Occlusion: The Role in Implant Prosthodontics
Avinash S. Bidra and Thomas D. Taylor
University of Connecticut School of Dental Medicine, Farmington, Connecticut, USA
Introduction
It is well recognized that occlusion is one of the most controversial aspects of implant prosthodontics.1 This is because occlusion in implant prosthodontics has historically evolved through a combination of dogma, expert opinion, in vitro studies, animal studies, and extrapolation from concepts used on natural teeth. Interestingly, little scientific evidence exists in the field of occlusion to justify one concept over another. In an extensive review of the occlusion literature, Taylor et al.1 concluded that there is minimal scientific evidence to support a direct cause–effect relationship between occlusal factors and deleterious biological outcomes for osseointegrated implants. The purpose of this chapter is to examine various occlusal considerations and disconsiderations for implant‐borne restorations, scrutinize the available and applicable scientific evidence, and lend clarity on this topic to the reader. Recommendations on choice of occlusal schemes are also presented to the reader to simplify and aid in clinical practice.
Occlusal considerations for implant‐borne restorations
Crown–implant ratio
The concept of crown–implant ratio has been directly derived from the concept of crown–root ratio described in natural teeth. In natural teeth, crown–root ratio is regarded as an important aspect of diagnosis, treatment planning, and predicting the prognosis of treatment.2 A favorable crown–root ratio is 1:1.5, while the minimal accepted ratio is 1:1. While the scientific merits to this approach are debatable for natural teeth, it is important to understand that implants do not have the same characteristics as roots of natural teeth, nor can they be subjected to the same principles and rules.3 A greater crown–implant ratio has been considered to be detrimental by previous authors.4 However, a recent systematic review that investigated the influence of the crown–implant ratio on the success rate and marginal bone loss of dental implants showed that the higher the crown–implant ratio, the lesser the peri‐implant marginal bone loss.5 There was a significant negative association between the two variables, indicating that a higher crown–implant ratio had a beneficial effect on implants. In implant‐borne restorations, crown–implant ratio is merely theoretical, as the height of a crown is dictated by esthetics and distance from the implant platform to the occlusal plane (Figure 14.1). The length of an implant is primarily dictated by the ability to gain primary stability and anatomic limitations (Figure 14.2). Multiple systematic reviews on short implants have shown high survival rates and marginal bone loss comparable to regular‐length implants.5–7 Therefore, once osseointegrated, a short implant is as efficacious as a long implant, and, within the scope of dental occlusion, clinicians may simply ignore the concept of crown–implant ratio as far as protecting the bone adjacent to an implant is concerned. However an increased crown–implant ratio may increase the risk of mechanical complications related to the implant components themselves. One method to reduce mechanical complications in such scenarios is to use an implant design that offers an internal connection for the abutment, whilst the restoration is supported by the implant pillar itself. This concept is similar to the ferrule effect concept used in restoring endodontically treated teeth with dowel and core restorations.
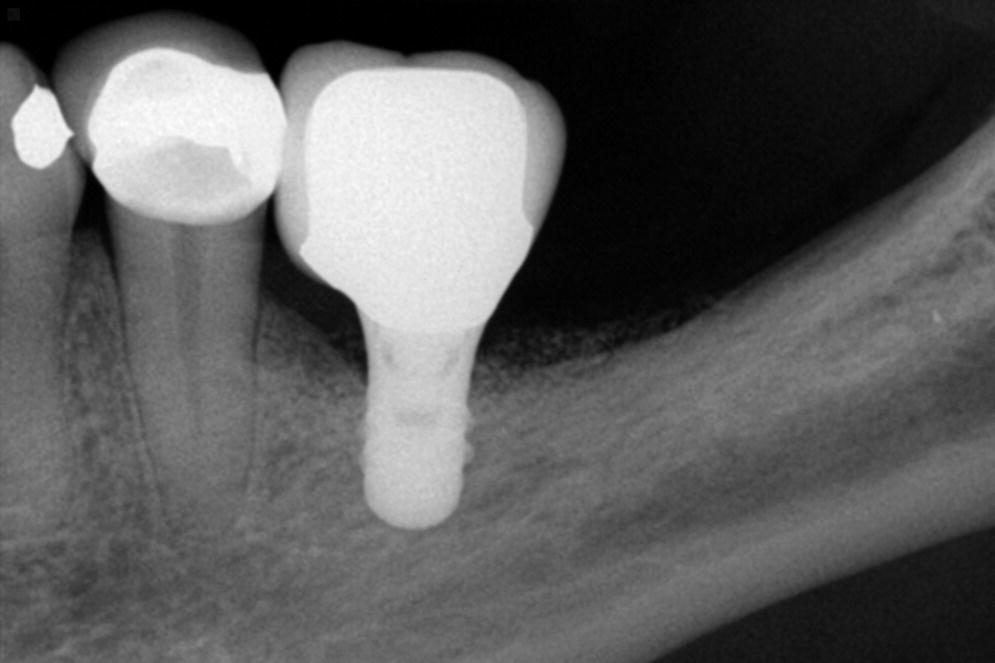
Figure 14.1 Periapical radiograph of a long‐term successful outcome from a short implant supported single crown with excessive crown–implant ratio. Notice that the veneering porcelain on the occlusal surface is well supported by the metal coping.
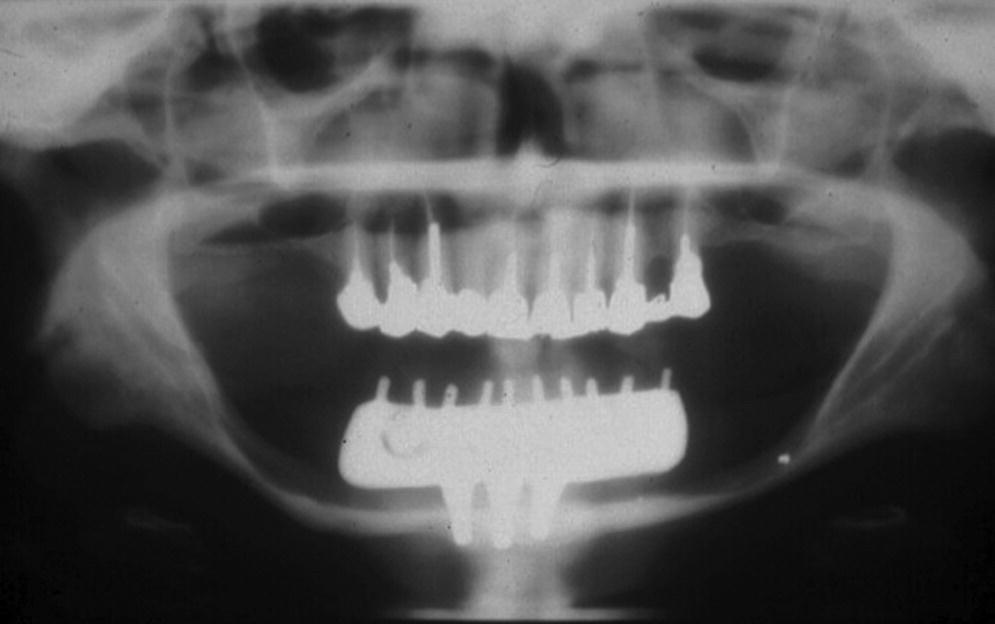
Figure 14.2 Panoramic radiograph of a long‐term successful outcome of a mandibular fixed prosthesis with bilateral cantilevers, supported by only three short implants. The implant length was dictated by the height of the residual mandible that was severely resorbed.
Progressive loading
Progressive loading has been defined as the “concept of gradually increasing the amount of functional load applied to a newly integrated dental implant or implants, by modifying the design and the material of the prosthesis.”8 Misch3,9 has described that progressive loading can be attained by the practice of increasing occlusal load over a time period of 6 months, and thus provide the requisite time for bone to adapt to loading by a gradual enhancement of loading. Accompanying tenets to this philosophy include using acrylic resin provisional restorations for an extended period of time during “bone adaptation”, keeping the implant‐borne restoration out of occlusion, and using shallower cusped teeth and a narrower occlusal table in the final restorations. While some of these tenets may be beneficial in patients with bruxism, or in immediate‐loading situations, primarily due to risk management and the ease of adjustment, the scientific basis for progressive loading in osseointegrated implants is questionable.
Stegaroiu et al.10 have shown that under static and nonimpact dynamic loading, three different types of superstructure materials tested (highly filled composite resin, acrylic resin, and gold alloy) had the same influence on the strain transmitted to a bone simulant that surrounded a single implant. Other authors have shown similar results, implying that keeping patients in provisional restorations solely for the purpose of progressive loading is a misconception.11 The laws of physics state that one cannot make mechanical energy disappear simply by changing the material through which a force is applied. Stated another way, a pound applied to the occlusal surface of an implant‐supported restoration is a pound transferred through the implant to the surrounding bone, regardless of the material the restoration is made of. With regards to using shallower cusps and reduced width of occlusal table, Morneburg et al.12 have shown that cusp height had no effect in reducing the load on implants. However, severely reducing the occlusal table width by 30% (which is clinically not possible without compromising esthetics and function) did reduce bending forces. This again questions the theory behind progressive loading. Finally, keeping the provisional restorations out of occlusion (or in “progressively light occlusion”) during maximum intercuspation is not advisable, because opposing natural teeth may supra‐erupt to contact the provisional restoration and disrupt the occlusal plane. Additionally, it is well known that during natural chewing, the food itself acts as a force amplifier, negating the effect of “progressively light occlusion”. Therefore, within the scope of dental occlusion, clinicians may ignore the concept of progressive loading in osseointegrated implants.
Occlusal overload
Occlusal overload has been defined as the “application of occlusal loading, through function or parafunction, in excess of what the prosthesis, implant component, or osseointegrated interface is capable of withstanding without structural or biologic damage.”8 To expound the implications of occlusal overload, it is important to first understand Wolff’s Law. Wolff’s law is defined as “principle of bone healing and/or remodeling based upon the understanding that bone remodels in response to physical stress by depositing bone in locations of increased stress and resorbing bone in areas of little or no stress.”8 It is named after German anatomist Julius Wolff, who first explained this concept. It is widely believed that occlusal overload can cause implant failure by failure of osseointegration, but interestingly the scientific evidence for this concept is lacking. Multiple animal studies have all demonstrated that osseointegrated implants are extremely resistant to occlusal overload.13–17
Contrarily, occlusal overload has been shown to promote new dense bone formation around the osseointegrated implants.17,18 There are no clinical studies on occlusal overload that have demonstrated a cause‐and‐effect relationship between occlusal overload and loss of osseointegration. One animal study by Isidor et al.,15 done on monkeys, demonstrated loss of implants when they were subjected to an unusually extreme loading environment, beyond what can be considered to be clinically relevant. In a recent systematic review by Chang et al.,19 the authors concluded that there was no scientific evidence to clarify whether occlusal overload may cause marginal bone loss or complete loss of osseointegration when the applied load exceeds the biologically acceptable limit. The authors also stated that this biological limit is presently unknown.19 Therefore, current scientific evidence does not demonstrate occlusal forces as being a substantial risk factor for the biological aspect of implant‐borne restorations. However, it is important to consider the unfavorable outcomes of occlusal overload on the mechanical aspect of implant‐borne restorations. The consequences of these detrimental effects may include fractured implants, fractured abutments, fractured occlusal screws, and, more commonly, fractured veneering resin or porcelain. Of these, fracture of the implant is obviously the most catastrophic complication (Figures 14.3 and 14.4). It is often difficult for clinicians to diagnose (before starting treatment) which patient may have higher occlusal loading and which patient may have parafunctional habits that are detrimental (Figures 14.5 and 14.6). Clinicians should recognize that the osseointegrated interface appears to be very strong and able to withstand occlusal overload within the limits of component structural integrity and appears to be the strongest link in the pillar of implant‐borne restorations.
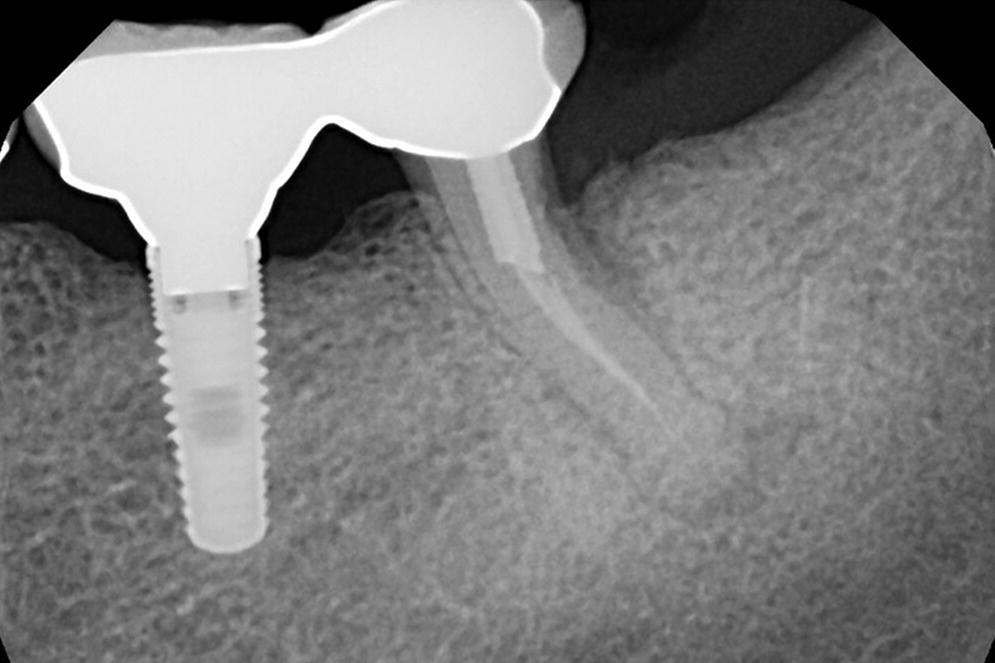
Figure 14.3 Periapical radiograph of a patient with an implant restoration splinted to natural tooth. This patient presented with pain and discomfort that was attributed to the failing natural tooth.
Source: reproduced with permission from Dr. Marie Falcone, DMD.
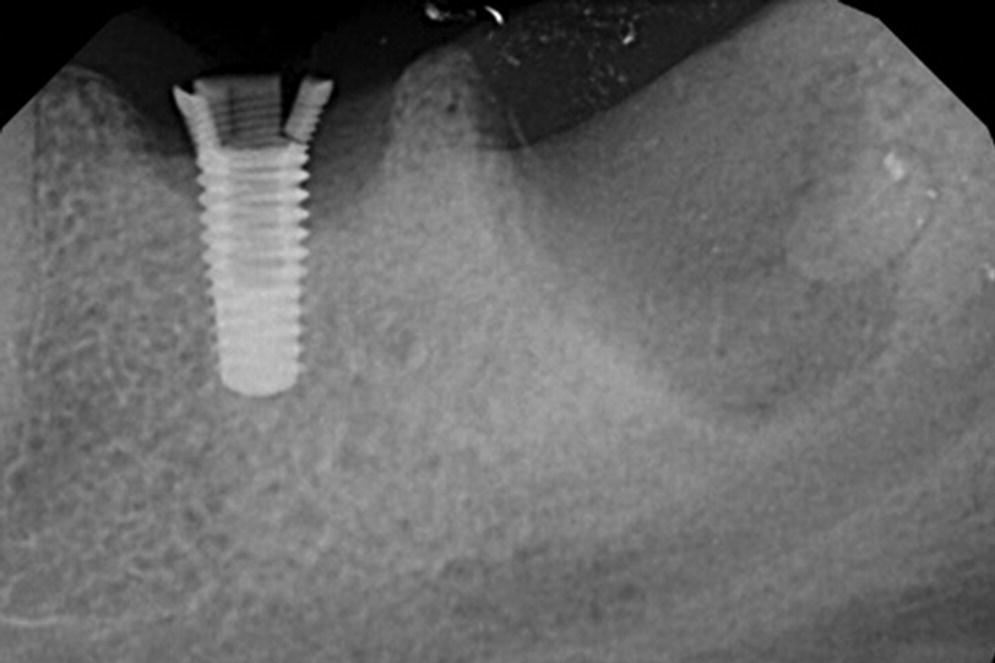
Figure 14.4 Periapical radiograph of patient in Figure 14.3 after removal of the restoration and extraction of the natural tooth indicates a fractured implant. This is a classic appearance of fracture of an internal‐connection implant caused by hoop stress.
Source: reproduced with permission from Dr. Marie Falcone, DMD.
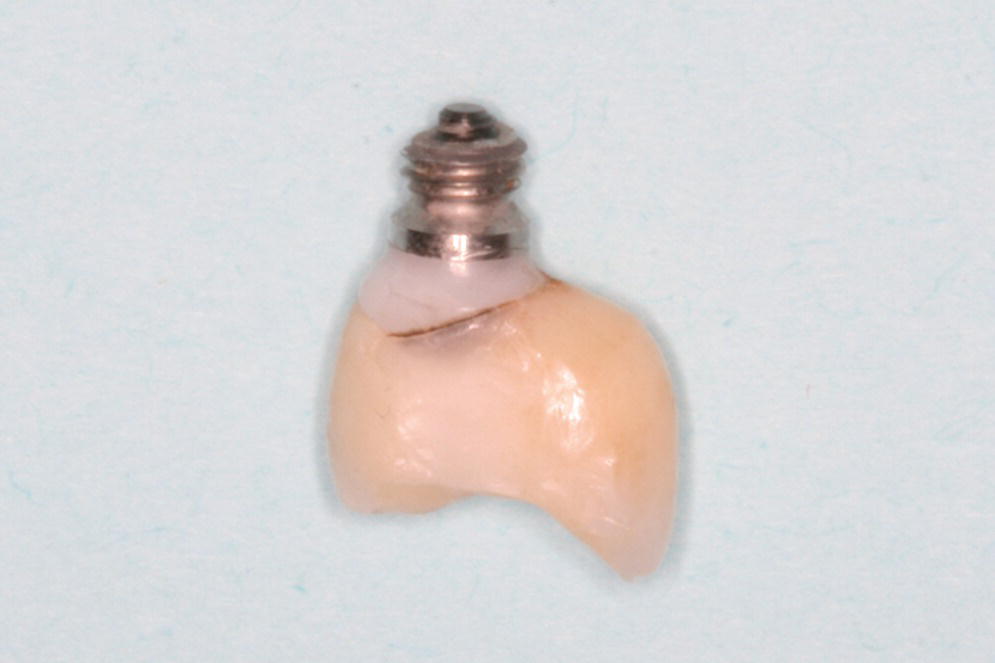
Figure 14.5 Clinical presentation of a fractured implant, which supported a single crown in the maxillary left second premolar region, from a bruxism patient.
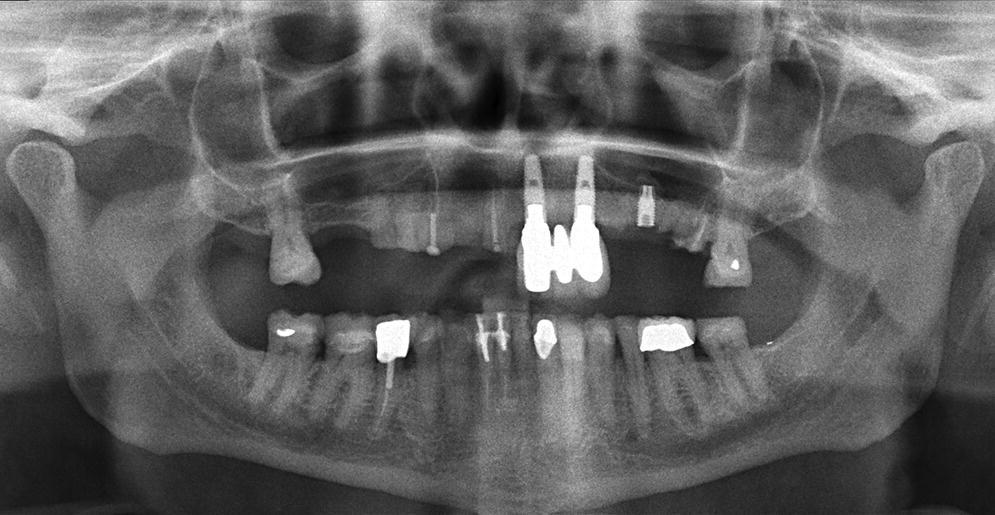
Figure 14.6 Panoramic radiograph of the bruxism patient from Figure 14.5 showing the extent of the fractured implant. Also notice multiple fractured teeth, fractured restorations, multiple missing teeth, as well as an acute gonial angle.
Nonaxial loading
Nonaxial loading refers to “loading of an implant body that is not along the long axis of an implant body,”8 whereas axial loading refers to “application of load, usually by the forces of occlusion, in the direction of the long axis of an implant body or tooth.”8 It is important to recognize that axial loading is purely theoretical, and, by nature, occlusal forces in the human dentition are nonaxial. Therefore, all implant‐borne restorations are subjected to nonaxial loading. A contemporary highly successful prosthodontic treatment that is based on nonaxial loading is the tilted implant concept, popularly known as the All‐on‐4® concept, which is a testimony to the lack of detrimental effects of nonaxial loading. Additional examples include successful usage of zygoma and pterygoid implants for maxillary rehabilitation (Figure 14.7). In natural teeth, occlusal load is transmitted to the surrounding bone through tensile loading of the periodontal ligament. With implants, the load is transferred directly from the implant to surrounding bone through an ankylotic connection. Previous animal studies have shown no adverse effects on bone surrounding implants, due to nonaxial loading.20,21 At the same time, it is important to recognize that, beyond a certain threshold, nonaxial loading may create strain through the implant–abutment connection and relative motion between components, and eventually result in prosthetic component flexure, fatigue, and eventual component failure. This may not be true in the presence of cross‐arch splinting, as done in complete‐arch fixed implant‐supported prostheses.
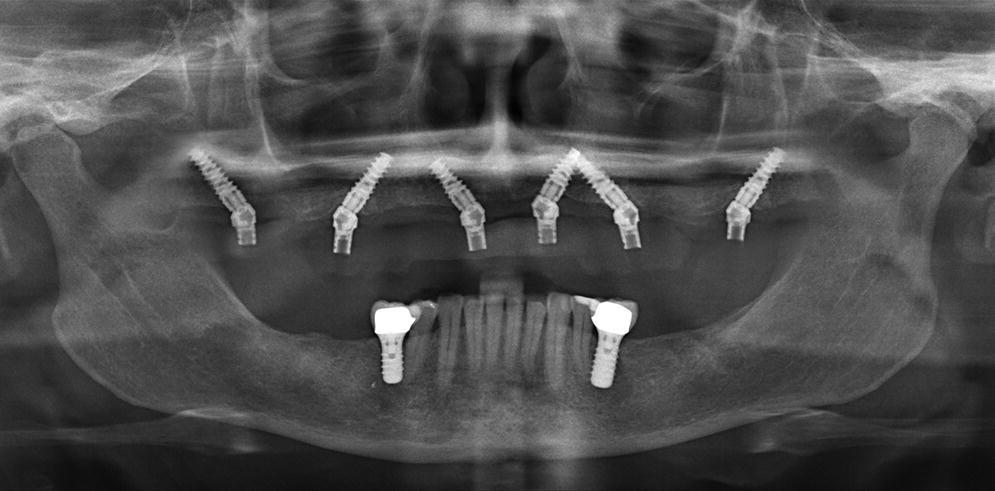
Figure 14.7 Panoramic radiograph showing all six maxillary implants being placed at a 30–45° tilt to overcome anatomic restrictions and allow a successful immediate load of the implants.
Nature of implant–abutment connection
The nature of the implant–abutment connection can either be external (usually external hex) or internal (numerous designs and shapes). Within the scope of occlusion, it is important to note that load transfer in external hex connections is centripetal in nature, while load transfer in internal connections is usually centrifugal in nature (specifically termed “hoop stress”).22 Hoop stress is the stress in a cylindrical object, acting circumferentially in a plane perpendicular to the longitudinal axis.22 Due to the higher clamping force (preload) required to secure the abutment in external hex connections, they are susceptible to abutment screw loosening, especially in single‐crown restorations. A recent systematic review on clinical outcomes of implant abutments used exclusively in the anterior region (where forces are lower) showed that screw loosening was exclusively seen in external‐hex implants and was entirely absent in internal‐connection implants.23 However, a recent study by Chae et al., that compared post‐loading complications on 2651 implants, concluded that implants with internal connection were more prone to technical complications, such as loosening or fracture of the abutment or screw, and the external connection was more vulnerable to biological complications, such as increased pocket depth, thread exposure, and soft tissue complications.24 Whether the results of this large sample study are attributed to the specific implant systems studied should be verified by future clinical trials.
Internal‐connection implants are known to offer good anti‐rotational resistance, decreased dependence on clamping force, and good resistance to nonaxial loading. However, as these implants are hollow to accommodate the internal connection, they are susceptible to hoop stress, which requires the relatively thin walls of the implant to carry the load (Figure 14.8). Fortunately, the reported prevalence of implant fracture is very low (1%) at the present time.25 However, fatigue failure related to the implant material itself is an impending threat in the long term. One solution to mitigate this problem and still use an internal‐connection implant, is to use an implant design that offers an internal connection for the abutment, whilst the restoration is supported by the implant pillar itself (e.g. Straumann Tissue Level Implant). Thus, the internal‐connection implant is now loaded through internally directed compressive forces rather than externally directed hoop stresses (Figure 14.9). This concept is similar to the ferrule effect concept used in restoring endodontically treated teeth.
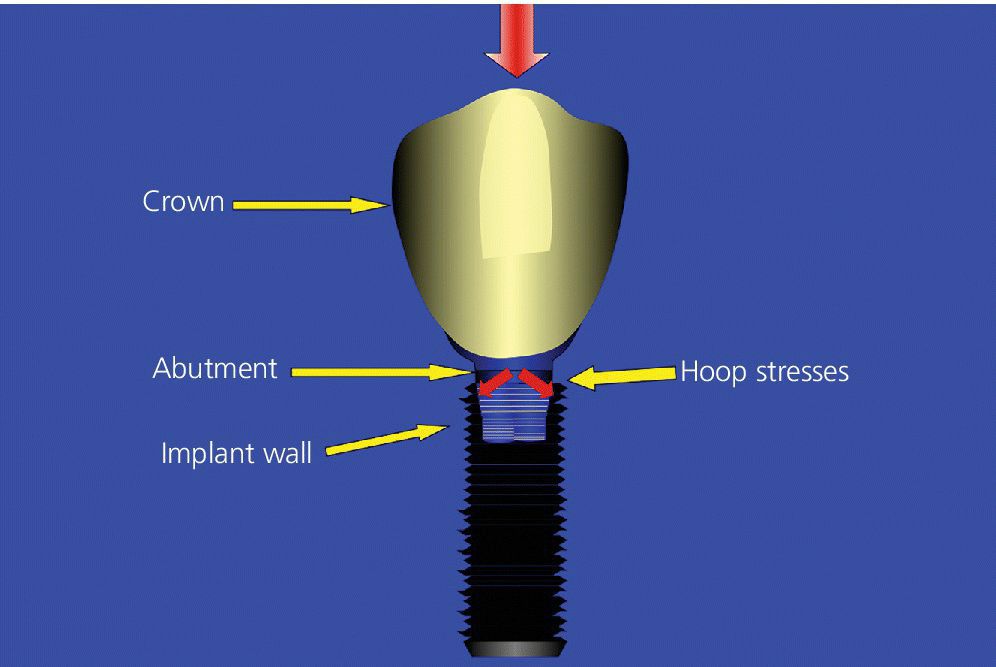
Figure 14.8 Illustration showing the susceptibility of an internal‐connection implant to hoop stress, which requires the relatively thin walls of the implant to carry the load due to external‐directed (centrifugal) forces.
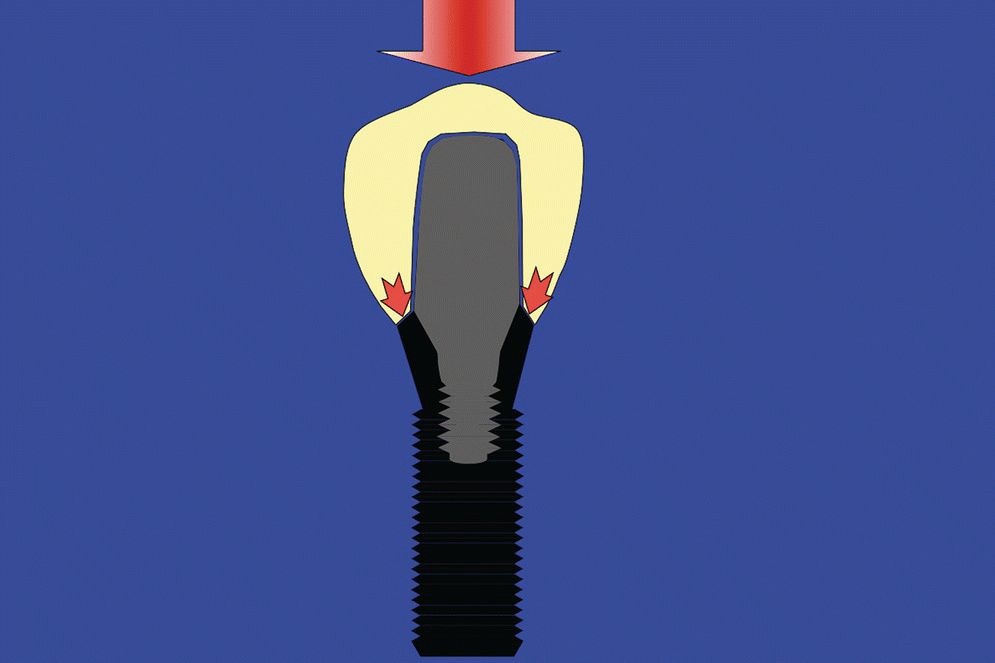
Figure 14.9 Illustration showing the advantage provided to an internal‐connection implant when the restoration is supported by the implant pillar itself. In this situation, the implant is loaded by internally directed compressive (centripetal) forces.
Passive fit
Passive fit has been defined as “the adaptation of one component to another in a manner that does not impart strain.”8 Achieving passively fitting prostheses is desirable in implant prosthodontics. Popular misconceptions about passive fit have stated that damage to the osseointegrated interface is much more likely to occur from a poorly adapted prosthesis than by the transient forces of occlusion.3 Additionally, a popular thought has been that nonpassive adaptation creates permanent, unremitting strain between two or more implants. However, the scientific evidence (largely from animal studies) has shown no adverse effects on bone surrounding implants, due to misfit of prosthetic components.26–31 One clinical study by Jemt et al.27 on 14 patients also concluded that the marginal bone loss around implants was within normal limits when passive fit was absent. Therefore, it can be concluded that nonpassive prosthesis adaptation does not appear to be a major risk factor to the health of the osseointegrated interface.32
It is important for clinicians to acknowledge that some degree of passive fit may be important for the success and survival of prosthetic components, including porcelain fracture.32 It is also important to recognize that some degree of misfit may be present amongst all prosthetic components. The threshold for misfit tolerance before failure of prosthetic components is yet to be defined. Use of computer‐aided design and computer‐aided manufacturing (CAD/CAM) technology and laser welding technology has certainly improved the passive fit of components compared to the conventional casting and soldering techniques. Currently available tools for the clinician and laboratory technician to verify passive fit of prosthetic components over the implants include: 1) plain visual examination; 2) use of a disclosing medium to aid in visual examination (Figures 14.10 and 14.11); 3) tactile feedback from palpation; 4) tactile feedback from a one‐screw test; 5) tactile feedback from a dental plastic explorer; 6) tactile feedback from screw‐clamping force; and 7) radiographs. It is obvious that none of these methods appear scientifically sound nor accurately measurable and reproducible. Moreover, passive fit between an abutment and implant is more difficult to verify in implants with internal connection using the above techniques, and this does not provide confidence of passive fit to the clinician. Finally, it is important to recognize that passive fit in cement‐retained restorations can result in less strain between implants, compared to screw‐retained restorations.33,34 This is perhaps due to the cement behaving as the “slop factor” or as a gasket and ameliorating the adverse effects of increased preload of the abutment screw or occlusal screw.
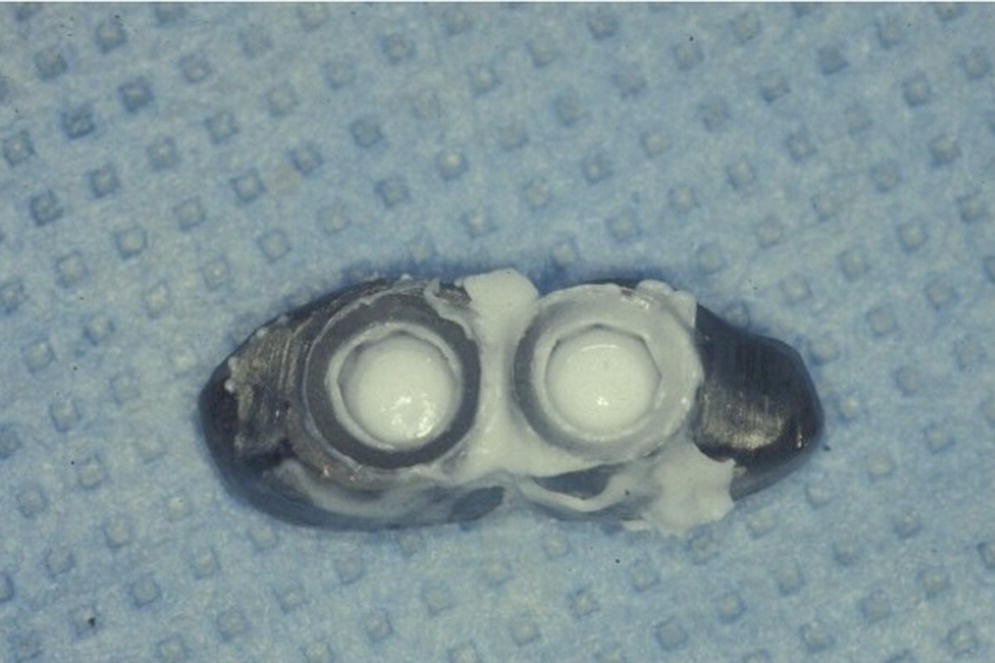
Figure 14.10 Image showing initial attempt at obtaining a complete and passive seating of a splinted implant‐supported restoration using a silicone disclosing medium.
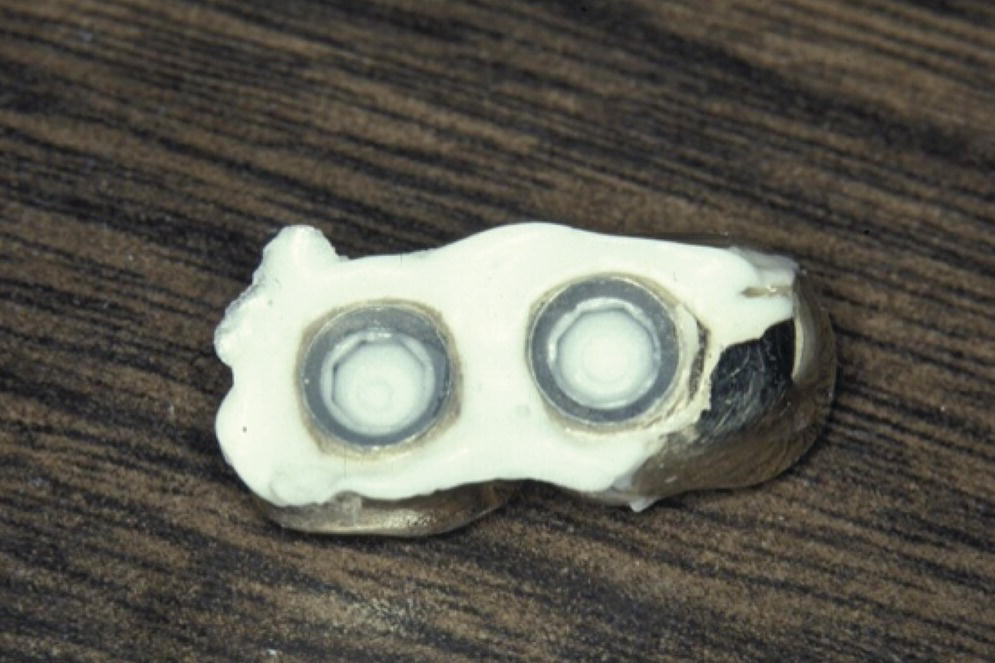
Figure 14.11 Image of the same restoration as Figure 14.10, after adjustments of internal aspect, and proximal contacts show complete seating as revealed by the silicone disclosing medium.
Splinting
The concept of splinting implants to decrease the force magnification around osseointegrated implants is debatable. Scientific evidence is lacking on the beneficial effects of splinting for the osseointegrated interface itself. A recent 10‐year follow‐up randomized controlled trial (RCT) study on 44 patients by Vigolo et al. reported that there was no difference in crestal bone loss with splinted or nonsplinted designs.35 As discussed in the topic of occlusal overload, once osseointegration has been achieved, occlusal overload may only play a role in component failure rather than osseointegration failure. The advantages of splinting two or three adjacent implants is primarily a clinical decision, which provides the advantage of minimizing the number of adjustable proximal contacts at the time of insertion. However, the disadvantages to this approach include potential for compromised patient’s oral hygiene performance, as well as the need for remake of the entire prosthesis should the veneering ceramic fracture. Splinting multiple restorations is an obvious choice when a distal or mesial cantilever is necessary. Splinting across the arch (or commonly called cross‐arch splinting), as done in complete‐arch fixed implant‐supported prostheses, provides the opportunity for simplicity of the prosthetic design and may avoid remaking the prosthesis if there is a future failure of one of the implants. However, cross‐arch splinting does compromise the patient’s oral hygiene performance as well as repair of the prosthesis. Therefore, screw‐retained prosthesis is the obvious choice in such situations.
Splinting of implants to natural teeth should be avoided because of risk of intrusion of the natural tooth or teeth, and risk of secondary caries and periodontal disease resulting in failure of the entire prosthesis. Finally, the opportunity to obtain an adequately fitting prosthesis on the implant abutments and with closed margins on the natural teeth is challenging. In a recent systematic review on splinting natural teeth to implants for fixed prosthesis, intrusion of the abutment teeth occurred in about 5% of cases (all were nonrigid connectors), and about 10% of natural teeth were lost after 10 years. Loss of implants or implant fractures was 15.6% after 10 years, resulting in the survival rate of splinted tooth and implant‐supported restorations at 77.8% after 10 years.36 Another systematic review showed a survival rate of 77.7% in the fixed prostheses connected to natural teeth and implants.37 These data are compelling enough to suggest clinicians should avoid splinting implants to natural teeth.
Cantilevers
Cantilevers in completely edentulous patients treated with implant‐supported fixed prostheses have been used in since the evolution of osseointegration.38 They have a long track record of successful usage for complete‐arch fixed implant‐supported prostheses in the edentulous jaw.39 This is perhaps due to the cross‐arch splinting and the load‐distribution mechanism (Figure 14.12). However, the data are largely restricted to the edentulous mandible, while there are minimal data for the edentulous maxilla. Based on the theoretical models of anterior–posterior (AP) spread, in vitro studies, and empiricism, a cantilever length of 1.5 times the AP spread has been adopted for the mandible by clinicians worldwide.40 This model is not applicable for the maxilla, where reduced cantilever length is usually favored due the biomechanical considerations of the moving mandible that occludes against it. The scientific evidence indicates no adverse effects to bone adjacent to implants due to cantilevers, as discussed in the section of occlusal overload.41
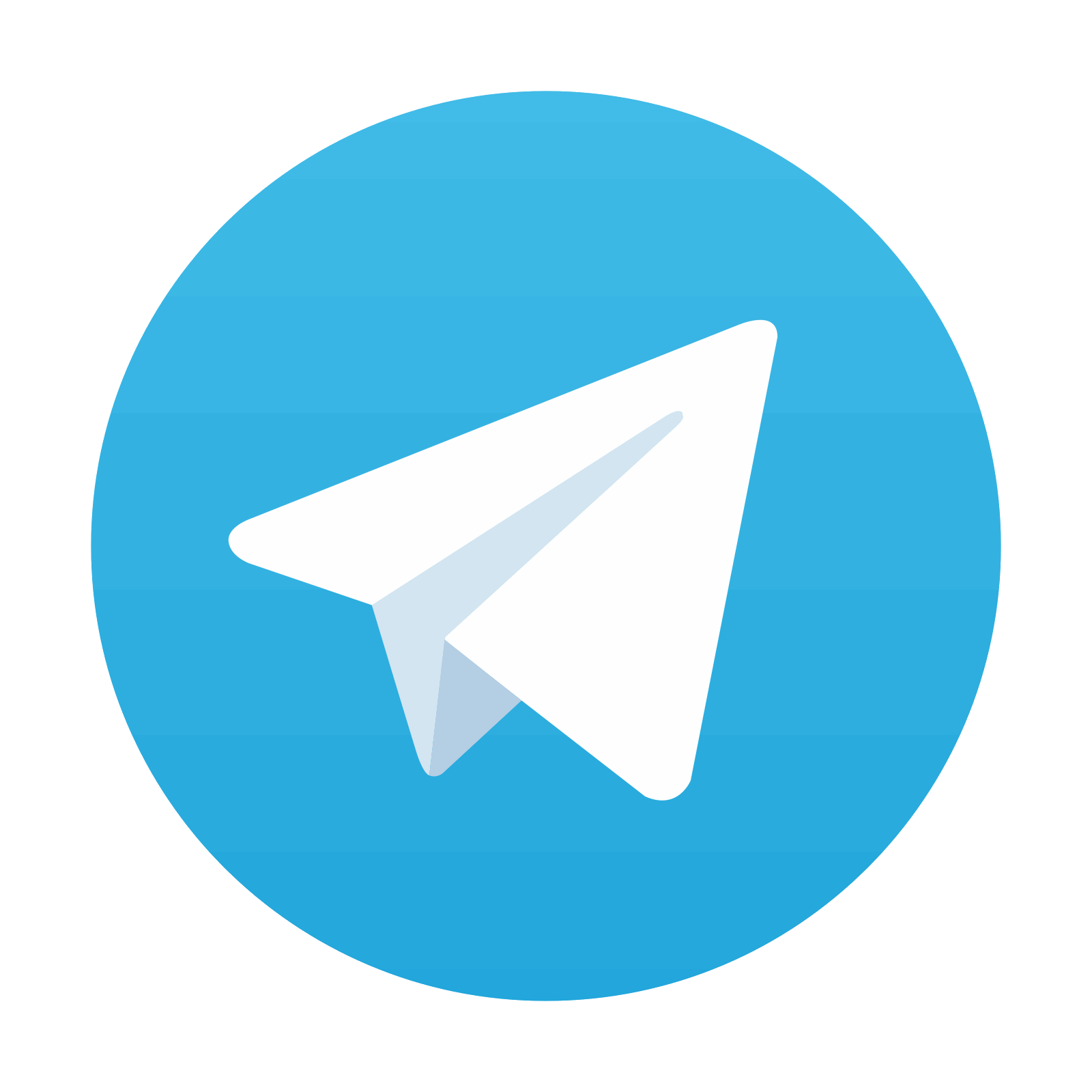
Stay updated, free dental videos. Join our Telegram channel

VIDEdental - Online dental courses
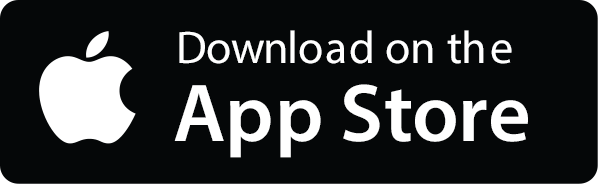
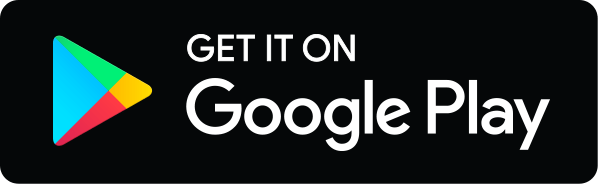