12
How Orthodontic Appliances Move Teeth
Introduction
When an orthodontic appliance delivers a force of sufficient magnitude to a tooth, the tooth will move, if its root is surrounded by healthy periodontal tissues and alveolar bone. An orthodontic force compresses the periodontal ligament and alveolar bone adjacent to the advancing root, stimulating resorption of alveolar bone by osteoclasts. The root surface opposite to the compression side stretches the periodontal ligament and, in turn, stimulates the activity of osteoblasts to form new alveolar bone in the wake of a moving tooth. A healthy periodontal ligament is essential for tooth movement. When the root of a tooth is directly connected to the alveolar bone with no intervening periodontal ligament, a condition called ankylosis, the tooth will not respond to orthodontic forces. For a similar reason, it is not possible to move an osseous integrated dental implant with an orthodontic appliance.
An orthodontic force produces different cellular responses on the compression and tension sides of the root during tooth movement (Foster 1982). On the compression side, lower orthodontic forces that do not occlude the blood vessels of the periodontal ligament stimulate osteoclasts to resorb the alveolar bone facing the compressed periodontal ligament. On the tension side, blood vessels dilate, osteoid tissue (new bone) is created by osteoblasts on the surface of the alveolar bone facing the stretched periodontal ligament, and the fibers in the periodontal ligament connected to the alveolar bone reorganize. High orthodontic forces on the compression side of the root that occlude blood vessels and create cell-free areas (hyaline-like) in the periodontal ligament prevent osteoclastic resorption of the alveolar bone surface. Without bone resorption, the tooth cannot move in the direction of the force. Under these conditions, increased blood flow to internal spaces in the alveolar bone on the pressure side eventually stimulates osteoclasts from within the bone to resorb the alveolar bone in a process called undermining resorption. Excessively high forces can kill cells in the periodontal ligament, cause pain to the patient, result in root resorption, and will delay movement of a tooth.
Schwarz (1932) demonstrated in a histologic study the rotation of the premolars in a dog around a point approximately in the middle of the root after the teeth were moved in a buccal direction by a finger spring. He named the center of rotation the tilt axis. On the side of the root opposite to the finger spring between the tilt axis and the alveolar crest, the periodontal ligament was compressed and the surface of the alveolar bone facing the periodontal ligament was undergoing resorption. On the opposite side of the root between the tilt axis and the crest of the alveolar bone, the periodontal ligament was stretched and new alveolar bone was developed on the surface of the bone facing the stretched periodontal ligament.
This pattern was reversed on the sides of the root between the axis of rotation and apex. Schwarz placed lingual arches with finger springs in the mandibular and maxillary arches of a young dog. He moved a lower right fourth premolar buccally with a 0.5-mm (0.020-inch) diameter spring that delivered 3 to 5 grams of force for 5 weeks, adjusting the spring twice, at the beginning and after 2.5 weeks. The tooth moved 1 mm. Drawings were made of Schwarz’s histologic photomicrographs. Two histologic drawings of the tooth after 5 weeks of movement are shown in Figure 12.1. A low-magnification view of the tooth, its axis of rotation, and surrounding tissues is shown in Figure 12.1A. A high-magnification view of the compression side of the tooth near the alveolar crest is shown in Figure 12.1B. This view shows the old bone with its haversian systems, a line of resorption at the surface of the alveolar bone facing the compressed periodontal ligament, and some individual osteoclasts near the resorption line. Schwarz moved an upper right second premolar buccally with a 0.35-mm (0.014-inch) diameter spring that delivered 20 grams of force for 2.5 weeks. Two histological drawings of this tooth are shown in Figure 12.2. A low-magnification view of the tooth, its axis of rotation, and the surrounding tissues is seen in Figure 12.2A. A high-resolution view of the tension side of the tooth near the cervix of the crown is shown in Figure 12.2B. This view shows new bone developed adjacent to the stretched periodontal ligament, a line of demarcation between the new, and old bone with its haversian systems.
On the basis of his histologic evidence, Schwarz concluded that the 3- to 5-gram and 20-gram finger spring forces resulted in biologically favorable orthodontic tooth movement. Schwarz also moved a lower right second premolar buccally with a 0.5-mm (0.20-inch) diameter spring that delivered 67 grams of force for 5 weeks, adjusting the spring twice, at the beginning and after 2.5 weeks. At 2.5 weeks after starting the spring force, the tooth stopped moving; after the second activation, the tooth moved again. Two histologic drawings of this tooth are shown in Figure 12.3. A low-magnification view of the tooth, its axis of rotation, and the surrounding tissues is seen in Figure 12.3A. A high-magnification view of the compression side of the tooth near the alveolar crest is shown in Figure 12.3B. In the high-magnification slide, the periodontal ligament is compressed, and a line of resorption is seen on the alveolar bone facing the periodontal ligament. According to Schwarz the force from the spring had decreased to zero, the resorption line is densely covered by osteoblasts, and the initial resorption has quickly changed to apposition. A resorption cavity reached into the dentin of the root. Schwarz (1932) concluded that a force of 67 grams was fairly strong and caused root resorption.
Buck and Church (1972) moved human maxillary first premolars buccally with 70 ± 7 grams of force using 18-mil (0.45-mm) diameter stainless steel finger springs soldered to a stainless steel palatal arch soldered to bands on the maxillary first molars. At this level of force, they observed osteoclasts within the alveolar bone on the compression side participating in undermining resorption and loss of cells in the compressed periodontal ligament.
From this brief evidence in animal and human studies, we conclude that finger spring forces from 5 to 20 grams produce biologically favorable tooth movement. Finger spring forces of 70 grams and higher will cause biologically unfavorable events in the tooth root, periodontal ligament, and alveolar bone.
Biomechanics
The material in this Chapter is an updated version of a previous publication (Staley 1987). Orthodontic appliances move teeth in three planes of space (Badawi et al. 2009). For this discussion, the three planes of space associated with the dental arches are (1) a parabolic-shaped mesiodistal vertical (MDV) shell that passes through the long axis of each tooth [Dempster, Adams, and Duddles (1963)]; (2) buccolingual planes that pass through the long axis of each tooth at right angles to the MDV shell for each tooth; and (3) a horizontal plane that passes through the midpoints of the buccal and facial surfaces of the clinical crowns at a right angle to the buccolingual and parabolic MDV shell (Fig. 12.4). The MDV shell may have a curvature in the anteroposterior direction (curve of Spee). The horizontal plane may have a curvature from the right side of the arch to the left (curve of Wilson).
Because orthodontic appliances move a tooth in all three planes of space simultaneously, none of the movements of a tooth are expressed wholly in only one of these planes. Andrews (1972, 1989) described the terms angulation and inclination in ideal occlusions. In the parabolic-shaped MDV parabolic shell that extends around the arch, an orthodontic appliance can alter the angulations of the long axes of teeth and move them along the long axis of the arch wire (Fig. 12.4B). Teeth also move vertically up and down in this plane (or shell). In the horizontal plane, an orthodontic appliance moves a tooth buccally, labially, or lingually (Fig. 12.4C). The appliance rotates the crown and root of an abnormally rotated tooth in the horizontal plane. The buccolingual planes bisect the teeth from the facial to lingual, are unique for each tooth, and extend around the parabolic arch (Fig. 12.4D). In the buccolingual planes, orthodontic appliances can change the inclination of the long axis of a tooth. In the edgewise appliance, change in the inclination of the long axis of a tooth is called “torquing,” because, the edgewise wire is twisted (torqued) by a clinician so that when the arch wire is engaged in the rectangular slot of a bracket or tube, it will rotate the tooth around the center of the arch wire in the rectangular slot of the edgewise bracket (Fig. 12.5C).
Isaac Newton (1952), an English mathematician and physicist, proposed a theory containing three basic assumptions that describe the motions of bodies or particles that travel great distances in comparison to their mass. He was interested in describing the motions of the planets and their satellites in the solar system. He considered the earth a particle, because for its mass the earth travels a great distance around the sun. Newton’s three “laws” of motion accurately describe the motions of bodies on earth. Scientists have analyzed the motions of rigid and deformable bodies composed of many particles. We assume that a tooth is a rigid body having a center of mass that corresponds to the particle that Newton described. A tooth is embedded in alveolar bone; therefore, its center of mass for our purposes is the center of mass of the embedded part of the root, not the center of mass for the entire tooth. The center of mass of the embedded part of the root is called the center of resistance. The roots of most single-rooted teeth are similar to the geometric form called a semiellipsoid. The center of mass of a semiellipsoid is located three-eighths the distance from its base to its apex on its long axis (Ginsberg and Genin 1984).
The upper central incisors have roots that resemble a cone. The center of mass of a cone is located one-fourth the distance from its base to its apex on its long axis. The center of resistance of a tooth was located in the illustrations of this chapter halfway between the cervix of the tooth and the apex of its root(s) on the long axis of the root, as an approximation.
Newton described two different forces that act on a body: a surface traction and a body force. An orthodontic appliance delivers a surface traction to a tooth. Gravity is an example of a body force, because it pulls on all the particles of a body.
Newton’s First Law
Newton’s first law of motion states that a particle that is free of all forces will remain at rest or in a state of moving forward uniformly unless an impressed force compels it to change. This is known as the law of inertia. Teeth are not bodies free of all forces. Occlusal, eruptive, growth, muscular, and habitual forces impress on the teeth of patients with a malocclusion. In patients with normal occlusion, intraoral forces exist in an equilibrium that stabilizes the teeth during growth, adulthood, and aging. Orthodontic appliances overcome the forces of inertia and move the teeth of patients with malocclusion into new, more normal positions to establish a new and hopefully more stable equilibrium of intraoral forces. Appliances must apply sufficient force to a tooth to overcome its innate resistance to movement; its inertia that comes from its mass; the gingival, periodontal, and bony tissues surrounding its root; and the forces that impact its crown. The tooth-moving force must also overcome the force of friction that is associated with the edgewise fixed appliance (Andreasen and Quevedo 1970). In addition, the force must overcome any impressed forces, such as those arising from occlusion, if these forces oppose the force delivered by the orthodontic appliance.
Newton’s Second Law
Newton’s second law states that the acceleration of a particle is proportional to the impressed force and inversely proportional to the mass of the particle, and the particle accelerates in the same direction as the force. Clinicians have two major concerns: (1) that the force delivered to the tooth is of sufficient magnitude to move the tooth in a biologically sound manner and (2) that the force will move the tooth in the desired direction. The brackets, tubes, bands, and cementing materials of a fixed appliance become part of the tooth. The strength of the bonds between the fixed appliance and the teeth must be great enough to resist the forces delivered by the appliance when it moves the teeth and moderate enough to not damage the enamel surface when the appliance is removed.
Newton summarized his second law of motion in the equation: F = MA, where F is force, M is mass, and A is acceleration. Engineers define force in terms of dynes and newtons. One dyne is the force required to accelerate a mass of 1 gram 1 centimeter per second squared. One Newton is the force required to accelerate a mass of 1 kilogram 1 meter per second squared. Clinicians usually measure force in units of mass or weight such as grams or ounces. One Newton is equivalent to 102.8 grams or 3.6 ounces.
Keys to Understanding the Delivery of Orthodontic Forces
To explain how teeth move in removable and fixed edgewise appliances, the following assumptions are made: (1) that a tooth is a rigid body partly embedded in an adaptable, flexible, biologically active matrix; (2) that a tooth, when subjected to a force of sufficient and appropriate magnitude, moves in the responsive matrix; (3) that the motions of a tooth in the appliance are described by rotations; and (4) that the forces delivered to the tooth by the appliance conform to the principles of a lever.
Crabb and Wilson (1972) retracted maxillary canines in 20 human patients with finger springs on removable appliances that delivered 30 grams (6 patients), 40 grams (7 patients), and 50 grams (7 patients. They found that the canines moved/>
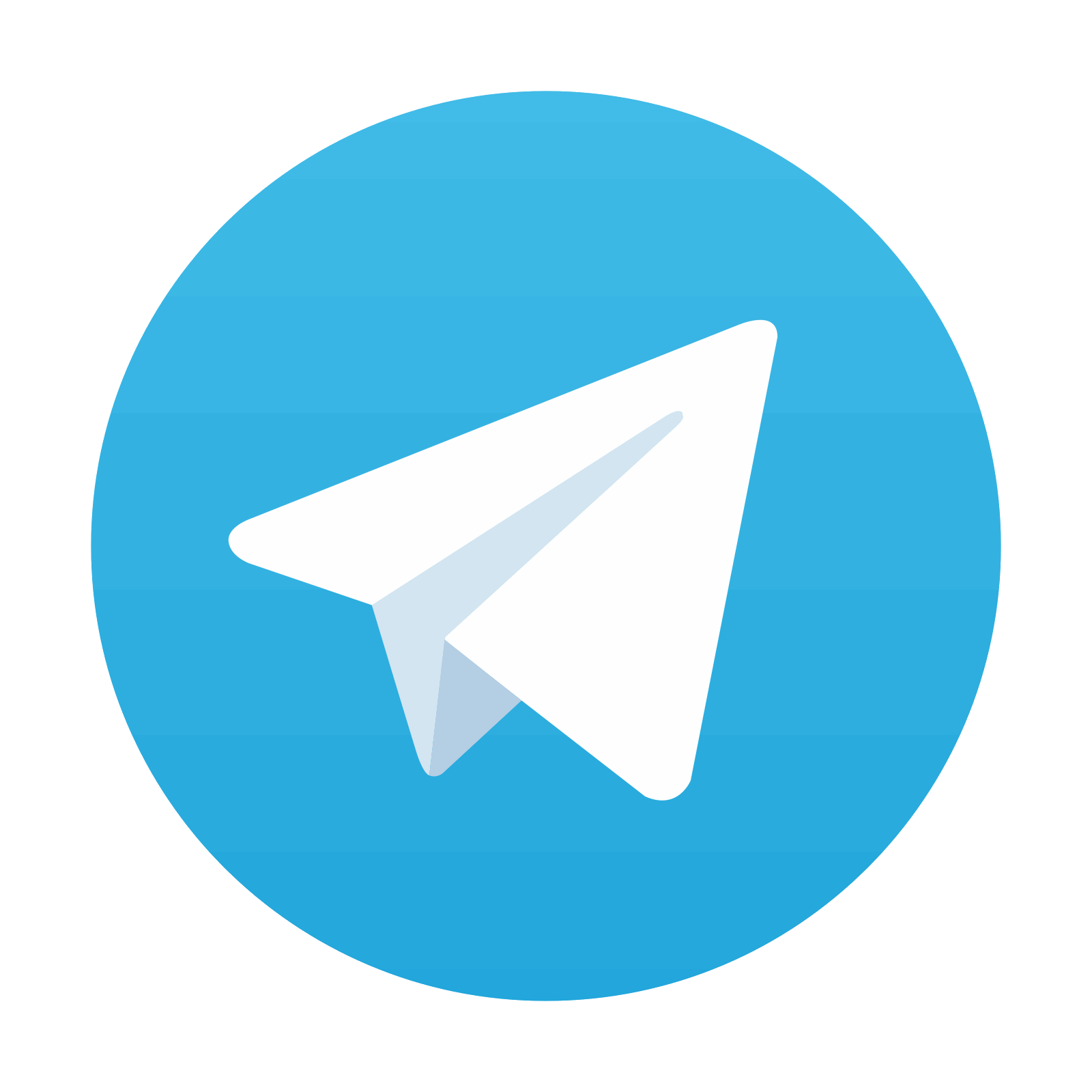
Stay updated, free dental videos. Join our Telegram channel

VIDEdental - Online dental courses
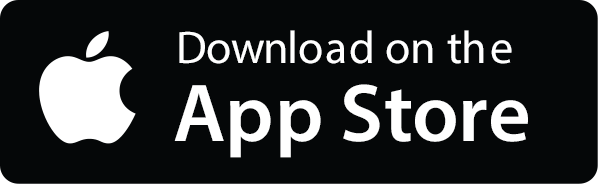
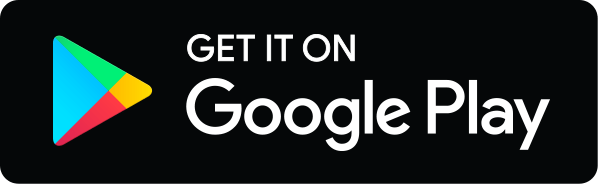