Casting Alloys, Wrought Alloys, and Solders
Objectives
After reading this chapter, the student should be able to:
< ?mpslid E1?>< ?mpslid S2?>
2. Describe the general composition and properties of high-noble, noble, and base-metal casting alloys.
< ?mpslid E2?>< ?mpslid S3?>
< ?mpslid E3?>< ?mpslid S4?>
4. Explain clinical problems associated with different types of ceramic-bonding alloys.
< ?mpslid E4?>< ?mpslid S5?>
5. Explain how solders are used in dentistry, and list properties important to their successful use.
< ?mpslid E5?>< ?mpslid S6?>
< ?mpslid E6?>< ?mpslid S7?>
7. Explain what properties of alloys are most important to alloy biocompatibility.
< ?mpslid E7?>
Key Terms
Alloys
Base metals
Brazing
Casting
Ceramic-bonding alloys
Cobalt
Copper
Crown
Density
Dental casting alloys
Gold
Grain boundaries
Grains
Greening
Hardness
High-noble alloys
Indium
Inlay
Iron
Melting range
Modulus
Nickel
Noble alloys
Noble metals
Onlay
Palladium
Platinum
Pontics
Predominately base-metal alloys
Silver
Solders
Strength
Titanium
Wrought alloys
Yield strength
Zinc
Alloys have been used in dentistry for thousands of years to replace natural tooth structure. The earliest restorations used pure gold because it was easy to purify, melt, and manipulate. Gold in foil form probably was used first as a dental restorative material several thousand years ago. Gold foil is unique because it can weld to itself under hand pressure into a solid mass at mouth temperature (Figure 11-1). This restorative strategy is still used but is not common in clinical practice. Other pure metals such as platinum also are used in dentistry, but pure metals, including gold and platinum, generally lack appropriate properties to be used for large dental restorations. For this reason, metals and nonmetals are mixed together to form alloys.
Alloys have physical and chemical properties more appropriate to dental applications than pure metals. Some alloys are formed into restorations by casting. A wax model of the restoration is made, and an alloy is melted and cast into the shape of the wax (Chapters 10 and 12). Thus, these alloys are referred to as dental casting alloys, and the restorations made from these alloys are castings. For anterior or posterior restorations, ceramic can be bonded to the alloy to provide an esthetic result. Alloys used for this purpose are called ceramic-bonding alloys or ceramic-fused-to-metal alloys. Other alloys are first cast but are then shaped by mechanical force (e.g.; machining) into their final forms. These alloys are referred to as wrought alloys and include wires, files, and dental implants. Still other alloys are used to join alloys together. These types of alloys are solders, and they must be melted without distorting the alloys they join. Finally, alloy composites are formed by sintering (Chapter 14) and are used as metal substructures for ceramic–alloy restorations.
Alloys play critical roles in dentistry (Figure 11-2). Some alloys are used for inlays, onlays, crowns, or fixed and removable partial dentures. Orthodontic wires have special bending properties that permit controlled application of forces to teeth and bone. Endodontic files used to shape root canals are made from stainless steel or titanium-based alloys. Endosseous dental implants are made from titanium-based alloys (Chapter 15). This chapter introduces several principles about dental alloys and solders and surveys the composition, physical properties, and biologic properties of alloys that are most important to clinical success in dentistry. Casting and soldering are discussed in Chapter 12.
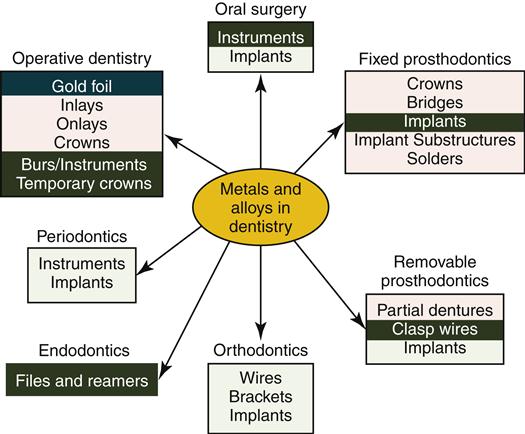
The manipulation of alloys is generally not the purview of the dental auxiliary. However, a basic knowledge of these important materials is critical to appropriate clinical care, communication with patients, coordination with laboratory personnel, and appropriate financial considerations.
Fundamental Concepts About Metals and Alloys
Noble Metals, Base Metals, and Nonmetals
Metals are elements in the periodic table of the elements that tend to react by donating electrons to other elements. Nearly two thirds of the periodic table is composed of metals (Figure 11-3). Most elements used in dental alloys or solders are metals, but nonmetals also play important roles. For example, carbon is sometimes added to alloys in small amounts (<1 wt. %) to strengthen the alloy significantly (e.g., “carbon-steel”).
In dentistry, metals are subdivided into two major groups: noble metals and base metals (see Figure 11-3). Noble metals are defined by their resistance to corrosion even under extreme conditions that occur in the oral cavity. There are seven noble metals in the periodic table, but only three are common in dental casting alloys: gold (periodic table symbol Au), palladium (Pd), and platinum (Pt). Some metallurgists also consider silver (Ag) a noble metal, but because of its tendency to corrode in the oral environment, silver is not considered a noble metal in dentistry. Noble metals are expensive simply because they are rare. For this reason, noble metals are traded as precious metals in commodities markets. However, the term precious should not be used to describe dental metals or alloys as this term indicates only costs on the free market. Furthermore, there are precious metals that are not noble. The term noble is preferred because it describes an important physical property of the metal or alloy.
In dentistry, base metals are metals that are not noble metals (see Figure 11-3). In dental casting alloys, common base metals are titanium (Ti), nickel (Ni), copper (Cu), silver (Ag), cobalt (Co), and zinc (Zn). Base metals often are mistakenly viewed as being “bad” metals. In fact, base metals are required in alloys to ensure the strength, flexibility, and wear resistance necessary for dental restorations. However, in pure form, base metals have a greater tendency to corrode in the oral environment than noble metals. For this reason, pure base metals are almost never used for dental restorations. One exception is titanium, which is used in nearly pure form for endosseous implants (Chapter 15).
Elements in Dental Alloys
It is beyond the scope of this book to describe the 25 or more elements that are used in dental alloys. However, several of the most common elements are described in more detail here. Gold is a noble element that is perhaps the best known of the dental metals (Figures 11-3 and 11-4). Historically, gold played a bigger role than it does today, but it is still a common component of many dental alloys. Gold is used because of its excellent resistance to corrosion, good malleability (ability to be mechanically formed), yellow color, and relatively low melting point (1064° C). Gold may make an alloy appear yellow, but color is not reliable for identifying gold-containing alloys (see later discussion).
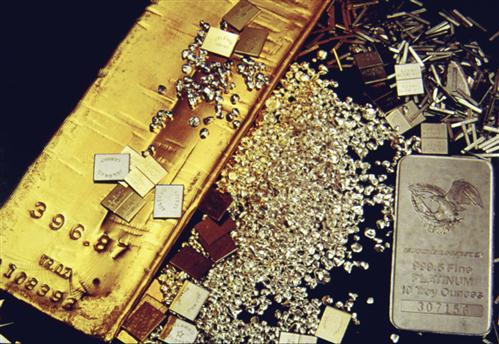
Palladium is a second common noble element component of dental casting alloys (see Figures 11-3 and 11-4). Its corrosion resistance is excellent, but it has a much higher melting point (1554° C) and is much harder than gold. Thus, palladium is not practical to use in pure form but often is mixed with gold-based alloys to increase their hardness or increase their melting temperature. Palladium also whitens the color of gold-based alloys. For example, an alloy may have 90% gold and only 10% palladium (by weight), but its color will be white, not yellow. Platinum is the third common noble element in dental casting alloys (see Figures 11-3 and 11-4). Platinum has a high melting point (1772° C) and is even harder than palladium. However, platinum is used less in dental alloys because it does not mix as freely with gold as palladium does to form alloys, and it is the most expensive of the three metals.
Copper, silver, and zinc are common base metals used in dental casting alloys. Copper is reddish and its addition significantly hardens gold- or palladium-based alloys through a phenomenon called solid-solution hardening. Silver also is used to harden alloys this way. Zinc is a low-melting element (420° C) used to prevent oxidation of the alloy during the casting process; zinc has been used as a hardener for gold-platinum alloys.
Nickel, cobalt, titanium, iron, and indium are other base metals used in dental alloys. These elements may occur in trace amounts to elicit specific properties or may serve as the major element in the alloy. For example, iron is added in trace amounts to enhance the bonding of ceramic to gold-based alloys, or indium may be added to encourage a small grain size. On the other hand, alloys with nickel or cobalt as the most common component are increasingly common because of the low cost of these elements. Alloys of titanium are commonly used as endosseous implants (Chapter 15).
Crystal Structure and Grains of Alloys
Dental alloys have a crystal structure like ice, salt, or sugar. For example, when water freezes, microscopic crystals of ice first form in the water then grow slowly in size until the crystals run into one another and all of the water is frozen. There is a period in the freezing process when ice and liquid water coexist. Alloys behave much the same way. When a molten (liquid) alloy freezes, crystals form and grow as the alloy cools. These crystals, or grains as they are called in metallurgy, can be seen clearly under a microscope (Figure 11-5). Each grain consists of a crystal lattice of metal atoms. The lines between crystals are called grain boundaries. The size of the grains is important; a small grain size is generally more desirable because it ensures uniform properties of the alloy. Elements called grain refiners often are added to gold-based alloys to reduce the grain size (e.g., Ir or Ru in Figure 11-3). The grain structure of many alloys is far more complex than shown in Figure 11-5 and is highly dependent on the composition of the alloy. Alloys that are predominately base metals such as nickel generally have larger grain sizes and grain refiners cannot be used.
The grain structure of an alloy is significantly altered by mechanical forces. For example, if the alloy is rolled into a sheet or drawn into a wire or machined by a cutting instrument, the grains are disrupted. This type of alloy is called a wrought alloy and the grain structure takes on a fibrous appearance (Figure 11-6). Heating an alloy after casting or mechanical work changes its grain structure. These changes may lead to significant changes in properties of the alloys and clinical problems. For example, if the springlike qualities of an orthodontic wire rely on a fibrous grain structure, then overheating the alloy and altering the fibrous structure will cause the spring to be weakened. Thus, operations in dentistry that involve heating, such as the application of ceramic or soldering, must be done with consideration for how the grain structure of the alloy may change.
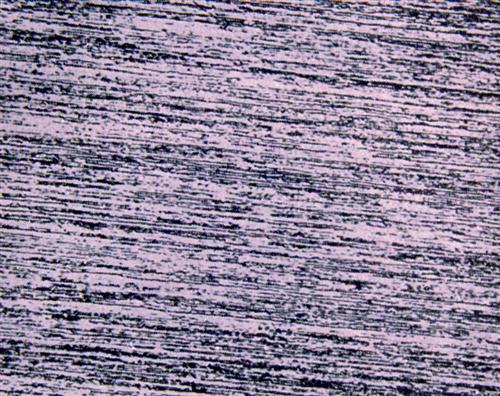
Other important changes in alloy crystal structure are not visible, even under a microscope. In some dental alloys, how the atoms occupy the crystal lattice of the alloy is exceedingly important. For example, for an alloy of gold and copper, the alloy will be considerably stronger if the copper atoms occupy regularly occurring positions in the crystal lattice (called an ordered crystal structure; see Figure 11-5). The copper atoms can be induced to change lattice positions depending on how the alloy is cast (or reheated) and cooled. Thus, for gold-based alloys with appropriate amounts of copper, heating (to within 100° C of the molten form) and cooling actually may be used to improve the properties of the alloy. Changes in the order of the atoms in the crystal lattice are invisible to the eye and even invisible in an electron microscope (they must be detected by x-ray diffraction). Not all alloys exhibit ordered-structure hardening. For example, with base-metal alloys, heating and cooling cycles generally deteriorate the properties of the alloy. Labs use ordered crystal structures to harden or soften some alloys to a clinical advantage.
Important Physical and Mechanical Properties of Alloys
Dental alloys have several properties important to their laboratory and clinical performance. These properties—which include color, melting range, density, modulus strength, and hardness—are discussed in the following paragraphs (Table 11-1).
TABLE 11-1
Clinically Important Properties of Alloys
PROPERTY (units) | DESCRIPTION | CLINICAL IMPORTANCE |
Color | White (silver) to yellow | —Has little significance other than personal preference |
Melting range (°C) | Range of temperatures over which alloy goes from solid to liquid; first melting occurs at the solidus; all liquid occurs at the liquidus | —Soldering (alloy melting range must be higher than melting range of solder) —Application of ceramic (alloy melting range must be higher than ceramic sintering temperature) —Ease of casting (higher ranges are more difficult to cast) |
Density (g/cm3) | Mass of alloy per volume of alloy | —Ease of casting (alloys with higher densities are easier to cast) —Cost of purchasing alloy (restorations containing alloys with higher densities are more expensive per restoration) |
Modulus (GPa) | Stiffness or flexibility of the alloy | —High moduli (stiff) are important for restorations where flexure causes failure —Low moduli (flexible) are important for ortho wires and partial denture clasps, where flexure is needed |
Strength (MPa) | Force required to break alloy (usually in tension, by pulling) | —High strength needed to avoid distortion or fracture of restorations, instruments. |
Hardness (kg/mm2) | Resistance to indentation | —Hardness of restorations to resist scratching (harder is better) —Hardness of restoration for cutting or adjustment (softer is easier) —Ease of polishing (softer is easier) —Wear of opposing teeth (if hardness of restoration is higher than enamel) |
Color is often used to describe an alloy, and it is often important to patients. Typically, alloys will be yellow or silver (often called “white” by metallurgists). Many dental personnel and patients assume that yellow alloys have a high content of gold and white alloys do not. However, the color of an alloy is not a good predictor of its composition or other properties. Yellow and white alloys may contain gold or not; it is impossible to tell from the color. This reality is often confusing for patients; the patient will equate yellow alloys with gold, and white with less expensive (“cheap”) content. The dental auxiliary can help resolve confusion for the patient.
Unlike pure compounds like sugar, alloys do not melt at a single temperature but have a melting range; the melting range reflects to some degree the melting points of the constituents of the alloy. If an alloy has a melting range of 950 to 1000° C and the alloy is heated gradually from room temperature, the first sign of liquid formation will be at 950° C. At 975° C, some of the alloy will be liquid, but some will still be solid. Once the temperature reaches 1000° C, all of the alloy will be liquid. When the alloy is cooled again, the reverse process will occur. The temperature at which all of the alloy melts on heating (1000° C in the example) is called the liquidus, and the temperature at which all of the alloy freezes on cooling (950° C in the example) is called the solidus. The liquidus and solidus of an alloy are important to the casting and soldering of the alloy. An alloy must be heated above the liquidus to be cast. The liquidus also determines the burnout temperatures and which investments are necessary for casting (Chapter 12). The solidus is important to soldering because if the soldering operation heats the alloy above its solidus, then the alloy loses shape, and the soldering procedure is a failure. The solidus is also important for ceramic-bonding alloys, which must be heated to high temperatures to fire the ceramic onto the alloy. The ceramic must sinter and bond to the alloy at a temperature below the solidus (≤50° C is needed, Chapter 14).
By definition, the density of an alloy is the mass in grams that occupies a volume of one cubic centimeter (g/cm3). Thus, if equivalent sized pieces of several types of alloys are weighed, the alloy with the highest density will weigh the most. The density of an alloy is a function of the densities of the elements that make up the alloy. The densities for dental casting alloys range from 4.5 g/cm3 for the titanium-based alloys to more than 18 g/cm3 for some alloys high in gold and platinum. The density of an alloy is important in the casting of the alloy and its final cost. High-density alloys are easier to cast because gravity can accelerate the molten metal more easily into the casting mold. Furthermore, because alloys are sold by mass, high-density alloys cost more because more mass is present in any given volume of restoration. Of course, the final cost of the alloy also depends on the cost per gram of the alloy; costs of allo/>
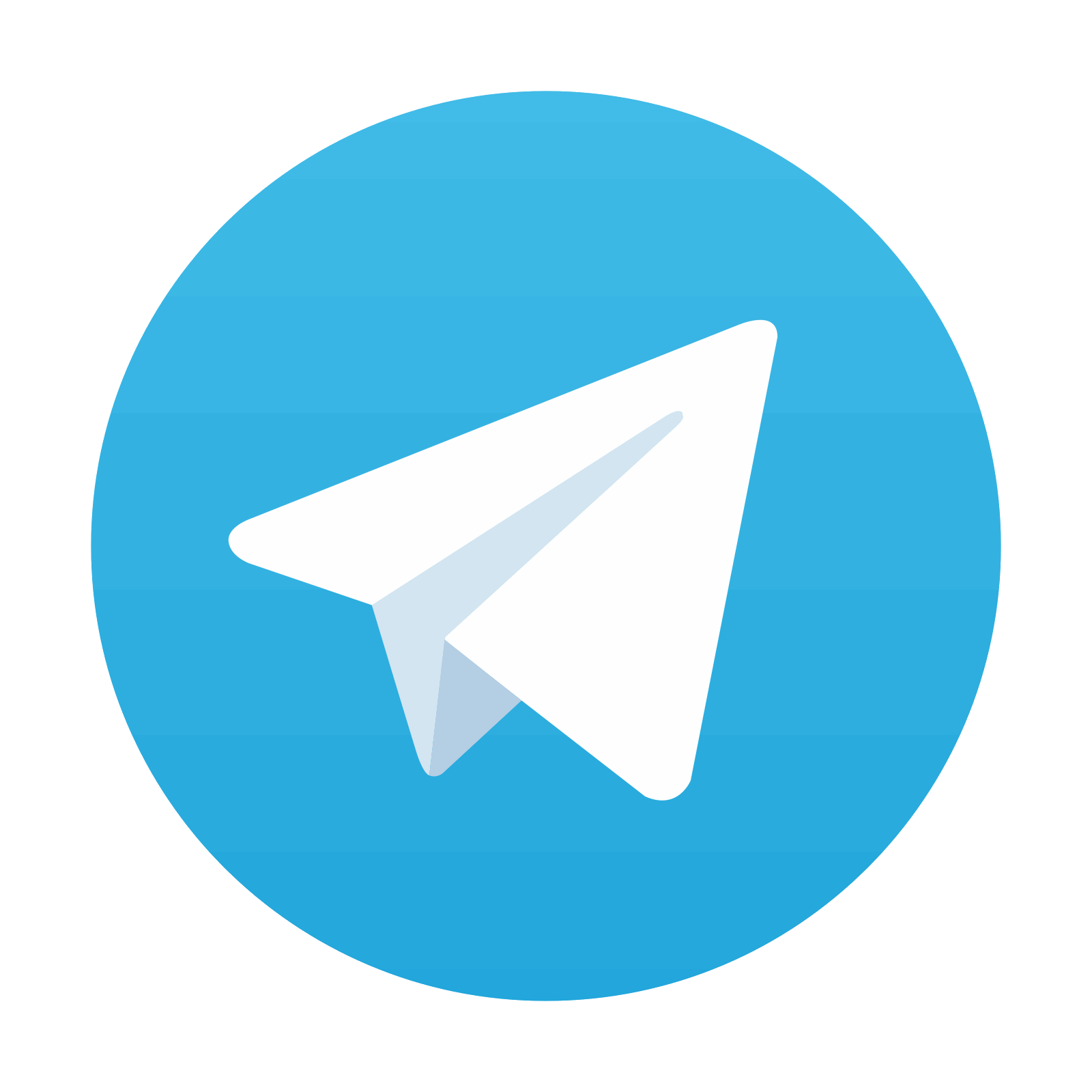
Stay updated, free dental videos. Join our Telegram channel

VIDEdental - Online dental courses
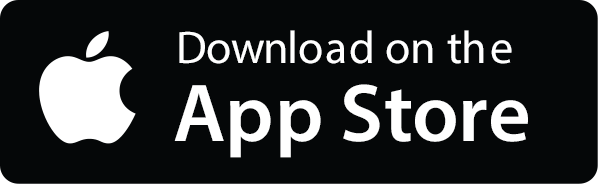
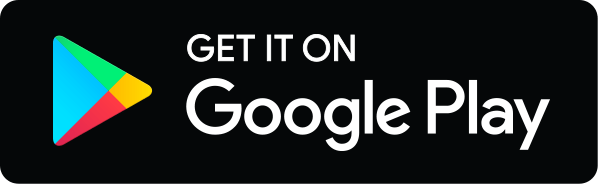