Biomaterials
Introduction
General Considerations
Structure
1. Physical reaction—solidification by drying or cooling with no chemical reaction
2. Chemical reaction—solidification by creating new primary bonds within the composition
(1) Mixing time—time elapsed from onset to completion of mixing
(2) Working time—time elapsed from onset of mixing to onset of initial setting time
(3) Initial setting time—time at which sufficient reaction has occurred to cause the materials to be resistant to further manipulation
(4) Final setting time—time at which the material is practically set, as defined by its resistance to indentation
(1) Mixing interval—length of time of mixing stage
c. All water-based materials lose their gloss at the time of setting
Properties
A Physical properties—events that do not involve changes in composition or primary bonds
a. Weight—gravitational force that attracts a body
b. Mass—resistance of a body to acceleration (or being moved)
a. Linear coefficient of thermal expansion (LCTE, α)
(1) Rate of expansion or contraction of one dimension of a material with temperature change (Figure 13-1)
FIGURE 13-1 Linear coefficient of thermal expansion.
(2) LCTE = [(L2 − L1)/(L1)]/(T2 − T1) where
(3) Values reported as: in/in/°F (inch per inch per degree F), 10−6/°F; cm/cm/°C, (centimeter per centimeter per degree C), 10−6/°C; ppm/°C (parts per million per degree C)
(4) LCTE values—tooth, 9 to 11 ppm/°C; amalgam, 25 ppm/°C; composite, 35 to 45 ppm/°C; inlay wax, 300 ppm/°C
(5) When the thermal expansion of restorative material does not match the tooth structure, percolation of fluids occurs at the margins during cyclic heating and cooling
(1) Insulators transmit heat poorly, for example, dental enamel, dental cements, acrylic polymers, dental porcelain, and ceramic restorations
(2) Conductors transmit heat easily, for example, dental amalgam and cast gold alloys
(3) Teeth with metal restorations may be sensitive to hot and cold foods because of their good thermal conduction
(4) Individuals wearing dentures may not sense normal temperature differences attributable to the thermal insulation of the acrylic denture base
(5) To be an effective insulator, the material must be at least 0.5 mm thick
3. Electrical properties—electrical conductivity
a. Conductors transmit electrons easily, for example, metals
b. Semiconductors transmit electrons sometimes, for example, ceramics and often composites
c. Insulators transmit electrons poorly, for example, ceramics and polymers
a. Contact angle—internal angle of liquid droplet with solid surface
a. Perception—physiologic response to physical stimulus by the eye, which can distinguish three parameters
(1) Dominant wavelength—blue, green, yellow, orange, and red
(1) Munsell Color System (e.g., 5R 6/4)
(a) Hue—color family (R, YR, Y, GY, G, BG, B, PB, P, RP)
(2) Instrumentation techniques—record the spectral reflectance versus wavelength curves (405 to 700 nm)
(1) Metamerism—colors with different spectral energy distributions that look the same under certain lighting conditions but look different with different light sources
(2) Fluorescence—emission of light by a material when a beam of light is shined on it
(3) Opacity—degree of light absorption by a material
(4) Translucency—degree of internal light reflection
(5) Transparency—degree of light transmission through a material
1. Primary chemical bonding types
(1) Hydrogen bonding—where hydrogen is attracted to an electronegative element; found in most water-based liquids
(2) Van der Waals forces—dispersion forces caused by fluctuating dipoles; found in dental composites and acrylics
b. Events related to changes in secondary chemical bonding
(1) Adsorption—uptake “onto” the surface of the solid
(2) Absorption—uptake “into” the solid
(3) Desorption—fluid lost from the solid; for example, water lost from alginate (syneresis)
(4) Solubility—material loss by dissolution of surface
(5) Disintegration—material loss by disruption of solid, usually by absorbed water
a. Chemical corrosion—chemical reaction at surface
b. Electrochemical corrosion—chemical reaction that requires an anode (e.g., dental amalgam), a cathode (e.g., gold crown), an electrolyte (e.g., saliva), and an electrical circuit (e.g., contact) for electron flow (Figure 13-2)
FIGURE 13-2 Electrochemical corrosion.
(1) Galvanic corrosion—dissimilar metals in contact (examples above)
(2) Local galvanic corrosion (structure selective corrosion)—dissimilar phases in the same metal in contact
(3) Crevice corrosion—corrosion in the crack under plaque, between a restoration and the tooth structure, or in the scratch on the surface of a restoration, where the metals may be the same but the electrolytes are different locally
1. Resolution of forces (Figure 13-3)
FIGURE 13-3 Resolution of forces.
a. Uniaxial (one-dimensional) forces—compression, tension, and shear
b. Complex forces—torsion, flexure, and diametral tension or compression
2. Normalization of forces and deformations
(1) Applied force (or material’s resistance to force) per unit area
Lb/in2 (pounds per square inch, psi)
FIGURE 13-4 Stress–strain curve.
a. Plot of stress (vertical) versus strain (horizontal)
(1) Allows convenient comparison of materials
b. Analysis of curves (see Figure 13-4)
(a) Elastic strain—initial response to stress (elastic—when the stress is removed, the strain returns to zero and the material returns to its original length)
(b) Elastic modulus—slope of first part of curve; represents the stiffness of the material, or the resistance to deformation under force
(c) Elastic limit (proportional limit)—stress above which the material no longer behaves totally elastically
(d) Yield strength—stress that is an estimate of the elastic limit at 0.002 permanent strain
(e) Hardness—value on a relative scale that estimates the elastic limit in terms of a material’s resistance to indentation, for example, Knoop hardness scale, Diamond pyramid hardness scale, Brinnell hardness scale, Rockwell hardness scale, Barcol scale, Shore A hardness scale, and Mohs’ hardness scale (Table 13-1); hardness values are used to determine the ability of abrasives to alter the substrates they contact
(f) Resilience—area under the stress–strain curve up to the elastic limit; estimates the total elastic energy that can be absorbed before the onset of plastic deformation
(2) Elastic and plastic behavior
(a) Beyond the stress level of the elastic limit, a combination of both elastic and plastic strain exists
(b) Ultimate strength—highest stress reached before fracture; the ultimate compressive strength is greater than the ultimate shear strength and the ultimate tensile strength
(c) Elongation (percent elongation)—percent change in length up to the point of fracture = strain × 100%
(d) Brittle materials—<10% elongation at fracture
(e) Ductile materials—>10% elongation at fracture
(f) Toughness—area under the stress–strain curve up to the point of fracture (it estimates the total energy absorbed up to fracture)
(a) Strain rate sensitivity—the faster a stress is applied, the more likely a material is to store the energy elastically and not plastically
(b) Creep (i.e., strain relaxation with time in response to a constant stress, such as dental wax deforming because of built-in stresses created during cooling)
(c) Stress relaxation (with time in response to a constant strain)
4. Principles of cutting, polishing, and surface cleaning
(1) Cutting—gross removal of excess material from the surfaces of restorations or teeth
(2) Finishing—fine removal of surface material in an effort to produce finer surface scratches
(3) Polishing—smoothing of surfaces by removal of fine scratches
(4) Debriding—removal of unwanted material attached to surfaces
(5) Air abrasion (air-polishing)—removal or polishing of hard tissue by the kinetic energy from particles sprayed against the surface
(6) Microabrasion—removal of stains by a mixture of an abrasive and hydrochloric acid
b. Surface mechanics for materials (Table 13-2)
TABLE 13-2
Hardness Values for Dental Substrates*
Hardness Value | Number |
CAD/CAM ceramic | 6–7 |
Porcelain | 6–7 |
Composite | 5–7 |
Glass | 5–6 |
Dental enamel | 5–6 |
Dental amalgam | 4–5 |
Dentin | 3–4 |
Hard gold alloys | 3–4 |
Pure gold | 2–3 |
Acrylic | 2–3 |
Cementum | 2–3 |
*Based on Mohs’ scale: diamond = 10; talc = 1 (see Table 13-1).
(1) Cutting—requires materials with the highest possible hardness to produce the cuts
(2) Finishing—requires materials with the highest possible hardness for the best effect, except at the margins of restorations, where the tooth structure may be inadvertently affected
(3) Polishing—requires materials with a Mohs’ hardness that is only 1 to 2 units above that of the substrate
(4) Debriding—requires materials with a Mohs’ hardness that is less than or equal to that of the substrate to prevent scratching
c. Factors affecting cutting, polishing, and surface cleaning
d. Factors affecting air abrasion
(1) Abrasive particle size—27 µm or 50 µm aluminum oxide
(2) Air pressure—higher air pressure cuts faster but may cause discomfort
(1) During cutting, heat will build up and change the mechanical behavior of the substrate from brittle to ductile and encourage smearing
(2) Instruments may transfer debris onto the cut surface from their own surfaces during cutting, polishing, or cleaning operations (this has important implications in cleaning dental implant surfaces)
2. Definitions of local tissue interactions with biomaterials
a. Fibrous tissue capsule formation (tissue encapsulation)
3. Classification of biologic materials—tissue interfaces
4. Clinical analysis of biocompatibility
5. Agencies that oversee materials, devices, and therapeutics
Direct Preventive and Restorative Materials
Dental Amalgam
a. Load-bearing restorations for posterior teeth (class I, class II)
a. Amalgam alloy—powder particles of Ag-Sn-Cu-(Zn) (Note: Minor elements are indicated in parentheses)
b. Amalgam—reaction product of any material with mercury
c. Dental amalgam—reaction product of amalgam alloy (Ag-Sn or Ag-Sn-Cu) with mercury
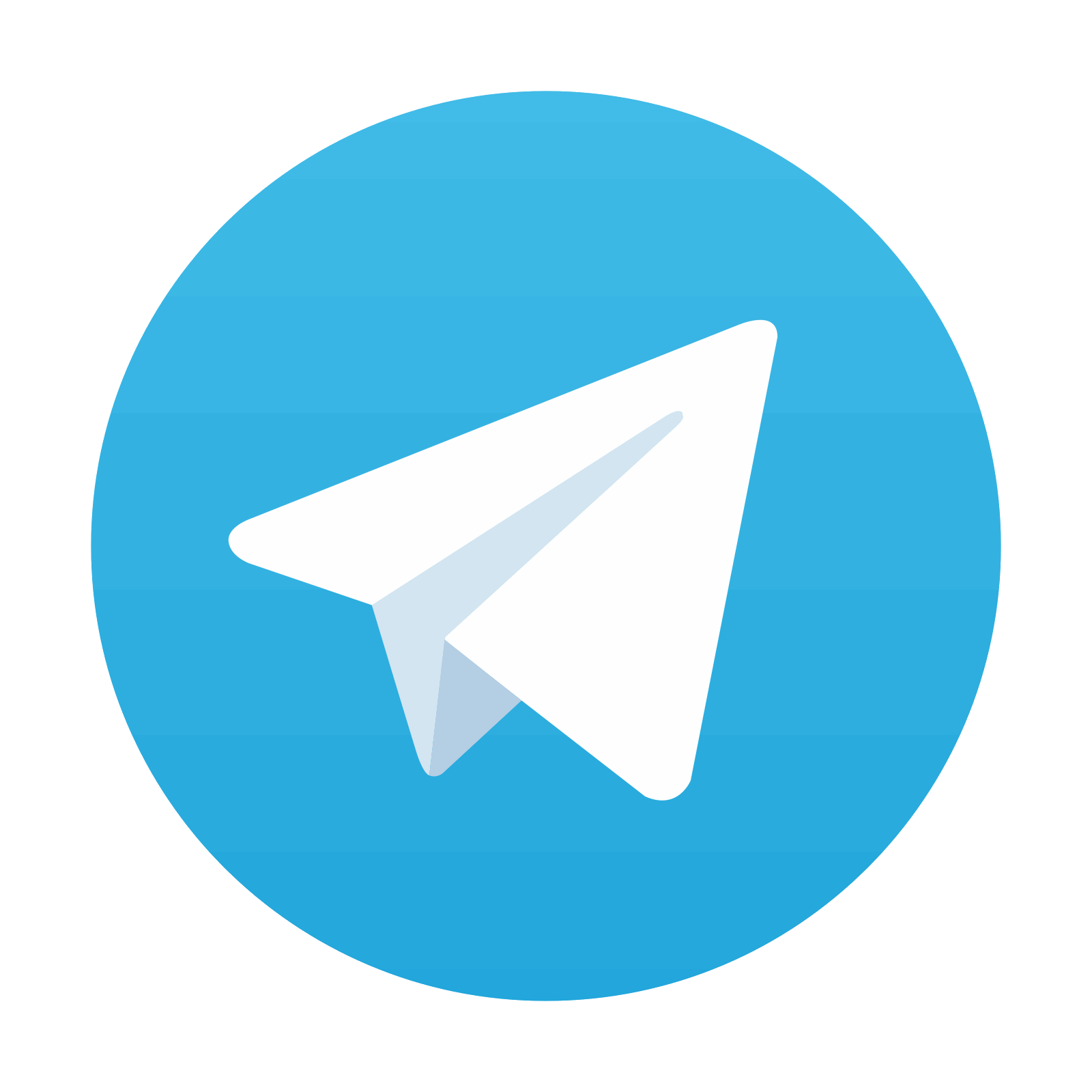
Stay updated, free dental videos. Join our Telegram channel

VIDEdental - Online dental courses
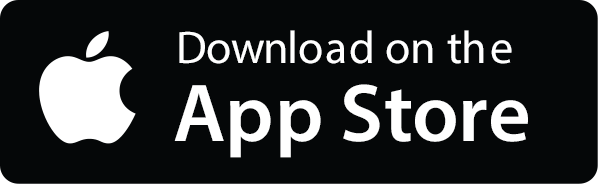
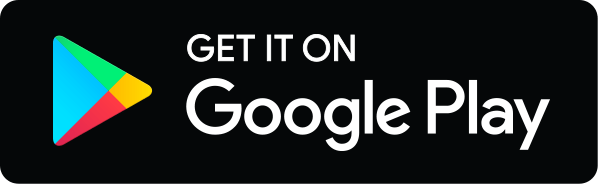