15
The structure of the central nervous system
Chapter contents
15.1 The braincase and cranial fossae
It is important to have a picture of the relationship of the brain and spinal cord to the bones of the skull and vertebral column that house and protect them and the protective layers of connective tissues known as the meninges that cover the CNS; these lie between the bones and brain and spinal cord.
The brain is housed within the skull which will be described in much more detail in Section 4. As you can appreciate by feeling your own skull, the top, front, sides, and back are smoothly curved. The surface of the brain is similarly curved and conforms to the shape of the bones. Note that, in reality, it is really the other way round—brain shape determines the shape of the bones of the skull vault forming the braincase (see Section 33.3). If the top of the braincase and the brain are removed to reveal the floor of the cranial cavity formed by the bones of the cranial base, it is anything but smooth. Viewed from the lateral aspect and going from anterior to posterior, it is like three descending steps. This structure is shown diagrammatically in Figure 15.1 and shows how different parts of the brain conform to these steps. The first step lies above the nasal and orbital cavities and is known as the anterior cranial fossa; it houses the frontal lobes of the cerebral hemispheres. The second step is the middle cranial fossa and contains the temporal lobes of each cerebral hemisphere laterally and the midbrain and pons medially. The final step is the posterior cranial fossa where the rest of the brainstem and cerebellum lie. The floor of the posterior fossa is pierced by the foramen magnum through which the medulla oblongata and spinal cord become continuous.
The spinal cord occupies the vertebral canal running in the vertebral column. As you can see in Figure 3.5, in adults, the cord occupies the vertebral canal from the upper border of the first cervical vertebra, the atlas, down to the level of the disc between the first and second lumbar vertebrae. The lower lumbar, sacral, and coccygeal spinal nerves travel down from their relevant spinal cord segments through the lower vertebral canal to exit from their respective intervertebral foramina. For example, the fifth lumbar segment of the spinal cord is at the level of the twelfth thoracic vertebra, fifth lumbar nerve; the roots of the fifth lumbar nerves, therefore, have to run down past all the lumbar vertebrae to their exit from the canal through the intervertebral foramen below the fifth lumbar vertebra. The lower spinal nerves form a bundle of nerves known as the cauda equina (Latin = horse’s tail). The narrow lower end of the cord is attached to the first coccygeal vertebra by a thin filament, the filum terminale. In the early fetus, the vertebral canal and spinal cord are virtually equal in length; the vertebral column elongates more rapidly than the cord during later fetal life and to a lesser extent, in childhood so the cord does not fill the full extent of the vertebral canal.
15.1.2 The meninges
The meninges are three layers of connective tissue that lie between the bone of the braincase and the external surface of the brain and continue through the foramen magnum to surround the spinal cord within the vertebral canal. Figure 15.2 shows the disposition of the three layers; follow the diagram as you read the description. The outermost layer, the dura mater, lies immediately adjacent to bone. The innermost layer, the pia mater, is intimately applied to the surface of the CNS. An intermediate layer or arachnoid mater lies between the dura and pia. (Although the full name of each layer consists of two words, ‘mater’ is frequently omitted in practice; the layers thus become the dura, arachnoid, and pia. We will follow this convention in the following descriptions.)
The dura (mater)
Technically, the dura consists of an outer endosteal layer and an inner meningeal layer. As Figure 15.2 demonstrates, these two layers are in fact united except where they separate to enclose the venous sinuses, specialized venous channels draining blood from the brain and meningeal blood vessels (see Section 15.1.6).
Fig. 15.1 The relationship of the brain to the cranial fossae of the skull.
Fig. 15.2 The meningeal layers and formation of venous sinuses.
The endosteal layer is the periosteum of the internal surfaces of the braincase and vertebral canal to which it tightly adheres. This layer continues into the foramina, carrying blood vessels and nerves into and out of the cranial cavity and it blends with the periosteum on the external surface of the braincase. The meningeal layer is tough and fibrous and gives the dura its characteristics. It forms tubular sheaths around the cranial and spinal nerves as they leave the cranial cavity and spinal canal which fuse with the epineurium of the nerves. As illustrated in Figure 15.2, the meningeal layer is reflected at certain points to form double-layered folds that project into the cranial cavity between different parts of the brain and help stabilize and protect the brain; they are described in Section 15.1.3.
The pia (mater)
The pia is a delicate layer of richly vascular connective tissue. The pia is represented in black in Figure 15.2. You can see how it closely covers the surface of the brain and spinal cord at all points; it enters even the deepest sulci and the walls of the ventricles of the brain to form the choroid plexuses that produce cerebrospinal fluid.
The arachnoid (mater)
The delicate arachnoid lies against the internal surface of the meningeal layer of the dura mater and the two are separated by the narrow subdural space that contains a thin film of tissue fluid. A wider gap separates the arachnoid and the pia. This is the subarachnoid space which is filled with cerebrospinal fluid. As illustrated in Figure 15.2, fine strands of arachnoid tissue extend across the subarachnoid space to the pia and look like a spider’s web; it is this likeness that gives the arachnoid its name (from the Greek = spider). The size of the subarachnoid space varies. It is relatively narrow over convexities of the brain and wider over fissures and other depressions. The subarachnoid space is widest on the underside of the brain in areas called the basal cisterns. If you have the chance to examine a hemisectioned head with the brain still in place, the arachnoid bridging the subarachnoid space is most clearly seen at the cerebellomedullary cistern occupying the angle between the cerebellum and the posterior surface of the medulla.
The arteries and veins of the brain travel within the subarachnoid space before entering or after leaving the brain tissue. As the cranial nerves cross the subarachnoid space, they pick up sheaths of pia and arachnoid. These sheaths extend as far as the exit of the nerves from the skull where they fuse with the epineurium.
The arachnoid herniates through the meningeal layer of the dura mater to protrude into the venous sinuses and venous lakes in certain areas. Small herniations are called arachnoid villi. The arachnoid granulations are considerably larger and may be seen with the naked eye if the superior sagittal sinus is opened. The villi and granulations are the structures through which cerebrospinal fluid is absorbed into the bloodstream (see p. 131)
15.1.3 Dural reflections
The two largest dural reflections can be seen in Figure 15.3 in which the right cerebral hemisphere and overlying bone have been removed. The falx cerebri is a double-layered fold of the dura attached along the mid-line inside the cranial vault where it is continuous with the meningeal layer of the dura. Study Figure 15.3 to understand its general shape, its attachments to the skull, and its relationship to venous sinuses. It is attached anteriorly to the crista galli, a short bony process above the nasal cavity, and posteriorly it attaches to the superior surface of the tentorium cerebelli. The two leaves forming the falx separate and blend on each side with the tentorium cerebelli to enclose the straight sinus. The falx is, therefore, relatively narrow anteriorly and broadens out posteriorly, resembling the shape of a sickle (hence its name from the Latin falx = sickle). At the convex superior attachment, the two leaves of the falx cerebri are separated to form the superior sagittal venous sinus. The free concave lower border of the falx contains the inferior sagittal venous sinus. The two leaves of the falx are firmly united between the superior and inferior sagittal sinuses to form a strong, inelastic membrane that extends into the longitudinal fissure between the two cerebral hemispheres. The falx, therefore, is ideally positioned to prevent excess movement of the brain if the head is subject to violent turning movements.
Fig. 15.3 Dural reflections and formation of venous sinuses.
As you can see in Figure 15.3, the tentorium cerebelli (Latin = roof (or tent) over the cerebellum) lies in the horizontal plane at right angles to the falx cerebri. The position and attachments of the tentorium is much more easily appreciated when viewed from directly above as in Figure 15.4 which should be studied as the following description is read. Its two leaves separate on each side to blend with the meningeal dura on the inner aspects of the occipital and parietal bones, enclosing the transverse venous sinus between them. The course of the transverse sinuses is marked by prominent grooves in these bones. Anteriorly, the tentorium is attached to the posterior superior border of the petrous temporal bones; the superior petrosal sinuses are found at these attachments. The anterior attachments extend to just behind the pituitary fossa. Figure 15.4 shows how this arrangement creates short, concave, free inner margins, enclosing an opening occupied by the midbrain. The tentorium projects into the cranial cavity above the cerebellum and below the occipital lobes of the cerebral hemispheres. It is well placed to prevent movement of the brain when the head is moved violently forwards and backwards as in nodding the head.
Near the apex of the petrous bone, the lower layer of the tentorium continues forwards to form a small outpouching between the endosteal and meningeal layers of the dura of the middle cranial fossa. This is the trigeminal (Meckel’s) cave that contains the roots and the sensory ganglion of the trigeminal nerve.
The falx cerebelli is a small curved fold of dura mater which projects into the posterior notch of the cerebellum below the tentorium.
Figure 15.4 illustrates a circular fold of dura, the diaphragma sellae, which forms the roof of the pituitary fossa in the sphenoid bone. The diaphragm of dura completely covers the pituitary gland, apart from a small central opening for the pituitary stalk.
15.1.4 Meninges of the spinal cord
The arrangement of the meninges enclosing the spinal cord is illustrated in Figure 15.5. The spinal dura forms the external layer and lies close to the periosteum lining the vertebral canal. The extradural (or epidural) space between them contains some adipose tissue and a venous plexus. The dura ensheathes the roots of the spinal nerves as they pass through the intervertebral foramina, then fuses with the covering epineurium.
The spinal arachnoid mater lies against the inner surface of the dura. The pia mater closely invests the spinal cord and surfaces of the roots of the spinal nerves. There is a substantial subarachnoid space between the pia and the arachnoid containing cerebrospinal fluid, with strands of arachnoid bridging the gap. The spinal cord is suspended within the subarachnoid space by the denticulate ligaments shown in Figure 15.6. These are linear structures which run from the upper cervical to the lumbar region on each side of the cord. They extend from the pia to the dura between the exits of successive spinal nerves. Tooth-like projections extend into the interval between the dorsal and ventral roots of the spinal nerves.
Fig. 15.4 The tentorium cerebelli and associated venous sinuses from above.
Fig. 15.5 A cross section of the spinal cord and covering meninges.
Fig. 15.6 The denticulate ligaments of the spinal cord. The dura mater dorsal to the cord has been removed.
The consequences of meningeal infections are described in Box 15.1.
Box 15.1 Infections of the meninges
Inflammation of the pia and arachnoid mater is called meningitis and usually involves spinal as well as cerebral meninges. It is most frequently caused by bloodborne infections from microorganisms; infective organisms may also spread from a brain abscess or be introduced through a penetrating wound. The outstanding features of meningitis are headache, fever, rash, and the signs of spinal meningeal irritation (neck rigidity). Some of the organisms that cause meningitis are extremely virulent; meningitis can have an extremely rapid onset and may quickly become fatal unless diagnosed and treated urgently.
15.1.5 Nerve and blood supply of the dura mater
The dura of the anterior cranial fossa are supplied by branches of the ophthalmic divisions of the trigeminal nerves; meningeal branches of the maxillary and mandibular divisions of the trigeminal nerves supply the middle cranial fossa. The dura of the posterior cranial fossa is innervated by ascending branches from the upper cervical spinal nerves entering the cranial cavity through the foramen magnum. The meningeal nerves contain sensory and post-ganglionic sympathetic neurons. The latter may have a vasomotor function and have been implicated in migraine attacks.
The arteries to the dura mater mostly supply the endosteal layer. The principal supply to the dura above the tentorium cerebelli is through the middle meningeal arteries which are branches of the maxillary arteries (see Section 24.5.2). They enter the cranial cavity through the foramen spinosum on each side and run laterally across the floor of the middle cranial fossa between the endosteal and meningeal layers of the dura and divide into anterior and posterior branches; they groove the bones that they cross. The subtentorial dura is supplied by meningeal branches of the vertebral arteries (see p. 135). The effects of blood loss into the meninges are outlined in Box 15.2.
15.1.6 The venous sinuses
The venous sinuses have been referred to several times as they are formed between the endosteal and meningeal layers of the dura adjacent to bone and its reflections. The venous sinuses are wide venous channels draining blood from the brain, the bones of the braincase, and the meninges. The sinus walls are tough sheets of dura with no muscular tissue or valves lined by endothelium like any other blood vessel. Furthermore, they are held patent at all times because of their construction; the sinuses will, therefore, not collapse when blood pressure falls, e.g. in shock, meaning that perfusion of the brain should continue.
The arrangement of the venous sinuses can best be pictured by studying Figure 15.7 in conjunction with Figure 15.3 and 15.4.
The superior sagittal sinus receives blood from superior cerebral veins draining blood from the superior, lateral, and medial surfaces of the cerebral hemispheres. Venous blood drains backwards to the back of the skull. Figure 15.3 shows what happens at the point where the straight sinus and the superior sagittal sinus meet at a point marked by a slight bulge of bone, the internal occipital protuberance. The superior sagittal sinus usually turns to the right, but occasionally to the left, to become the transverse sinus of that side.
As illustrated in Figure 15.3, the inferior sagittal sinus running in the inferior free margin of the falx cerebri receives blood from the falx and ends by opening into the straight sinus formed where the falx attaches to the tentorium cerebella. The straight sinus drains the inferior sagittal sinus and also receives blood from the great cerebral vein which drains the deep parts of the cerebral hemispheres. Figure 15.4 shows that the sinus ends at the internal occipital protuberance by turning to become, in most cases, the left transverse sinus.
The transverse sinuses pass forward until they reach the junction of the petrous and mastoid parts of the temporal bone where they curve downwards to become the sigmoid sinuses. The sigmoid sinuses have a shallow S shape, seen most clearly in Figure 15.7, as they continue downwards and forwards to the jugular foramina. They deeply groove the endocranial surface of the mastoid part of the temporal bone. The sigmoid sinuses expand to form the jugular bulbs in the posterior part of the jugular foramen; these are continuous with the internal jugular veins below.
Box 15.2 Extradural and subarachnoid haemorrhage
Intracranial haemorrhage may occur either within or outside the brain. Intracranial haemorrhage occurring outside the brain may occur in the extradural, subdural, or subarachnoid space. The effects of haemorrhages inside the brain are described on in Box 15.10.
The extradural space is a potential space, located between the fused endosteal and meningeal layers of the dura mater. Haemorrhage into this space—extradural haemorrhage—is relatively uncommon and is usually the result of tearing of meningeal vessels or venous sinuses consequent upon a fracture of or heavy blows to the braincase. The meningeal vessels are particularly vulnerable to blows to the temple because the bone is relatively thin.
The subdural space is another potential space between the meningeal layer of the dura and the arachnoid mater. Bleeding into this space (subdural haemorrhage) is more common, usually due to tearing of one or more of the superior cerebral veins at the point where they enter the superior sagittal sinus. Although the cerebral veins run for most of their extracerebral course in the subarachnoid space, they cross the subdural space to reach the venous sinuses. In blows to the head, the brain may be suddenly moved enough to tear the cerebral veins near where they are fixed where they pass through the dura mater. Blood then enters the subdural space.
Extradural and subdural haemorrhages result in raised intracranial pressure (ICP). The principal clinical features are a consequence of brain compression caused by the raised ICP. The rate of onset is extremely variable. Essentially, blood forces its way into a very limited space to produce localized, but extremely intense, pressure which raises the ICP quite rapidly. This, in turn, results in ‘coning’, the compression of the medulla as it is displaced into the foramen magnum; coning compromises the functions of the cardiovascular and respiratory centres of the medulla with life-threatening consequences (see Box 3.4).
The subarachnoid space is a real space occupied by cerebrospinal fluid. Blood has room to spread when vessels haemorrhage into this space (a subarachnoid haemorrhage). Subarachnoid haemorrhages may follow the rupture of a congenital aneurysm of one or other of the arteries comprising the arterial circle (see Section 15.5.1) or the rupture of cerebral veins by a blow to the head with consequent bleeding into the subarachnoid space. Although ICP will rise eventually, the principal clinical features of subarachnoid haemorrhage are those of meningeal irritation described above because extravasated blood is a powerful irritant of neuronal tissues.
Fig. 15.7 The venous sinuses seen from above after removal of the tentorium cerebelli.
15.1.7 The cavernous sinuses
Two important sinuses have not been encountered yet. These are the right and left cavernous sinuses on each side of the body of the sphenoid shown in Figure 15.7. If they are viewed in cross section, numerous fibrous partitions cross their cavities, partially dividing them into a series of small caverns—hence the name for these structures. Like most of the other sinuses, they lie between the endosteal and meningeal layers of the dura. The endosteal layer covers the lateral aspects of the body of the sphenoid as might be expected from the position of the cavernous sinuses. The meningeal dura extends laterally from the diaphragma sellae covering the pituitary fossa and then descends to the floor of the middle cranial fossa, forming the roof and lateral walls of the sinuses. Several important structures pass through or along the walls of the sinuses:
• The internal carotid arteries (see Section 15.6.1);
• The third, fourth, and sixth cranial nerves supplying the extraocular muscles that move the eyes (see Chapter 18);
Box 15.3 The venous sinuses and infection
The venous sinuses drain the cranial cavity whereas the structures in the head and neck lying outside the cranial cavity drain into conventional veins. The skin and immediately underlying tissues drain into superficial veins whereas deeper structures drain into the deep veins. The superficial and deep drainage of the head and neck will be described more fully in Section 23.2.2.
It is important to realize that the superficial, deep, and venous sinus components of the venous drainage are all interconnected. These connections can be a route for the spread of infection which can be particularly serious if it enters the cranial cavity where the meninges or venous sinuses may be infected. The cavernous sinus in particular has numerous connections with extracranial veins. The orbit drains through ophthalmic veins into the cavernous sinuses posteriorly, but also into the venous drainage of the face anteriorly; the two routes are connected. The ophthalmic veins also communicate with the pterygoid plexus of veins before entering the sinus; the pterygoid plexus receives venous blood from deep structures in and around both jaws, including most of the dental structures (see Section 24.5.3). Infection may thus spread from the face or from structures drained by the pterygoid plexuses into the cavernous sinuses. Blood flow through the cavernous sinuses is relatively slow because they are partitioned into small interconnected cavities, making it more prone to clot, producing an infected thrombosis. Thrombosis in the cavernous sinuses may produce a retrograde thrombosis into one of the middle cerebral veins that drain deep parts of the brain; retrograde thrombosis is usually fatal. It will also affect the cranial nerves passing through the cavernous sinus, leading to defective eye movements (see Box 18.3).
• The ophthalmic and maxillary divisions of the trigeminal nerve (see Chapter 18).
The cavernous sinuses receive blood from the lateral and inferior surfaces of the cerebral hemispheres and the ophthalmic veins from the orbit and drain via the superior and inferior petrosal sinuses that exit from the posterior part of the cavernous sinuses on each side (Figure 15.7). Each superior sinus runs along the superior border of the petrous temporal bone to enter the junction of the transverse and sigmoid sinuses. Each inferior sinus runs more or less directly downwards into the jugular foramen.
The potential spread of infection through venous sinuses and its consequences are described in Box 15.3.
15.2 The spinal cord
The spinal cord is oval in cross section, being flattened dorsoventrally as outlined in Figure 15.8. There is a general reduction in diameter from the cervical to the coccygeal region, but there are two enlargements where the thick spinal nerves supplying the limbs emerge. The cervical enlargement in the lower cervical and upper thoracic region is where the nerves forming the brachial plexus innervating the upper limb arise. The lumbar enlargement is the area where lumbar and sacral nerves form the lumbar and sacral plexuses to supply the lower limbs.
The external surface of the cord is shown from the dorsal aspect in Figure 15.6. A shallow dorsal median sulcus marks the midline and there are shallow dorsolateral sulci laterally. Figure 15.8 indicates the corresponding indentations on the ventral aspect; the ventral median fissure is obvious, but the ventrolateral sulci are shallow. Thirty-one bilaterally paired spinal nerves—eight cervical, 12 thoracic, five lumbar, five sacral, and one coccygeal—are attached to the cord. Each nerve is formed by the fusion of a dorsal and ventral root. Each root is formed from the confluence of a series of rootlets entering or leaving the corresponding segment of the cord. Dorsal roots exit along the dorsolateral sulcus (see Figure 15.6) and ventral roots along the ventrolateral sulcus. The structure of spinal nerves has already been described in Chapter 3; their relationship to the spinal cord and vertebrae has also been covered above and illustrated in Figure 3.5.
15.2.1 Internal structure
As shown in Figure 15.6 and 15.8, there is a clear distinction between the grey and white matter in a transverse section of the spinal cord which can be seen with the naked eye on anatomical preparations of the spinal cord. The grey matter is H-shaped in transverse section and forms a continuous mass throughout the length of the spinal cord; grey matter contains neuronal cell bodies, dendrites, and synapses. The crossbar of the H is the central commissure formed by neuronal processes connecting the right and left halves of the spinal cord. Look carefully at Figure 15.8 and you will see a narrow central canal centrally in the commissure; the canal is continuous with the ventricular system of the brain.
The cauda equina is surrounded by subarachnoid space. When a sample of cerebrospinal fluid is required for examination, it is usually taken from this region by means of a lumbar puncture. A needle is inserted between the arches of the third and fourth lumbar vertebrae and advanced until its tip enters the subarachnoid space. Inserting the needle at this level avoids any possibility of damaging the spinal cord and the nerves forming the cauda equina will float away from the needle. The same site is used for epidural injections that may be used to alleviate pain during childbirth.
Fig. 15.8 Transverse sections of the spinal cord in the cervical (above), thoracic (middle), and lumbar (below) regions.
The grey matter
The dorsal and ventral horns of the grey matter project dorsally and ventrally on each side. The dorsal and ventral horns form continuous columns throughout the length of the spinal cord. Figure 15.8 also indicates the position of the small intermediolateral (or lateral) horns between the junction of the dorsal and ventral horns on each side; they only extend from the upper thoracic to the upper lumbar region. As already described in Chapter 3, the dorsal horns are the site of termination of sensory neuronal processes and the ventral horns are the location of the cell bodies of motor nerves and the origin of their axons. The lateral horns are the site of the cell bodies of preganglionic sympathetic axons distributed via the ventral roots of the thoracic and upper lumbar spinal nerves.
The grey matter within the dorsal, ventral, and intermediolateral horns can be subdivided microscopically into layers (the laminae of Rexed) on the basis of the cell types and density that constitute each layer. These laminae are shown in Figure 15.9. Laminae I to III from the tips of the dorsal horns inwards are where many incoming primary sensory neurons terminate; neurons terminating in these laminae carry mainly temperature and nociceptive information. Laminae IV to VI, forming the deeper part of the dorsal horns, contain the cell bodies of secondary thalamic projection neurons. The primary and thalamic projection neurons have to be connected to form efficient ascending sensory pathways. This is achieved by interneurons connecting different layers within the dorsal horns. In addition, many thalamic projection neurons have comparatively long dendrites that extend from their cell bodies in the deeper laminae to synapse with the axons of primary sensory neurons entering the upper layers (see Section 16.2).
Fig. 15.9 A transverse section of the spinal cord in the thoracic region showing the laminae of the grey matter.
Lamina VII is, by far, the biggest layer. It contains numerous interneurons, but also two important columns of neurons. The intermediolateral cell column contains the cell bodies of autonomic preganglionic neurons. The nucleus dorsalis or Clarke’s column is the site where primary proprioceptive neurons synapse with neurons whose axons form the spinocerebellar tracts. Lamina VIII forms the major part of the ventral horns and encloses many groups of large lower motor neuron cell bodies; these groups constitute lamina IX. Lamina VIII is the area in which descending motor pathways synapse with the dendrites of lower motor neurons found in lamina IX. Lamina X is the central commissure.
The white matter
The white matter consists principally of nerve axons and neuroglia. Figure 15.8 shows its arrangement into three columns on each side incompletely separated by the dorsal and ventral horns. The dorsal columns lie between the dorsal grey horn and the posterior median septum, the lateral columns are between the dorsal and ventral horns, and the ventral columns are between the ventral horns. Note from Figure 15.8 that there is no clear demarcation between lateral and ventral columns anteriorly.
Neuronal processes forming the white matter are aggregated into microscopically distinct tracts with separate functions. Such tracts may be ascending sensory tracts connecting the spinal cord to the brain or descending motor pathways connecting the brain to the cord; other tracts connect different segments of the spinal cord. The distribution and function of these tracts is described in Chapter 16.
15.3 The brainstem
The brainstem is continuous with the spinal cord and contains the upward continuations of many of the structures present in the cord or their equivalents. All the major tracts in the white columns of the spinal cord run through at least part of the brainstem to reach their destinations.
The principal differences between the brainstem and the spinal cord are:
• The grey matter in the brainstem is broken up into discrete nuclei related to the origins of each pair of cranial nerves whereas it forms a single continuous mass in the spinal cord;
• The central canal, which is narrow throughout the cord, is greatly expanded in the middle region of the brainstem to form the fourth ventricle;
• The cranial nerves emerge from the brainstem in a less regular pattern than that in which the spinal nerves leave the cord because the proportion of motor, sensory, and autonomic components differ radically in different cranial nerves;
• The cerebellum is attached to the posterior aspect of the brainstem by three pairs of peduncles.
The brainstem is divided into three parts. As shown in Figure 15.10, they are, in ascending order, the medulla oblongata, pons, and midbrain. The brainstem lies on the basilar part of the occipital bone and the body of the sphenoid anteriorly; the cerebellum is posterior. Revisit Figure 15.1 to recall that the medulla and pons are situated in the posterior cranial fossa and the midbrain lies in the middle cranial fossa. As outlined in Figure 15.11, the fourth ventricle is located posterior to the upper part of the medulla and the lower pons. The fourth ventricle narrows above to enter the cerebral aqueduct as it passes through the midbrain to the third ventricle in the cerebrum.
To place the fourth ventricle and cerebral aqueduct in context, the ventricles are a series of chambers within the brain in which cerebrospinal fluid (CSF) is formed and circulates. The first three ventricles are in the cerebrum and connect to the fourth ventricle in the brainstem by the cerebral aqueduct. The fourth ventricle is continuous below with the central canal of the spinal cord. CSF leaves the ventricular system to surround the brain through foramina in the lining of the fourth ventricle. Eventually, CSF is resorbed back into the intracranial venous system. The ventricular system is described more fully on p. 131.
If you turn Figure 15.10 upside down, the pons and medulla together look like a flower bulb, with the pons forming the bulb and the medulla being the shoot. This resemblance accounts for the old name for the brainstem, the bulb. The tr/>
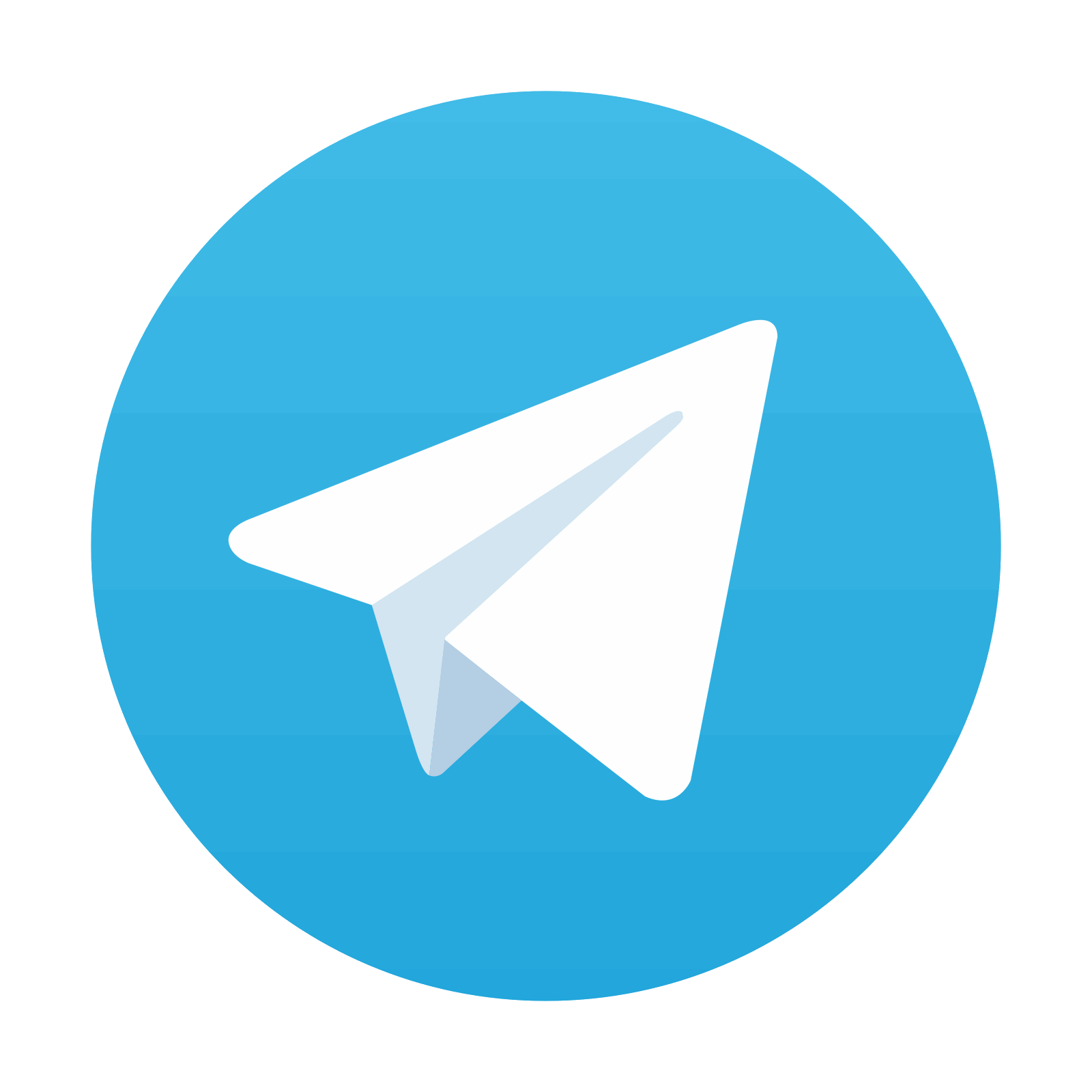
Stay updated, free dental videos. Join our Telegram channel

VIDEdental - Online dental courses
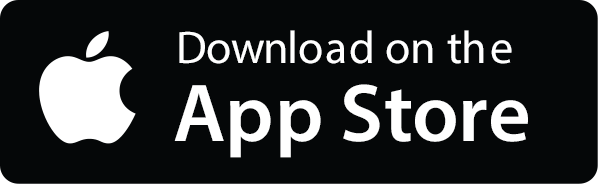
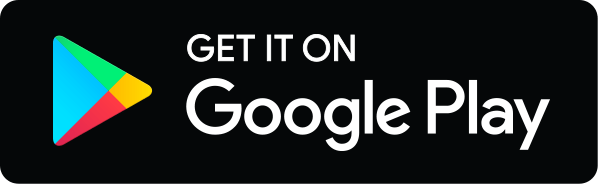