Chemical properties
Introduction
The oral environment is an aggressive environment. Materials may dissolve in the water that is present in saliva or release soluble components; they may corrode due to the presence of acids; they may discolour or break down due to absorption of substances from saliva; or they may tarnish and corrode.
All of these possibilities can adversely affect the chemical stability of the materials and limit their durability. The products released may have an adverse effect on the biological environment, both locally and systemically.
Dental ceramics are mostly compounds of oxygen, such as silica (SiO2) and alumina (Al2O3). These are chemically stable under most circumstances and immune from the oxidation process associated with electrochemical (or wet) corrosion. Degradation of ceramics generally involves a process of chemical dissolution. In contrast, metals are not immune to wet corrosion. With the notable exception of some noble metals, such as gold and platinum, metals are usually found in nature as compounds (principally oxides or sulphides), from which the metal is extracted. Corrosion of metals is, to all intents and purposes, the reversal of the reactions employed in the extraction process. Frequently, the corrosion product of a metal is very similar to the compound from which the metal was originally extracted. For instance, iron is extracted from naturally occurring iron oxide, and rust is simply hydrated iron oxide. Generally, polymers are not stable either, as many will burn once ignited, showing that the polymer oxidizes readily. However, polymer degradation is generally physio-chemical in nature, such as swelling, dissolution or covalent bond rupture. The latter may be due to heat or radiation and invariably results in a reduction in mechanical properties such as strength and toughness.
Degradation of polymers
Water sorption and soluble fraction
Many polymers used in dentistry, such as those used in resin composites, dentures and soft liners, are susceptible to absorption of solvents, particularly water, and the loss of soluble components. The solvent molecule forces the polymer chains apart, causing swelling. As the strength of the bond decreases, the polymer becomes softer, the glass transition temperature is reduced and the strength may be lowered. Nylon is particularly susceptible to water sorption and this is a significant contributing factor to limiting the life of a toothbrush. In the case of resin composites, water sorption is believed to be a contributory factor to the eventual discoloration of the restorations and the hydrolytic degradation of the resin–filler interface. Soft denture liners lose their flexibility due to the loss of water-soluble plasticizers, have an increased propensity to creep, and may even fracture under the osmotic pressure that can build up. Water sorption can have a significant effect on the properties of glass–ionomer cements, as too much or too little water can lead to loss of translucency or surface crazing respectively.
Generally, it is desirable for both the water sorption and soluble fraction of polymers to be as small as possible. This ensures that the polymer retains its characteristic properties, and that no components are leached out which might adversely influence the biocompatibility of the material.
The simplest method of assessing the water sorption and soluble fraction of a polymer is to monitor the weight change of a sample when immersed in water. The detailed analysis of the amount of water sorption by polymeric materials is complicated by the concurrent loss of water-soluble components such as residual monomers or plasticizers, as these two processes take place simultaneously, although at different rates. It is important in the characterization of these factors that the two processes are separated.
Both processes are controlled by the rates of diffusion of water and the water-soluble components through the material, such that the higher the rates of diffusion, the faster water will be absorbed and the faster the soluble fraction will be lost. It is important that any water that the sample has absorbed from the atmosphere has been removed prior to its immersion in water. To this end, samples must be stored in a desiccator until a constant weight is obtained.
The kinetics of a sorption and desorption cycle are shown in Figure 1.8.1. The peak in the weight of the sample in the first cycle is a consequence of the different rates of diffusion of water into the sample and diffusion of the soluble fraction out of the sample. Water is usually absorbed more rapidly than the soluble components are removed, such that there is an initial rapid weight gain until the sample is nearing saturation. At this point, the loss in weight due to the soluble fraction begins to show, as its release is aided by its dissolution into the absorbed water. The amount of water sorption and the soluble fraction can be calculated from the following:
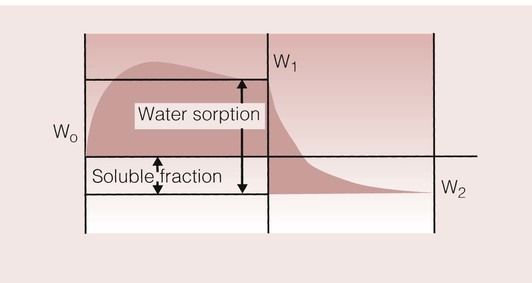
Weight%water sorption=(W1−W2)/W2×100

Weight%soluble fraction=(W0−W2)/W2×100

If the volume, V, at the end of the desorption cycle is calculated, and W2 replaced by V, then the water sorption and soluble fraction can be expressed in terms of µg/mm3, as recommended in the international standard (ISO/DIS 4049).
For most polymers, the amount of water sorption is approximately 30–50 µg/mm3. For resin composites, the value will be lower, due to the presence of the glass fillers, but if this is taken into account, the amount of water sorption into the resin should be in the range given above for polymers. Higher values for water sorption have been recorded for some resin composites, which may be associated with the presence of porosity, free space formed due to removal of the soluble fraction, hydrolytic breakdown of the resin–filler interface, or dissolution of the glass filler.
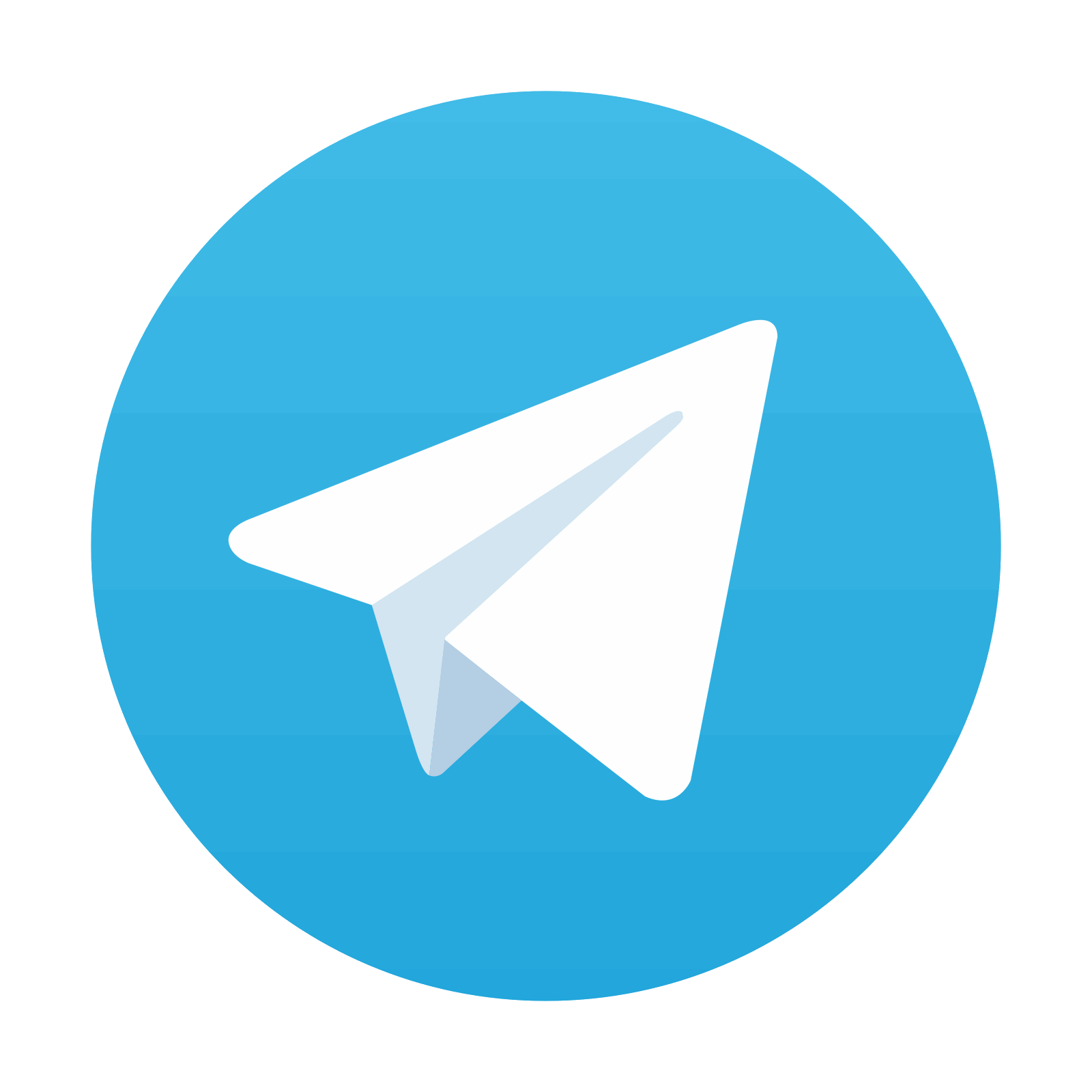
Stay updated, free dental videos. Join our Telegram channel

VIDEdental - Online dental courses
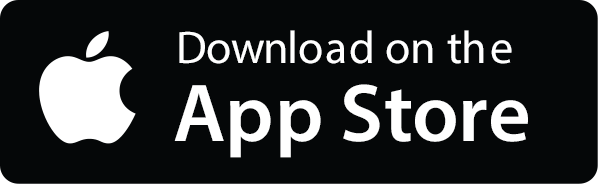
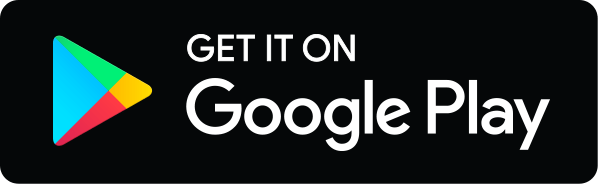