoscale features from other factors such as microroughness and surface chemistry. The best that can be said at present is that a number of commercially available implants have shown enhanced osseointegration compared with their predecessors from the same manufacturers, and one factor that the new models have in common is increased nanoroughness [29]. This is tantalizing but only further investigations will give a conclusive answer on the importance of nanoroughness and nanoscale features in implant technology.
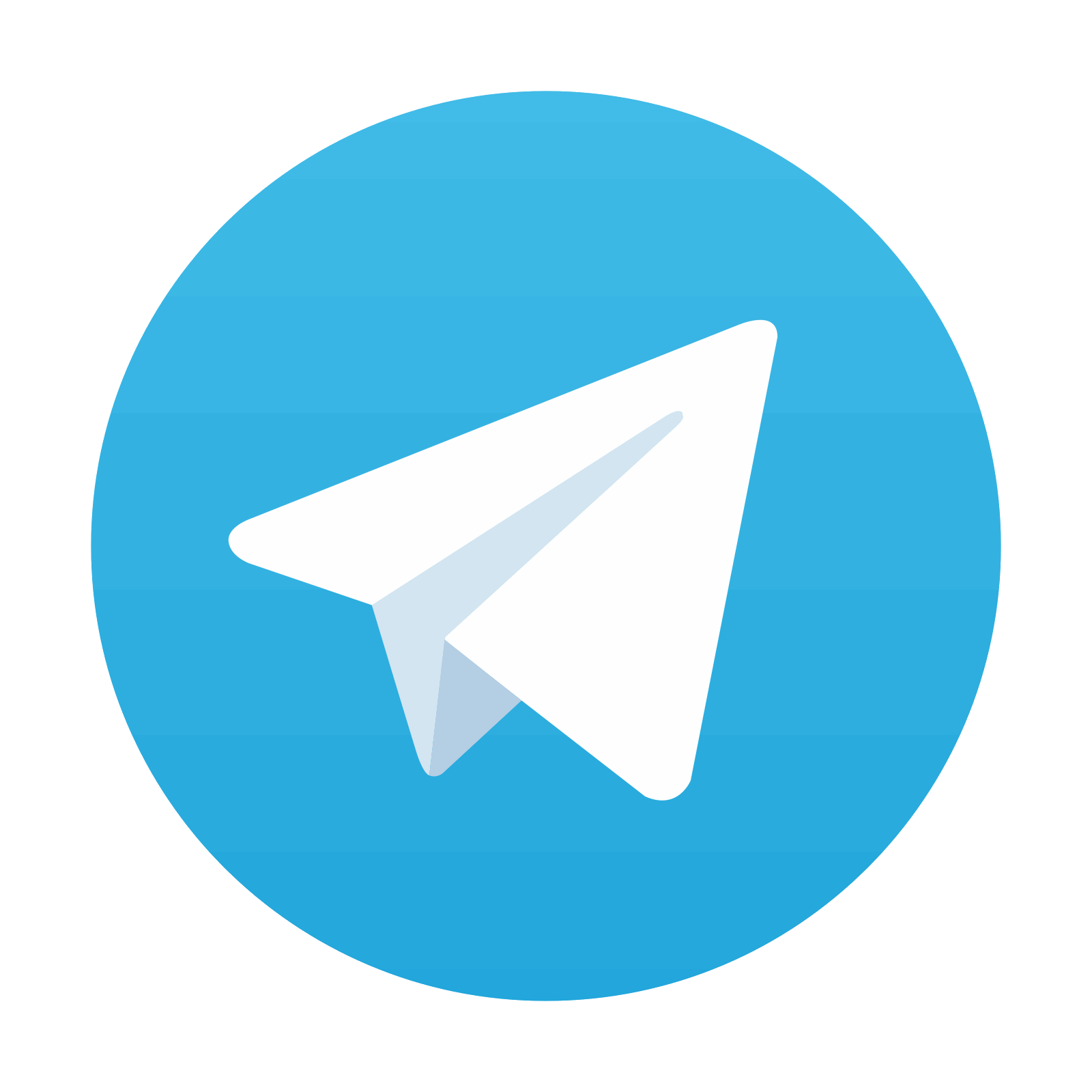
Stay updated, free dental videos. Join our Telegram channel

VIDEdental - Online dental courses
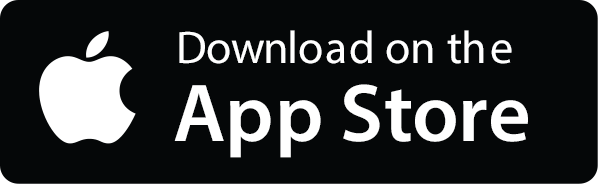
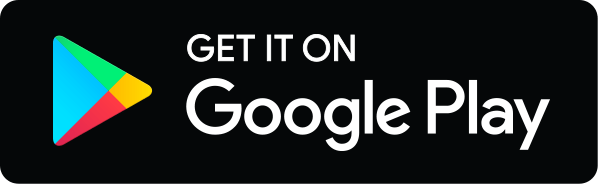