Reconstructive Oral and Maxillofacial Surgery
• Posterior Mandibular Augmentation
• Pectoralis Major Myocutaneous Flap
Posterior Mandibular Augmentation
Assessment
Caucasian male, 69 years old, with a history of hypercholesterolemia, prostatic hypertrophy, GERD, chronic neck pain, and chronic rhinitis/sinusitis presents for an evaluation for dental implant placement after extraction of periodontally involved teeth. The physical exam reveals vertical insufficiency of the right mandibular and bilateral maxillary posterior alveolar ridges, in addition to a horizontal alveolar deficiency of the right mandibular posterior alveolar ridge (Figure 12-1).
Figure 12-1 A, Initial panoramic radiograph showing generalized periodontal disease and insufficient height of bilateral maxillary and right mandibular posterior alveolar ridges for implant placement. B, Right posterior mandible showing severe periodontal disease and buccal horizontal defects at the posterior area.
Treatment
Alveolar deficiencies of the posterior mandible present unique surgical challenges. Defects must be accurately assessed for the horizontal and vertical deficiencies of bone and the amount of keratinized tissue available to support the final prosthesis. A host of reconstructive techniques and materials must be considered, and the most appropriate method selected to maximize the individual patient’s outcome (Figure 12-2). The success of endosteal implant restorations and prostheses has made augmentation of the posterior mandible a necessary skill for oral and maxillofacial surgeons. The use of titanium mesh, autogenous bone, allogeneic/xenogeneic bone, and inlay bone grafting is discussed later.
Figure 12-2 A, Panoramic radiograph showing titanium mesh placement for posterior ridge augmentation. A mixture of autogenous bone from a right mandibular torus, hydroxyapatite, and platelet-rich plasma was used under the mesh. B, Titanium mesh after 6 months of healing, with pseudomembrane intact. C, Implant placement at teeth #28, #29, and #30 after titanium mesh removal. D, Final panoramic radiograph showing right mandibular implants with restoration. The patient also underwent bilateral maxillary sinus lifts, right maxillary ridge split osteotomy, and implant placement at teeth #3, #5, #11, #12, #14, #18, and #20. E, Postoperative photograph showing restored posterior mandibular implants in function 8 years after placement.
Vertical Defects
In 2009, Esposito and colleagues performed a Cochrane systematic review of randomized controlled trials (RCTs) for horizontal and vertical ridge augmentation. Of the 13 trials that met the inclusion criteria, 10, enrolling 218 patients, addressed vertical ridge augmentation. Analysis of the trials found that vertical augmentation resulted in a high complication rate (20% to 60%) and graft failure rates of 10% to 15%. Interestingly, two split-mouth trials compared alloplastic grafting (anorganic bovine bone and Regenaform, respectively) with autogenous bone grafting (iliac crest and particulate bone) and showed no statistical difference in outcomes. Although both studies had small sample sizes (10 and 5 patients), the reduction in operative time, cost, and patient discomfort certainly justify further investigation. The review also included a meta-analysis of two RCTs examining mandibular ridge augmentation (iliac crest inlay graft and anorganic bovine inlay) versus short implant placement without augmentation. The meta-analysis showed an increased implant failure rate (borderline significance; p = 0.06), and a statistically significant increase in the complication rate in the augmented group. The additional time, cost (e.g., general anesthesia, hospitalization), and patient discomfort are also important factors. However, the long-term outcomes of short implants in the posterior mandible have not been adequately evaluated to date.
Discussion
With the growing popularity of implant restorations, mandibular ridge augmentation has become a necessary skill for oral and maxillofacial surgeons. The posterior mandible can be a particularly difficult area to successfully augment due to the unique anatomy of the area. A thorough understanding of surgical techniques and bone grafting options is vital to maximizing the final functional and esthetic outcomes for the patient (Figure 12-3).
Figure 12-3 A, A 59-year-old Caucasian male with left posterior mandibular vertical deficiency. B, Completion of osteotomy for inlay graft (sandwich osteotomy technique). C, Mobilization of the mandibular alveolar segment. A block of allograft bone was used without fixation for the interpositional graft. D, Postoperative panoramic radiograph with block graft in place, showing adequate height for implant placement in left posterior mandible.
Bell, RB, Blakey, GH, White, RP, et al. Stages reconstruction of the severely atrophic mandible with autogenous bone graft and endosteal implants. J Oral Maxillofac Surg. 2002; 60:1135.
Chiapasco, M, Abati, S, Romeo, E, et al. Clinical outcome of autogenous bone blocks or guided bone regeneration with e-PTFE membranes for the reconstruction of narrow edentulous ridges. Clin Oral Implants Res. 1999; 10(4):278–288.
Clavero, J, Lundgren, S. Ramus or chin grafts for maxillary sinus inlay and local onlay augmentation: comparison of donor site morbidity and complications. Clin Implant Dent Relat Res. 2003; 5(3):154–160.
Cordaro, L, Amade, DS, Cordaro, M. Clinical results of alveolar ridge augmentation with mandibular block bone grafts in partially edentulous patients prior to implant placement. Clin Oral Implants Res. 2002; 13:103.
Corinaldesi, G, Pieri, F, Sapigni, L, et al. Evaluation of survival and success rates of dental implants placed at the time of or after alveolar ridge augmentation with an autogenous mandibular bone graft and titanium mesh: a 3- to 8-year retrospective study. Int J Oral Maxillofac Implants. 2009; 24:1119–1128.
Esposito, M, Grusovin, MG, Felice, P, et al. The efficacy of horizontal and vertical bone augmentation procedures for dental implants: a Cochrane systematic review. Eur J Oral Implantol. 2009; 2(3):167–184.
Felice, P, Pistilli, R, Lizio, G, et al. Inlay versus onlay iliac bone grafting in atrophic posterior mandible: a prospective controlled clinical trial for the comparison of two techniques. Clin Implant Dent Relat Res. 2009; 11(Suppl 1):e69–82.
Felice, P, Marchetti, C, Iezzi, G, et al. Vertical ridge augmentation of the atrophic posterior mandible with interpositional bloc grafts: bone from the iliac crest vs bovine anorganic bone—clinical and histological results up to one year after loading from a randomized-controlled clinical trial. Clin Oral Implants Res. 2009; 20:1386–1393.
Her, S. Titanium mesh as an alternative to a membrane for ridge augmentation. J Oral Maxillofac Surg. 2012; 70:803–810.
Louis, P, Gutta, R, Said-Al Naief, N, et al. Reconstruction of the maxilla and mandible with particulate bone graft and titanium mesh for implant placement. J Oral Maxillofac Surg. 2008; 66:235–245.
Louis, P. Bone grafting the mandible. Oral Maxillofac Surg Clin North Am. 2011; 23:209–227.
Mertens, C, Decker, C, Seeberger, R, et al. Early bone resorption after vertical bone augmentation: a comparison of calvarial and iliac grafts. Clin Oral Implants Res. 2013; 24:820–825.
Nissen, J, Ghelfan, O, Mardinger, O, et al. Efficacy of cancellous block allograft augmentation prior to implant placement in the posterior atrophic mandible. Clin Implant Dent Relat Res. 2011; 13(4):279–285.
Pieri, F, Corinaldesi, G, Fini, M, et al. Alveolar ridge augmentation with titanium mesh and a combination of autogenous bone and anorganic bovine bone: a 2-year prospective study. J Periodontol. 2008; 79:2093–2103.
Proussaefs, P, Lozada, J. The use of intraorally harvested autogenous block grafts for vertical alveolar ridge augmentation: a human study. Int J Periodontics Restorative Dent. 2005; 25:351.
Proussaefs, P, Lozada, J, Kleinman, A, et al. The use of ramus autogenous block grafts for vertical alveolar ridge augmentation and implant placement: a pilot study. Int J Oral Maxillofac Implants. 2002; 17:238.
Roccuzzo, M, Ramieri, G, Spada, MC, et al. Vertical alveolar ridge augmentation by means of a titanium mesh and autogenous bone grafts. Clin Oral Implants Res. 2004; 15:73–81.
Roccuzzo, M, Ramieri, G, Bunino, M, et al. Autogenous bone graft alone or associated with titanium mesh for vertical alveolar ridge augmentation: a controlled clinical trial. Clin Oral Implants Res. 2007; 18:286–294.
Simion, M, Baldoni, M, Zaffe, D. Jawbone enlargement using immediate implant placement associated with a split-crest technique and guided tissue regeneration. Int J Periodontics Restorative Dent. 1992; 12:462–473.
Tolstunov, L, Hicke, B. Horizontal augmentation through the ridge-split procedure: a predictable surgical modality in implant reconstruction. J Oral Implantol. 2013; 39(1):59–68.
Waasdorp, J, Reynolds, MA. Allogeneic bone onlay grafts for alveolar ridge augmentation: a systematic review. Int J Oral Maxillofac Implants. 2010; 25(3):525–531.
Watzinger, F, Luksch, J, Millesi, W, et al. Guided bone regeneration with titanium membranes: a clinical study. Br J Oral Maxillofac Surg. 2000; 38:312–315.
Radial Forearm Free Flap
Examination
General. The patient is a slim, pleasant white man who appears his stated age.
Intraoral. The patient is edentulous and has a prominent ulceration at the left posterior lateral tongue that measures 3 cm in length and 2 cm in width. The red and white lesion is tender and has a necrotic center with firm, everted edges along its periphery (Figure 12-4). The tongue is freely mobile. There appears to be no extension into the floor of the mouth.
Imaging
The work-up of squamous cell carcinoma of the tongue includes CT scanning of the head and neck with intravenous contrast (for improved delineation of soft tissue) and a chest radiograph (see the section Squamous Cell Carcinoma in Chapter 11).
Treatment
Access to the lesion is considered first. In the current patient, the resection can be completed via a transoral approach. For larger oral squamous cell carcinomas of the oral cavity, it is not uncommon to gain access via a lip split mandibulotomy or “pull through” approach (Figure 12-5). Along with resection of the tumor, reconstruction of the defect is planned preoperatively. In general, reconstruction is based on the concept of the reconstructive ladder. The methods of reconstruction are ranked by complexity; most descriptions of the reconstructive ladder start with closure by secondary intention, primary closure, local flaps, regional pedicled flaps, and microvascular free flaps. In the current patient, the surgical defect after resection would result in a significant loss of tissue, because oncologic clearance generally incorporates 1 to 1.5 cm for the margins and, depending on frozen sections, may incorporate another 3 to 5 mm circumferentially.
Figure 12-5 Access surgery for tongue resection. A, Lip split mandibulotomy approach. An osteotomy is completed through the symphysis of the mandible. A subtotal glossectomy was completed with the remaining base of the tongue identified by the attached black stitch. B, Pull-through approach. A subtotal glossectomy was completed by the release of the lingual attachments of the floor of the mouth and the tongue. The remaining tongue is demonstrated on the right side of the image; on the left side is the skin paddle of a radial forearm free flap that is secured to the remaining posterior right base of the tongue.
The current patient was placed under general anesthesia and underwent a tracheostomy to secure his airway. A marking pen was used to delineate the planned resection edges, and a paper template was used to approximate the size and shape of the resection. A left hemiglossectomy was performed, and the margins of the resection were confirmed to be adequate with frozen sections (Figure 12-6, A and B).
Figure 12-6 A, Left tongue defect after left hemiglossectomy. B, Left hemiglossectomy specimen. C, After completion of the supraomohyoid neck dissection. The sternocleidomastoid muscle, internal jugular vein, common facial vein, and external jugular vein are clearly exposed for the microvascular reconstructive phase. D, An 8 × 6-cm skin flap is raised on the distal volar surface of the forearm. Note the radial artery and the cephalic vein travelling toward the antecubital fossae. E, Radial forearm free flap vascular pedicle; also, artery and veins associated with the harvest of a radial forearm free flap with exposure to the antecubital fossae. F, Inset radial forearm fasciocutaneous free flap.
A left selective neck dissection was performed to sample lymph nodes from levels I through III and to expose and preserve vessels for microvascular reconstruction (Figure 12-6, C). These vessels included the internal jugular vein and the internal and external carotid arteries and their associated branches.
Simultaneous harvest of the radial forearm free flap was performed on the nondominant hand (Figure 12-6, D). The template was used to approximate the area of skin needed for the fasciocutaneous flap harvest. Generally, the skin of the entire volar forearm may be harvested, extending from the antecubital fossae to the flexor crease of the wrist. The skin and subcutaneous tissue are thinner in the distal forearm than in the proximal portion of the forearm. Also of note, the area of the distal forearm is thinner in males than in females.
Clinical landmarks that assist in the harvest of a radial forearm free flap include the distal extent of the flap within a flexor crease of the wrist, the antecubital fossae, and the brachioradialis, flexor carpi radialis, and palmaris longus, in addition to the outline of the planned flap. The flap is centered to overlap the region of the vascular pedicle located between the brachioradialis and flexor carpi radialis (Figure 12-6, E).
Once the radial forearm flap had been harvested, it was inset into the intraoral defect (Figure 12-6, F). Because of the vessel geometry, the pedicle was delivered medial to the left mandibular body and placed alongside the great vessels of the neck. Excess skin was removed to achieve the desired bulk, and the flap was secured to the resection wound edge prior to the microvascular vessel anastomosis. The term “ischemia time of the flap” refers to the time from harvesting of the flap to the moment the artery is reestablished. Free flap reconstructions require a period of tolerance to tissue ischemia. Studies have demonstrated that this period of tolerance varies with the tissue type. Most flaps incorporate skin, fascia, muscle, and bone tissue. Skin and fascia are the most resistant to ischemia; they have a tolerance period of 4 to 6 hours before irreversible cellular injury occurs that could jeopardize the viability of the free flap. Muscle is generally the most sensitive to ischemia time; it has a tolerance period of less than 3 hours.
Discussion
Since the original description of the radial forearm fasciocutaneous free flap, more than 30 years ago, this technique has evolved into a versatile and reliable flap for head and neck reconstruction. First described by Taylor in 1976 and publicized in oral cavity reconstruction by Soutar and colleagues in 1983, this flap continues to be a popular soft tissue flap despite the challenges with donor site morbidity. Alternative flaps, such as the cutaneous lateral arm flap, fasciocutaneous ulnar flap, and anterior lateral thigh perforator flap, have limited advantages, which focus on less donor site morbidity.
There are many applications for the radial forearm free flap in head and neck reconstruction. As demonstrated in this case presentation, tongue reconstruction with the radial forearm free flap is a common indication. Use of this type of flap has been described for reconstructing the ablative defects of the soft palate and mandible, restoring soft tissue deficiencies, and repairing tracheoesophageal fistulas. Dual skin flaps have been used to reconstruct through-and-through defects in the cheek and lip, and with two-stage procedures, prefabricated grafts can be prepared for reconstruction of complex structures such as the nose (Figure 12-7).
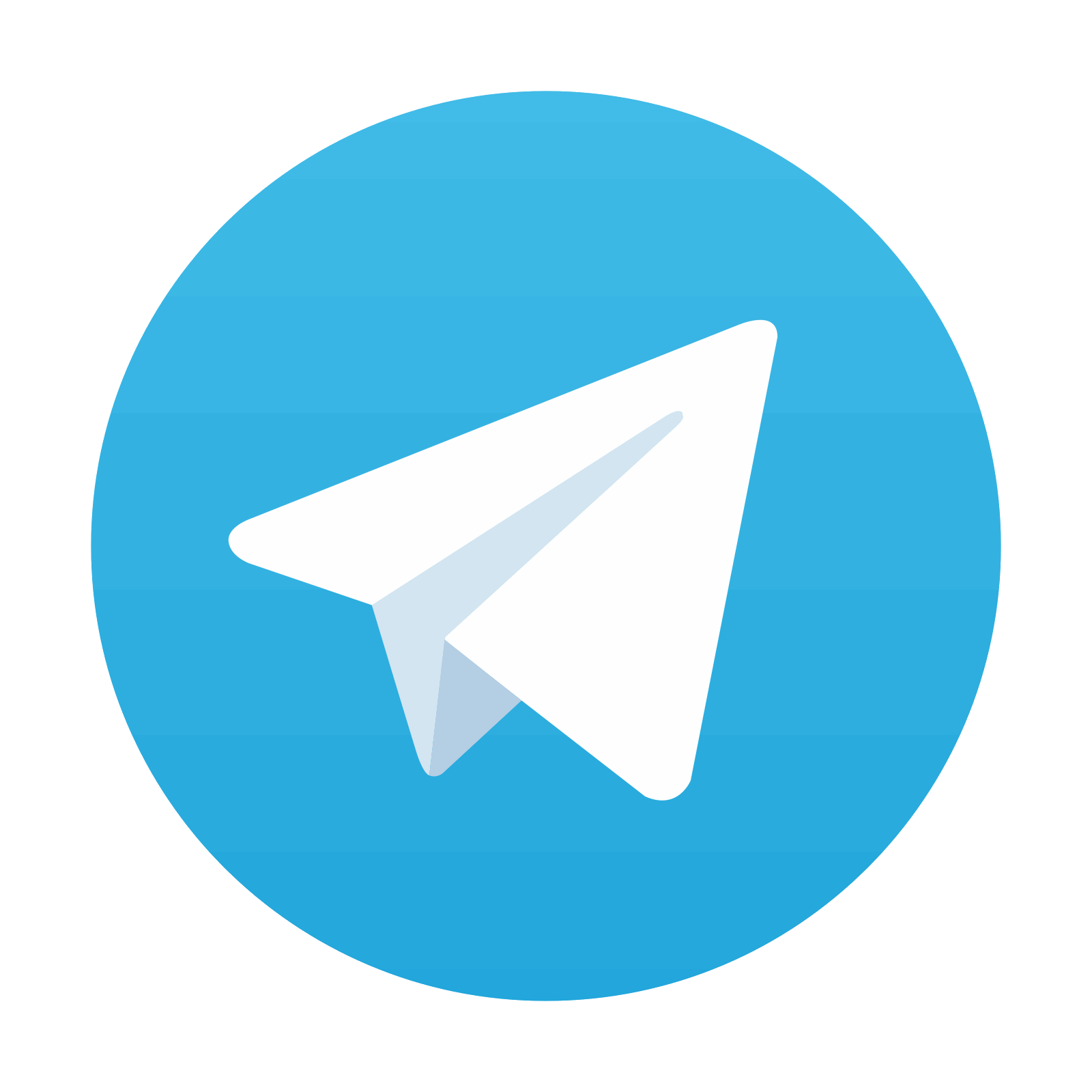
Stay updated, free dental videos. Join our Telegram channel

VIDEdental - Online dental courses
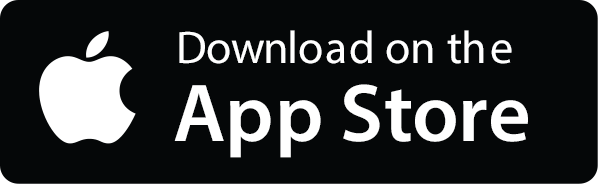
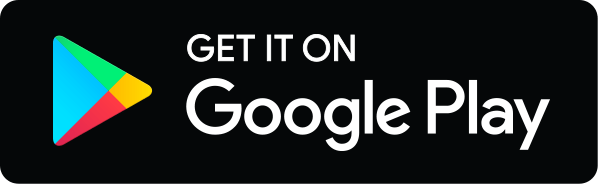