Chapter 12
Review of Concepts for Designing and Fabricating Metal Implant Frameworks for Hybrid Implant Prostheses
INTRODUCTION
Edentulous patients have reported difficulties in managing complete dentures. Marachlioglou et al. (2010) reported that patients had higher expectations regarding their complete dentures than did the dentists who treated them. Dentists reported that dentures would bring fewer benefits to patients than did the patients. Patients with complete dentures have also reported decreased masticatory function in that they avoided certain food types because they were simply unable to chew particular foods (Gjengedal et al. 2011). Lin et al. (2010) reported the results of a clinical study that investigated the relationship between chewing ability and diet among elderly edentulous patients. Approximately 58% of the subjects reported dissatisfaction with their dentures; 51% reported discomfort on chewing. Patient satisfaction or dissatisfaction with their dentures during mastication significantly impacted the diet of these elderly edentulous patients.
Clinical denture issues may be related to loss of alveolar bone post tooth extraction. Dental implants, in addition to providing increased retention and support for prostheses, also have been reported to maintain alveolar bone volume (Jemt 2008). Endosseous implants are thought to maintain bone width and height as long as implants remain anchored in bone with healthy, biologic attachments (Zarb & Schmidt 1996).
HISTORICAL PERSPECTIVE
Two of the objectives regarding definitive implant prosthodontic treatments were the design and fabrication of accurately fitting, strong metal frameworks to splint multiple implants; frameworks also served as the foundation for retaining fixed implant prostheses on a long-term basis. Over the years, multiple, diverse methods have been used for implant framework design and fabrication; different materials have also been used including but not limited to noble/base metal alloys and various ceramic materials (Figures 12.1–12.3).
Figure 12.1 Clinical image of a mandibular fixed hybrid prosthesis approximately 13 years post insertion. Note the extreme wear/abrasion of the artificial teeth; the implant framework on the patient’s right side was exposed secondary to occlusal abrasion.
Figure 12.2 Clinical image of a mandibular implant CADCAM framework for a fixed hybrid implant prosthesis. This framework was made utilizing an L-beam design. Facial and lingual finish lines were machined for finishing the processed acrylic resin.
Figure 12.3 CAD image of a mandibular implant-retained framework designed as an I bar. The facial and lingual finish lines were machined similar to the L-beam design in Figure 12.2.
Figure 12.4 Clinical image of an acrylic resin wrap around the mandibular, fixed implant hybrid prosthesis. The metal framework was completely enveloped within the hybrid prosthesis.
ORIGINAL FRAMEWORK DESIGNS FOR FIXED HYBRID PROSTHESES
Zarb and Jansson (1985a) stated that frameworks (fixed prostheses) could be designed in one of two ways: where metal frameworks comprised the bulk of the prostheses. Artificial teeth and minimal denture bases were the only nonmetallic components or implant fixed prostheses which consisted mostly of acrylic resin denture bases (wrap around design) and artificial teeth, with minimally sized metal frameworks (Figure 12.4). Implant treatment was based on basic prosthodontic principles that included preliminary and definitive impressions, jaw relation records, wax try-in, metal framework try-in (with and without the artificial teeth), and insertion of definitive prostheses. Frameworks were fabricated according to the following criteria: bulk for strength, adequate access for oral hygiene procedures, minimal display of metal on the facial and occlusal surfaces, and strategic thinning of implant frameworks to allow for retention of acrylic resin denture teeth and denture bases. In removable partial denture (RPD) design, it was noted that the retentive portions of RPD frameworks should allow for 1.5 mm thickness of resin. Thickness was also necessary to minimize the potential fracture of the acrylic resin base material surrounding the metal frameworks (Carr et al. 2005). These principles have been extrapolated to fixed implant framework design. It is interesting to note that in an early implant textbook, no mention was made relative to the lengths of the cantilevered segments (Zarb & Jansson 1985a).
Numerous authors have reported on prosthetic maintenance issues with fixed implant prostheses. Zarb and Jansson (1985a) noted that implant frameworks were vulnerable to fracture, especially at the junctions between distal abutments and cantilevered segments. Zarb and Schmitt (1990) reported clinical problems that included abutment screw fracture, gold alloy retaining screw fracture, and framework fractures (12/13 occurred in the cantilevered portions of the frameworks). Relative to framework fracture, Zarb and Schmitt suggested design changes that included cantilevered segments not exceeding 20 mm, increased cross-sectional surface areas, and using casting alloys with higher yield and tensile strengths compared to the alloys used in original osseointegrated prostheses. They also stated that prosthodontic treatment included a series of clinical steps that were mostly empirical and that treatment invariably was accompanied by varying degrees of problems (Figures 12.5 and 12.6).
Oral/facial symmetries and lip contours may be significantly influenced by appropriate/inappropriate maxillary tooth positions, vertical dimension, and/or the need for flanges of varying thicknesses for lip support (Graser et al. 1989; Henry 1998). Upper lip perioral activity may be far more revealing of maxillary gingival tissues than the corresponding activity of the lower lip.
Figure 12.5 Laboratory image of a fractured fixed, implant-retained mandibular prosthesis. This prosthesis fractured through the distal cylinder on the patient’s right side. The fracture also included the occlusal portion of the implant. The exact cause of this failure was unknown although the vertical height of the framework appeared to be approximately 3+ mm.
Figure 12.6 Laboratory image of a fixed, implant-retained mandibular prosthesis where a relatively long distal cantilevered prosthetic segment separated from the metal framework. Note that the implants were placed in a relatively straight line, with minimal A/P spread. Note also the limited vertical thickness of the acrylic resin denture base; this indicated that there was minimal interarch clearance.
Aesthetic demands tend to be more dramatic with maxillary prostheses than mandibular prostheses (Zarb & Schmitt 1990). Per Zarb and Schmitt, unlike mandibular implant prostheses where hygienic-type designs have proven to be functionally and aesthetically acceptable, maxillary implant prostheses demand different sized and shaped labial/buccal flanges that may or may not compensate for optimal aesthetics (including lip support), phonetics, and masticatory function (food impaction between intaglio surfaces and edentulous areas). If flanges are fabricated for upper lip support and phonetics, they may not be readily reduced for access for adequate peri-implant oral hygiene procedures (Figure 12.7). Maxillary prostheses impact speech significantly more than do mandibular prostheses. Patients have also identified speech as a major factor in perceived satisfaction of their prostheses (Jemt et al. 1992; Naert et al. 1992; Marcus et al. 1996; Heydecke et al. 2004; Springer et al. 2011).
Figure 12.7 Laboratory palatal image of a maxillary fixed, implant-retained prosthesis. Note the junction between the prosthesis and the replica soft tissues in the anterior segment. This type of design is thought to facilitate phonetics.
Maxillary functional issues are different from those encountered in edentulous mandibles. Maxillary complete dentures tend to predictably restore original soft tissue contours, tooth positions, and arch forms. Maxillary complete dentures, relative to tongue movements, are generally negligible; speech is not usually impaired. Functional demands for maxillary hybrid implant prostheses are complicated in that phonetics may be affected by hybrid designs and contours, and prosthetic gingival tissues are often required due to the resorptive patterns of edentulous maxillae. Resorptive patterns in the maxillae are dissimilar to mandibular resorption patterns: the maxillae resorb superiorly, posteriorly, and medially; mandibles resorb inferiorly, anteriorly, and laterally (Atwood and Coy 1971; Tallgren 2003). Differences in maxillary and mandibular resorption patterns often lead to unfavorable implant and prosthetic relationships between opposing jaws.
Frameworks for the original fixed, hybrid prostheses were waxed with gold alloy cylinders, cast with silver palladium alloys, and screwed into place with small retaining screws (Zarb & Schmitt 1990). Fixed hybrid prostheses splinted implants together via a strong, rigid metallic unit that fulfilled the objectives of strength, support, nontissue impingement, and noninterference in order to obtain the desired cosmetic results (Zarb & Jansson 1985b). It is interesting to note that in this specific chapter (Chapter 15 Prosthodontic Complications), there was no mention of optimal framework design (Zarb & Jansson 1985c; Goll 1991; Hulterstrom & Nilsson 1991; Bergendal & Palmqvist 1995).
Glantz (1985) in an early textbook stated that fixed implant prostheses were almost invariably extended distal to the most distal implants in order to create optimal functional balance between mandibular and maxillary prostheses. He stated that at the time (1985), there was no precise equation for describing functional deformation patterns of fixed prostheses with cantilevered pontics. He offered a complex equation where deformation of implant-retained, fixed prostheses was inversely related to the module of elasticity and width and height (H) of frameworks and positively correlated with the amount of force and length (L) of frameworks. Therefore, for a given alloy with a known modulus of elasticity, taller (height) and thicker (width) frameworks resisted deformation better than thinner or more narrow frameworks. Therefore, the greater the force generated onto a given framework, with increased framework lengths (cantilever), frameworks would be more likely to undergo deformation when compared to lesser forces and frameworks with decreased cantilever lengths (CL).
PASSIVELY FITTING IMPLANT FRAMEWORKS
Traditionally, implant frameworks were fabricated by using the lost wax technique and casting noble alloys. It has been well established that casting errors may be corrected using various soldering techniques (Moon et al. 1978; Sutherland & Hallam 1990; Jemt 1995). It has been consistent from early reports regarding implant frameworks that passive, accurate fits should be obtained between implant frameworks and implant restorative components (Zarb & Jansson 1985b). It has also been well established that implant frameworks cannot be made to fit passively (Hjalmarsson et al. 2010). Zarb and Jansson identified this in an early textbook by stating that if a clinical passive fit was not obtained, frameworks should be sectioned, an intraoral index made, and then the segments should be soldered (Zarb & Jansson 1985c). Zervas et al. (1999) reported in a laboratory study that soldering did not improve the casting misfit of 3-unit fixed partial dentures (FPDs). Rubenstein and Lowry (2006) reported in another laboratory study that assessed the accuracy of segmental indexing/soldering for full-arch frameworks, using two types of resin, that there were no significant differences noted between alloy/index combinations, except for angular changes around the y-axis.
Laser welding relative to implant frameworks has also been studied. In a study undertaken to describe the effect of laser-welding conditions on material properties of welded frameworks, Uysal et al. (2005) reported that, within the constraints of their finite element analysis, mechanical failure of welded joints should not be expected under simulated intraoral conditions. Silva et al. (2008) reported that implant frameworks may show a more precise adaptation between frameworks and implant restorative platforms when segments were sectioned and laser welded. Hjalmarsson et al. (2010) measured and compared the precision of fit of laser-welded (Cresco) and computer numeric controlled (CNC)-milled metal frameworks for implant-supported fixed complete prostheses. Overall, the maximum three-dimensional (3D) range of center point distortion was 279 µm. None of the frameworks presented a perfect, completely passive fit to any master cast. However, CNC frameworks had statistical significantly less vertical distortions as compared to the Cresco groups. Other reports have resulted in similar findings (Al-Fadda et al. 2007; Eliasson et al. 2008).
Computer-aided design and computer-aided manufacturing (CADCAM) frameworks have been found to fit more accurately when compared to frameworks cast with gold alloys (Takahashi & Gunne 2003; Drago et al. 2009). Implant framework fit and its effect on associated peri-implant bone levels have also been researched. Some authors have concluded that a perfect passive framework is impossible to achieve and, arguably, unnecessary (Hermann et al. 2001; Sahin & Cehreli 2001; King et al. 2002). Although research regarding framework misfit as a cause of peri-implant bone loss is difficult to prove, others have described the value of excellent framework fit for optimal screw mechanics (Binon 1996; McGlumphy et al. 1998).
PROSTHETIC COMPLICATIONS ASSOCIATED WITH FIXED IMPLANT FRAMEWORKS
Prosthetic complications have been defined as treatments, adjustments, or repairs of implant prostheses that became necessary secondary to unexpected events (Taylor et al. 2000; Taylor & Agar 2002). Zarb and Schmitt (1996) identified three types of prosthetic complications: structural, cosmetic, and functional. Zarb and Schmitt (1990) followed 46 patients treated with 274 implants (49 frameworks) for 4–9 years and reported a high incidence of prosthodontic complications associated with fixed implant prostheses: 9 abutment screw fractures (3.3%), 53 gold alloy screw fractures (19.3%), and 13 framework fractures (26.5%). It is important to note that Zarb and Schmitt’s report included patients treated with early prosthetic protocols that included cast alloy frameworks and minimal understanding regarding screw mechanics, torque, preload, and anterior/posterior (A/P) spread. Contrast the aforementioned results with more recent reports. In a 5-year clinical study, Hjalmarsson et al. (2011) reported on the clinical outcomes associated with screw-retained fixed implant prostheses made with laser welding versus frameworks made with milled commercially pure (cp) titanium. They noted significantly more complications in the laser-welded framework group compared to the milled framework group (Hjalmarsson et al. 2011). Ortorp and Jemt (2012) reported the results of a 10-year clinical study and noted that the frequency of prosthetic complications was low, with similar clinical and radiographic results for CADCAM milled and cast gold alloy frameworks. One prosthesis was lost in each group due to loss of implants; one prosthesis fractured in the CADCAM milled group. They noted more maintenance appointments were needed for maxillary prostheses.
PHYSICAL PROPERTIES OF METALS USED IN FIXED IMPLANT FRAMEWORKS
Cast Noble Alloys
Noble metals have been defined on the basis of their chemical and physical properties; noble alloys resist oxidation and corrosion by acids. There are four noble metals used in dental alloys: gold, palladium, silver, and platinum. These metals give noble metal alloys their inert intraoral properties. Alloys that contain more than 6% palladium are usually white/silver colored (O’Brien 2008).
There has been increased usage of palladium/silver alloys in implant prosthodontics. These alloys provide mechanical properties that are similar to type III gold alloys, but at reduced cost. Increased amounts of silver increase ductility and lower hardness; silver also decreases tarnish resistance. Alloys with high palladium contents generally contain limited amounts of other noble metals.
Physical properties such as yield strength, Vickers hardness, and ductility (% elongation) are some of the properties clinicians and dental laboratory technicians consider when deciding which alloy should be used for dental frameworks (O’Brien 2008). Reproducible procedures that result in consistent, accurate, strong castings with high yield strengths are critical for long-term successful metal frameworks. Stress resistance of alloys has an impact on the minimum dimensions in critical areas such as connector areas and cantilevers. Elastic modulus is also important because it determines the flexibility of metal frameworks. Flexibility is inversely proportionate to the elastic modulus – an alloy with a high elastic modulus will flex less under load than an alloy with a low elastic modulus. Casting accuracy is also important in order to fabricate clinically acceptable frameworks.
Palladium/silver alloys usually contain about 50–60% palladium; most of the balance is generally silver. They generally exhibit satisfactory tarnish and corrosion resistance. The elastic modulus for this group of alloys is the most favorable of all the noble metal alloys and results in the least flexible castings (O’Brien 2008). One disadvantage with this group of alloys does not factor into frameworks for implant hybrid prostheses – the tendency to change to a green color with porcelain applications.
Cast Base Metal Alloys
Nonprecious or base metal alloys are composed of non-noble metals, except for beryllium, a precious but non-noble metal. Most base metal alloys are based on combinations of nickel and chromium, although cobalt/chromium and iron-based alloys are also used. Corrosion resistance for base metal alloys depends on other chemical properties. After casting, a thin chromium oxide layer provides an impervious film that passivates the alloy surface. The layer is so thin that it does not dull the alloy surface. These alloys differ significantly from noble alloys as they possess significant hardness, high yield strengths, and high elastic moduli. Elongation is equivalent to the gold alloys but is countered by the high yield strength. Base metal alloys are significantly less expensive than noble alloys, but this may be negated by higher labor costs associated with finishing and polishing procedures. Allergies associated with nickel and nickel-containing alloys have been documented (Moffa 1982). Inhaling dust from grinding nickel- and beryllium-containing alloys should be avoided.
Milled Titanium Frameworks
Titanium and titanium alloys are well suited for use in clinical dentistry because they have excellent corrosion resistance, low specific gravity, and excellent biocompatibility; are inexpensive; and possess mechanical properties that are similar to cast gold alloys. Titanium and its alloys are difficult to cast due to their high melting points, low density, and reactivity with elements in casting investments (Pieralini et al. 2010).
Milled Zirconium Frameworks
Zirconia has been available for use in restorative dentistry as a dental ceramic replacement for metal frameworks in fixed and implant prosthodontics. The type of zirconia used in dentistry is yttria tetragonal zirconia polycrystal (YTZP). YTZP is a monophase ceramic material formed by directly sintering crystals together without any type of intervening matrix to form a dense, polycrystalline structure. Yttria is added to zirconia to stabilize and maintain the material’s physical properties at lower temperatures than would otherwise occur without yttria.
The flexural strength of zirconia oxide materials has been reported to be 900–1100 MPa (White et al. 2005). There are three main types of zirconia used in clinical dentistry: fully sintered or hot isostatic pressing (HIP), partially sintered zirconia, and non-sintered or green-state zirconia. The latter two types are softer than HIP zirconia and more cost efficient to mill. After milling, zirconia frameworks are sintered />
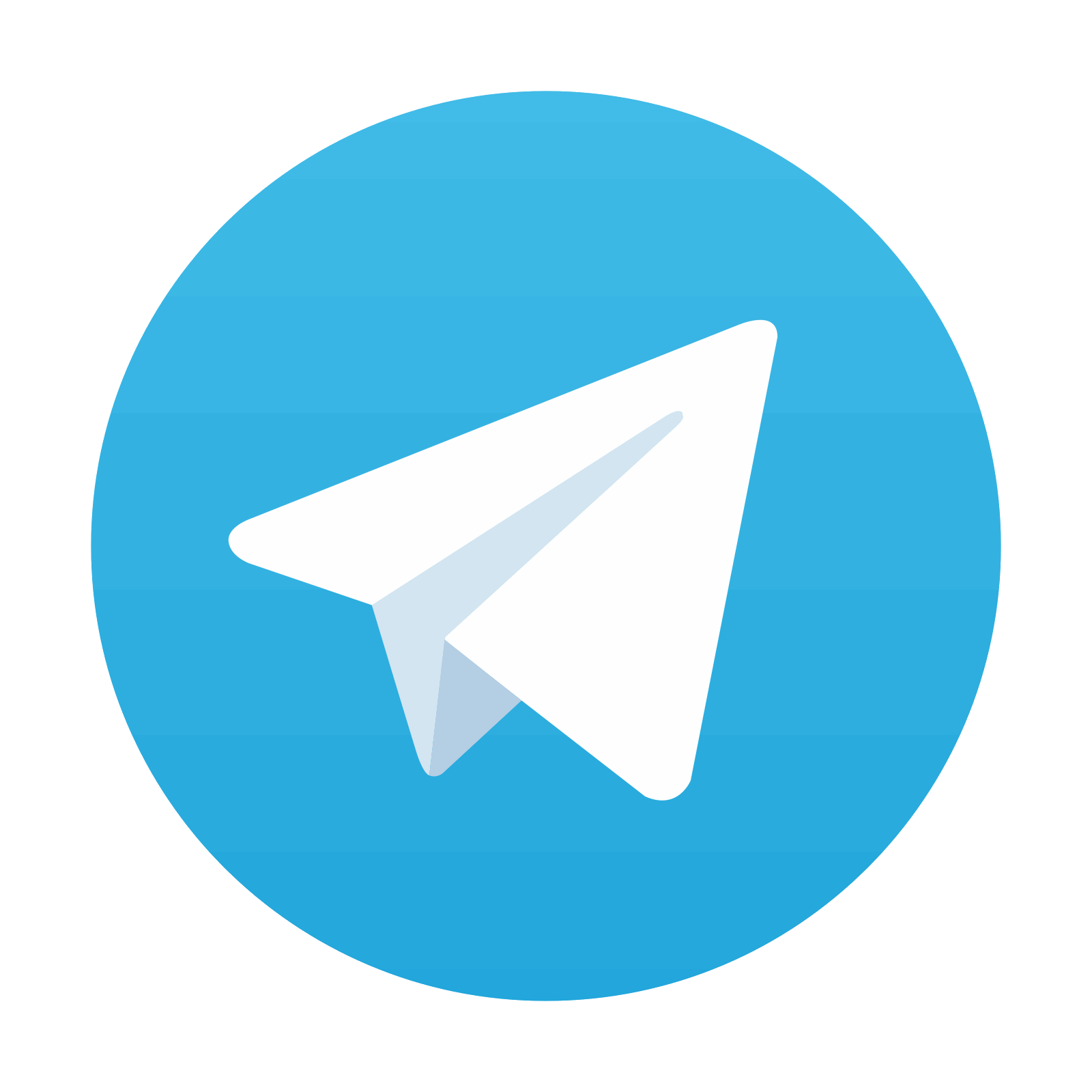
Stay updated, free dental videos. Join our Telegram channel

VIDEdental - Online dental courses
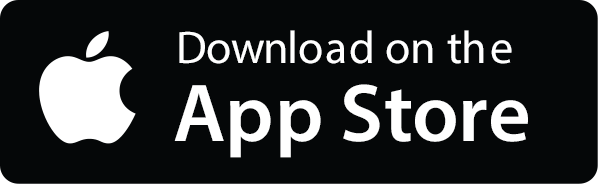
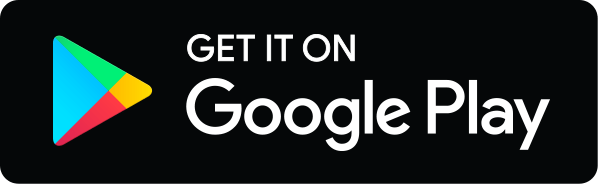