Fig. 7.1
Illustration of biomolecular transport across salivary cells and blood endothelial cells
The method of sample collection has also been shown to affect protein concentrations in saliva [24, 25]. This poses a challenge in standardizing saliva collection prior to developing diagnostic assays. Further difficulty is posed by factors that can cause intraindividual variations due to circadian fluctuations [26, 27]. As an example, salivary growth hormone levels are relatively higher in the morning compared with measurements made at night/evening/nightly?. Inherently these causes of intraindividual variation can lead to exacerbation of interindividual variations. A standardized protocol for collecting saliva specimens, which specifies the time and method of collection and restricts dietary intake for a fixed period before collection, could be used to minimize the effects of some of the variables causing intraindividual variation. Yet, it is not realistic to control certain other factors that affect saliva, such as prescribed drugs [3] that people may be taking to control other illnesses. Even differences in the normal bacterial flora in the oral cavity can cause changes to the protein content of saliva between or within individuals because bacterial enzymes catalyze reactions involving salivary proteins [28]. More so, saliva is susceptible to blood contamination: if there is a microscopic bleed in the oral cavity that contaminates saliva with blood, the direct mixing of serum proteins could lead to artificially raised protein levels in saliva [6]. This will obviously impact the measurement of salivary biomolecular profiles and must be taken into consideration in the development of saliva-based tests. However, the advancement of technology, particularly MS and nanotechnology, has greatly increased our ability to detect trace amounts of salivary constituents [4], making the prospect of developing salivary diagnostics a reality.
There are numerous saliva-based kits in the market to measure an array of biomolecules either relevant in disease or to detect contraband drugs, and these include cortisol (steroid), DHEA (adrenal gland secretion), estradiol, estriol (steroid sex hormone), estrone, progesterone (steroid hormone), testosterone (steroid hormone), SIgA (secretory immunoglobulin A), alpha-amylase, cotinine (nicotine exposure), C-reactive protein, human immunodeficiency virus (HIV), and drugs of abuse. Table 7.1 illustrates a catalog of some of the currently available saliva diagnostic tests. (Figure 7.2 shows the devices used in the authors’ laboratory [24].)
Table 7.1
Saliva tests and manufacturers
Application
|
Product name
|
Manufacturer
|
Web site
|
---|---|---|---|
Drugs of abuse
|
Oral Stat
|
American Bio MedicaCorporation
|
|
DDS®2 Mobile Test System
|
Alere
|
||
Human immunodeficientvirus (HIV)
|
OraQuick ADVANCE® RapidHIV-1/2 Antibody Test
|
OraSure Technologies
|
|
Hepatitis C
|
OraQuick® HCV Rapid Antibody Test
|
OraSure Technologies
|
|
Hormone
|
Saliva ELISAs
|
DRG
|
|
Caries
|
Dentocult® SM/LB
|
Orion Diagnostica
|
|
Periodontal diseases
|
MyPerioPath®, MyPerioID®
|
OralDNA® Labs
|
|
Human papillomavirus (HPV)
|
OraRisk® HPV salivary diagnostic test
|
OralDNA® Labs
|
|
Personal genome service
|
23andMe’s Personal GenomeService
|
23andMe
|
|
Ovulation test
|
Ovulation Scope
|
Quest Products
|
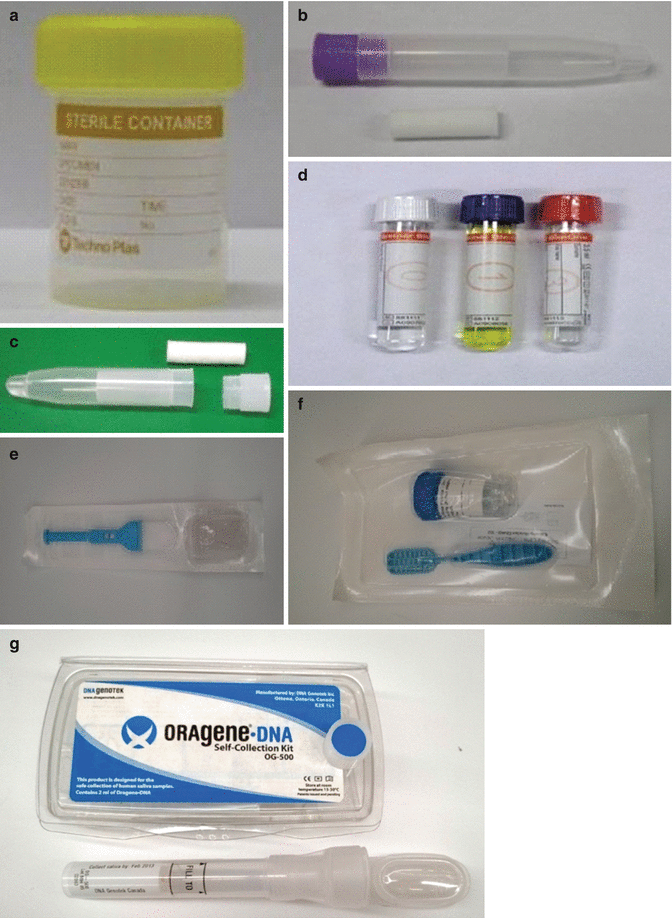
Fig. 7.2
The commercially available saliva collection devices that are used in our laboratory. (a) Drool collected in a sterile specimen container [24]. (b) Salimetrics® Oral Swab (SOS). (c) Salivette. (d) Greiner Bio-One, Saliva Collection System® (GBO SCS®). (e) Versi-SAL® saliva collection system. (f) DNA-SALTM saliva collection system. (g) Oragene® DNA Self-Collection Kit to isolate DNA and RNA from saliva
Biomarkers
A biomarker is “a characteristic that is objectively measured and evaluated as an indicator of normal biological processes, pathogenic processes, or pharmacological responses to a therapeutic intervention,” according to the Biomarkers Definitions Working Group of the National Institutes of Health [29]. This definition inherently describes the usefulness of biomarkers for diagnostic purposes by detecting the presence of pathogenic processes or the absence of normal biological processes in disease. It is not just diagnosis, but the potential of earlier diagnosis compared to current methods, that makes biomarkers a promising avenue for research.
The general clinical benefits of early diagnosis are numerous. Catching a disease in its infancy often gives doctors more treatment options to pursue. The increased treatment options plus the additional time afforded to make a decision enable patients to make better, well-considered choices in cooperation with their doctors, thus furthering the ethos of patient autonomy in medical practice. Beginning treatment earlier in the course of disease also often greatly improves its efficacy. All of these factors contribute to better patient outcomes and satisfaction. Biomarkers play important clinical roles in diagnosis, stratification, prognosis, and therapy for many diseases and promise to feature in many more. There are various types of biomarkers, including DNA, RNA, proteins, and cells. In terms of biological processes, they can also be classified as inflammatory biomarkers, cancer biomarkers, etc.
This chapter is dedicated to three main diseases in the oral cavity, dental caries, periodontal diseases, and oral lesions/cancers, which have inflammation as a common etiology factor. Each section is dedicated to highlighting the epidemiology, clinical problem, and the treatment strategies. We also highlight where possible biomarkers that are either clinically used or still within a research phase.
Inflammatory Biomarkers
Inflammatory biomarkers have been identified as candidates for dental caries, periodontal diseases and oral cancer because of the growing evidence of a reciprocal, causal relationship between these diseases and either acute or chronic inflammation [30]. Transcription factors common to both cancer and inflammation have been shown to produce inflammatory mediators that enhance DNA damage, oncogenic activity, immune evasion, angiogenesis, and metastasis, all of which contribute to cancer development [31]. Because of the early involvement of host inflammation in the cancer process, inflammatory biomarkers are useful in enabling early detection of cancer [32]. The inflammatory events in oral cancer have many potential triggers: tobacco, alcohol, etc. These triggers cause the activation of inflammatory transcription factors such as NF-κ(kappa)β, STAT3, and AP-1 [31]. The activated transcription factors can then mediate a number of effects: expression of oncogenes, silencing of tumor suppressor genes and the production of inflammatory chemokines, cytokines, and prostaglandins [31]. It is therefore important to investigate inflammatory biomarker changes in a disease state compared with a healthy state.
Body Fluids as Biomarker Sources
Biomarkers can be measured in a wide range of biological media: blood, urine, cerebrospinal fluid (CSF), sputum, feces, saliva, body tissues, etc. Blood testing generally involves sampling of blood from a superficial arm vein by venipuncture. This is one of the most common biological fluids currently used in a clinical laboratory setting. The close contact and subsequent substance exchange of blood with most organs make it ideal for detecting underlying pathology almost anywhere in the body. Blood biomarkers are utilized for a plethora of diseases—CA-125 for ovarian cancer [33], troponin I for myocardial infarction [34], immunoglobulin A for PD, and miRNA-21 for oral cancer [35], to name a few. While listing the uses of blood tests is outside the scope of this chapter, it is evident that blood tests are currently part of the standard clinical practice when it comes to clinical diagnosis or stratification of disease. CSF is another diagnostic fluid often used for standard clinical practice, though perhaps not as frequently as blood. Examination of CSF is the gold standard for the diagnosis of several central nervous system disorders, e.g., meningitis [36, 37], subarachnoid hemorrhage [37, 38] and carcinomatous meningitis [37].
Similar to blood, the use of urine is also extremely common in clinical practice. Urine output measurements for kidney function, urine dipstick tests and urinalysis are all routinely performed in hospitals and clinics. Many biomarkers in urine are derived from blood and serve as a proxy to noninvasively observe systemic events. Proteins are ultrafiltrated in the kidney glomeruli based on size and charge [39] and then mostly reabsorbed in the proximal convoluted tubules [40]. Nevertheless, a large number of blood serum proteins are still present in urine, albeit at a much lower concentration. These serum proteins alongside exosomal apical membrane proteins [41] and exocytosed lysosomal proteins [42] make up a very rich urine proteome—consisting of 1,543 different proteins [43]—with a lot of diagnostic potential.
Urine collection, despite being embarrassing for some, can be done relatively easily, in large amounts and noninvasively if catheterization is not required. This makes it an excellent diagnostic medium. Finally, proximity dictates that urine is particularly suitable for the diagnosis of renal and postrenal pathologies [44–67]. All these factors point toward the inevitable use of urine diagnostic biomarkers in the coming years. Similarly, saliva is used in clinical practice for monitoring and diagnostic purposes. Monitoring roles include that of alcohol, drugs of abuse [68], estrogen [69], progesterone, cholesterol [69, 70], as well as cardiac-specific biomarkers. It is also used to diagnose Cushing’s syndrome [71], HIV [72], prostate cancer [69], and breast cancer [73]. Saliva avoids the classic problems associated with urine specimen collection, which can be distressing if catheterization is involved and the additional downside of embarrassment due to violation of privacy. The use of saliva sampling is beneficial not only because it simplifies the testing of poorly compliant patients (e.g., children or psychiatric patients), but also because it improves overall patient compliance. Enhanced compliance for routine, follow-up, and monitoring tests would contribute to better health-care outcomes.
Dental Caries
Dental caries (holes in the teeth), also known as tooth decay or the formation of cavities, is a bacterial infection that causes demineralization and destruction of the hard tissues of the teeth (enamel, dentin, and cementum) (Fig. 7.3). Dental caries is a result of the production of acid by bacterial fermentation of food debris accumulated on the tooth surface. If tooth demineralization exceeds remineralization (the latter is driven by factors such as the amounts of calcium and fluoride present in toothpaste or in the drinking water), this then promotes hard tissues to progressively break down, producing dental caries. Depending on the extent of tooth destruction, various treatments can be used to restore tooth function and aesthetics, but there is no known method to regenerate large amounts of demineralized tooth structure. Instead, dental health organizations advocate preventive and prophylactic measures, such as regular oral hygiene examinations by professional dental staff and dietary modifications to avoid dental caries.
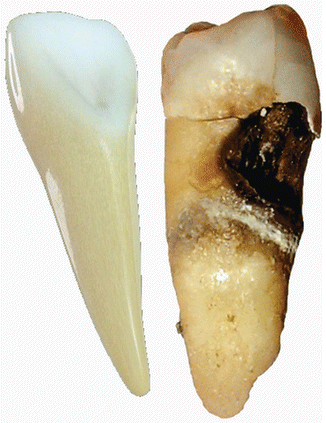
Fig. 7.3
The comparison of a healthy tooth (left) and a decayed tooth (right)
Epidemiology
Dental caries is one of the most common diseases in the modern world. About 36 % of the global population (2.43 billion people worldwide) have been diagnosed with dental caries or have had teeth removed due to dental caries [74]. Overall, the prevalence of dental caries is relatively low in the developed countries compared with developing countries due to the availability of preventive measures and better oral hygiene practices. Countries in Latin America and in the Middle East have the highest rates of dental caries, while China has the lowest rate of dental caries because of mandatory addition of fluoride to the water supply. However, the excess addition of fluoride has caused other dental problem such as fluorosis [75].
Causes, Signs, and Symptoms
Prior to the establishment of modern dentistry, dental caries was believed to be caused by “tooth worms” in some ancient civilizations [76]. It was not until the seventeenth century that the medical community started to reject the idea that “tooth worms” caused dental caries [77]. Due to the advancements in technology (improved microscopy), scientists began to realize the real cause of dental caries. During the golden age of microbiology in the late nineteenth century, American oral microbiologist Willoughby D. Miller proposed a theory that caries is caused by the acids produced by oral bacteria as a result of the fermentation of sugars [78]. Since mineral is the main component of a tooth, demineralization and remineralization between the teeth and saliva are constantly occurring in our oral cavity, and if demineralization exceeds remineralization, this then leads to dental cavities. The pits and fissures of the teeth and other regions that are hard to clean with either saliva or a toothbrush are hot spots for caries, since residues of food accumulate easily, giving the bacteria more nutrition to live on. Certain types of bacteria can ferment carbohydrates (e.g., sucrose, glucose, and fructose) which are left in the oral cavity through a glycolytic process and produce acid that will lower the pH and promote demineralization. Among all the bacteria present within the oral cavity, those which can produce a high level of acid through sugar fermentation have the ability to survive in a low pH environment and cause dental caries. Streptococcus mutans and Lactobacilli are believed to be the two main bacteria involved in the formation of caries. When they colonize the teeth and gum area, they form a biofilm called plaque. Plaques serve as the frontier of demineralization. At the initial stage of caries, there is often a white-spot lesion on the surface of the enamel. This white spot is a sign of extensive demineralization of the tooth. As the demineralization continues, the white spot may turn brown before the decay becomes an irreversible cavitation. The cavity will become more obvious as the decay carries on. The color and texture of the affected areas of the tooth will get darker and softer. Sharp pain will occur when the decay passes through enamel and dentin and reaches the pulp where the blood vessels and nerves are located. If left untreated, the bacteria will eventually overwhelm the pulp tissue and cause infection.
Pathophysiology
Virulence factors released from various oral bacteria residing either in the tooth or in the oral cavity cause degradation of host tissue by directly or indirectly activating host immune response. The latter initiates the release of biological mediators from host cells, leading to the host tissue destruction [79]. A number of bacteria-derived enzymes, such as collagen-degrading enzymes, elastase-like enzymes, trypsin-like proteases, aminopeptidases, and dipeptidyl peptidases, are recognized as important players in tissue destruction. Therefore, host and bacteria-derived enzymes, proteins, and other inflammatory mediators hold great promise as salivary biomarkers for the diagnosis of dental caries. Salivary phosphopeptides appear to be involved in the remineralization processes, delaying the loss of tooth structure [80]. As an example, when the demineralization is caused by acid on the outer part of the tooth enamel, there is no regeneration of this structure due to the lack of cells with enamel-producing ability (ameloblasts). But when the decay penetrates the enamel, dentin can react to the caries because of the existence of odontoblasts, which can continuously produce dentin at the border between dentin and pulp. These salivary proteins serve as key biomarkers in the development of pathogenic process: inflammation, collagen degradation, and bone turnover.
Current Diagnostics and Treatment Strategies for Dental Caries
As dental caries is reversible before the formation of cavity, early diagnosis of dental caries holds the promise for early interventions. However, caries are often unnoticeable until they penetrate the enamel and cause pain to the patients, hence making early identification very difficult. The current diagnosis for dental caries relies heavily on visual inspection and dental radiography performed by a trained dentist, and these requirements have made diagnostics challenging in some developing countries due to the associated costs.
A tooth extraction may be the most traditional treatment option for dental caries since it requires minimal equipment to perform. In the modern day dental practice, removal of the decayed tooth is performed if the tooth has been destroyed by the decay and will lead to further complications in the future. Dental restoration is now a more common treatment option for most dental caries. After removal of large portions of the decayed part of an infected tooth, restorative materials such as dental amalgam, composite resin, porcelain, or gold may be used to fill in the decayed area.
Saliva Proteomics for Dental Caries
The salivary defense system includes salivary proteins, which play important roles in maintaining the health of the oral cavity and preventing caries, as stated by Mazengo et al. [81]. Saliva surrounds soft and hard oral tissues and is in direct contact with the teeth. This gives saliva the advantage of proximity to serve as a diagnostic medium for dental caries. Currently, there is no established saliva-based method to diagnose dental caries in a clinical setting. However, saliva can be easily used to monitor the risk of caries [82]. An increase in salivary phosphopeptide levels (PRP1/3, histatin-1, and statherin) was associated with the absence of dental caries, emphasizing the importance of these peptides in the maintenance of tooth integrity [80]. In a study investigating early childhood caries, it was found that a higher number of proline-rich proteins correlated with caries-free individuals, substantiating the protective role of this type of protein [80]. Tulunoglui et al. demonstrated that salivary calcium concentrations are significantly decreased in children and teenagers who previously have had dental caries [83]. They also found that the total protein concentrations and antioxidant concentrations were higher in people with underlying caries compared with individuals with no previous history of caries. Ayad et al. demonstrated that IB-7 (a phenotype of proline-rich proteins) was higher in the saliva of caries-free individuals than in people who had previously suffered from caries [84]. Due to the fact that dental caries is mainly caused by mutans streptococci, the level of salivary mutans streptococci was also used to evaluate the caries risk. The association between salivary mutans streptococci and dental caries varied between studies [85–87]. Zhang et al.demonstrated that in children aged 6–7 years of age, salivary mutans streptococci counts were a good indicator for dental caries [87], while another study had a different conclusion [86]. A possible reason for conflicting data may be due to the fact that besides mutans streptococci, there are other bacteria that can cause caries, which may vary between individuals studied. Table 7.2 gives an overview of the salivary biomarkers that are used in a research phase to diagnose dental caries [83]. Further laboratory and clinical studies are required to truly understand the clinical utility of these salivary biomarkers before they can be implemented in a standard dental practice.
Table 7.2
Salivary biomarkers in a research phase to diagnose dental caries
Salivasample type
|
Findings
|
Reference
|
---|---|---|
Acid-stimulated parotid gland saliva
|
No differences in the salivary levels of proline-rich, parotid acidic, and double-band parotid proteins were found between subjects with and without caries
|
[88]
|
Unstimulated whole-mouth saliva
|
The total protein concentrations increased (0.65 g/dL vs. 0.35 g/dL) in boys aged 7–10 years with caries compared with boys without caries
|
[83]
|
Unstimulated whole saliva
|
A 17 kDa protein was found to be (not characterized) increased in patients with dental caries
|
[89]
|
Tongue surface scrape
|
Salivary mutans streptococci concentration was increased in people with dental caries
|
[85]
|
Future Perspective
The current diagnosis for dental caries is largely based on visual inspection and often not made until caries become irreversible, warranting reliable early diagnostic methods. As the medium that is in direct contact with the teeth, naturally saliva has a strong potential to be used in dental caries. The noninvasive nature of saliva collection provides another advantage in contrast to the traditional method (dental explorer) in which the pressure from the dental explorer can create a cavity even if the demineralization may still be reversible. Diagnostic tests that utilize oligosaccharide biomarkers in saliva to predict caries formation in children are under development [90].
Periodontal Disease
After dental caries, the most common oral disease is periodontal disease. Gingivitis and periodontal disease may affect 80 % of the adult population, making it one of the most prevalent oral diseases. Periodontal is a combination of two meanings derived from the Greek language, “peri” for around and “odontal” for the teeth. Hence, this is a disease of the structures surrounding the teeth, which include the gums, the cement, the periodontal ligament, and the alveolar bone. Teeth are embedded in the bone and cushioned in the socket by the periodontal ligament; the ligament spans from the bone to the root of the tooth where there are some ligament fibers above the bone, which hold the gums tightly to the tooth.
Periodontitis presents in three ways: initially the pocket depth increases due to swelling and bone loss, followed by the bleeding of the gums, and finally supporting bone is reduced around the tooth. The first stage of periodontal disease is gingivitis, the infection of the gums. In infected patients, plaque (a sticky, colorless bacterial deposit) and tartar (a crusty deposit) cause irritation of the gums and bone loss; this leads to the loss of tooth support structure. This can ultimately lead to the loss of teeth, which is readily seen in adult populations around the world. The bone and gum should ideally fit snugly around the teeth; however, when periodontal disease is present, the supporting tissue and bone is destroyed, forming pockets around the teeth. Over time, these pockets deepen.
Epidemiology
As of 2010, chronic periodontitis affected more than 750 million people or about 11 % of the global population. In the USA, one in two American adults aged 30 years and above has periodontal disease, according to the Centers for Disease Control and Prevention (CDC) [91]. It was documented that 47.2 % or nearly 65 million American adults have early, moderate, or severe periodontitis. In adults over the age of 65 years, the prevalence was 70 %. The disease was more prevalent in men than in women, with the distribution 56.4 and 38.4 %, respectively. Other factors that were found to correlate with high prevalence were the patients’ smoking status, whether they lived below the poverty line, and whether they had a high school education.
Approximately 800,000 dental implants are used in the USA annually, with this steadily increasing at a rate of 25 % as of 2006 [92]. Smoking, diabetes, hormonal changes in women, medications, and genetic susceptibility are a few of the risk factors that predispose people to periodontal disease. There seems to be increasing evidence that chronic periodontal disease may be associated with smoking, since the risk has also been found to be elevated in smokers [93, 94]. Diabetes affects the body’s ability to use blood sugar, thereby making diabetic patients more susceptible for developing infections that do not readily heal. Hormonal changes in women, which occur during puberty, monthly menstruation, pregnancy, and menopause, can make gums sensitive, whereby increasing the risk for gingivitis. Furthermore, illnesses that may compromise the immune system such as AIDS and cancer may affect the condition of the gums. Not forgetting, poor oral hygiene is a contributing factor, and one has to brush and floss on a daily basis using the correct technique to reduce the chance of gingivitis. Family history of dental disease also plays an important role in oral health. There is evidence that stress is associated with periodontal disease, and certain lifestyle events, such as emotional and financial stressors [95] are associated with increased disease susceptibility. In recent studies, it has been found that people with gum disease are more likely to develop heart disease or type 2 diabetes. Furthermore, it has been found that women with gum disease are more likely to deliver preterm babies with lower birth weights than women without gum disease. When the body’s immune system is triggered due to periodontal disease, the body releases prostaglandins, which, at increased levels, can cross the threshold and induce labor [96, 97].
Causes, Signs, and Symptoms
Numerous factors give rise to periodontal disease, such as bacteria and dental plaques. It has been estimated that approximately 600 different bacteria are capable of colonizing our mouths. The bacteria associated with periodontal diseases are predominantly gram-negative anaerobic bacteria, which can include Porphyromonas gingivalis and Aggregatibacter actinomycetemcomitans. The bacteria possess virulence factors that allow for colonization to occur in the oral cavity as well as produce harmful by-products that cause tissue damage [98].
A sticky, soft film forms on the surface of the teeth and matures over the duration of a day. This accumulation and eventual hardening results in the formation of calculus or tartar. The dispensation of calculi in the teeth helps form uneven and rough surfaces, further encouraging the bacteria to grow. The unevenness and irregularity of these surfaces are niches that are ideal for the growth of bacterial colonies. Over time, these irritants will destroy the tissues that attach the gums to the teeth, allowing the gums to move away and forming small pockets that can undergo further plaque accumulation. These processes ultimately lead to bone destruction unless interventions occur.
Signs of periodontal disease include red, inflamed, and tender gums that bleed easily. In addition, bad odors, loose and sensitive teeth, pus, teeth depressed into sockets, and teeth that seem to move over time are noteworthy signs. Figure 7.4 presents the clinical manifestation of gum disease over time. The left side is the healthy gums, whereas the right side shows the diseased conditions. The periodontal probe is shown in black, which is adjacent to the teeth (Fig. 7.5). The tip of the instrument is placed into the gingival sulcus, which is the region between the tooth and surrounding tissue. The probe is kept parallel to the contours of the root of the tooth and inserted to the base of the pocket to measure the depth. The average healthy pocket depth is around 3 mm with no bleeding upon probing. It can be seen that the pocket depth gets deeper as the stage of periodontitis progresses toward the advanced stage.
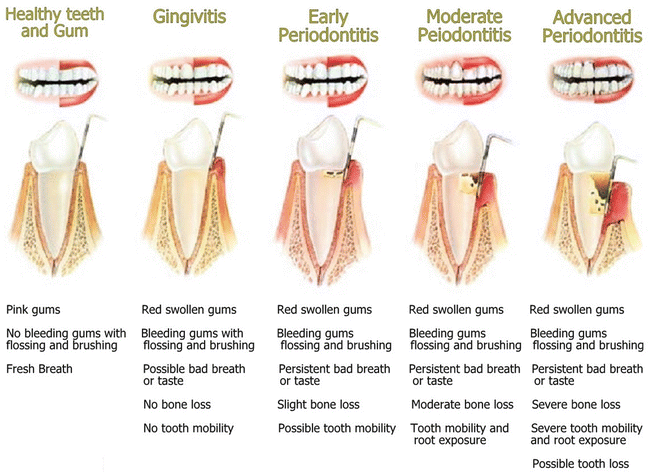
Fig. 7.4
The different stages highlighting the progression of periodontal disease (Reprinted with permission from the Advanced Institute of Oral Health, Brentwood, TN)
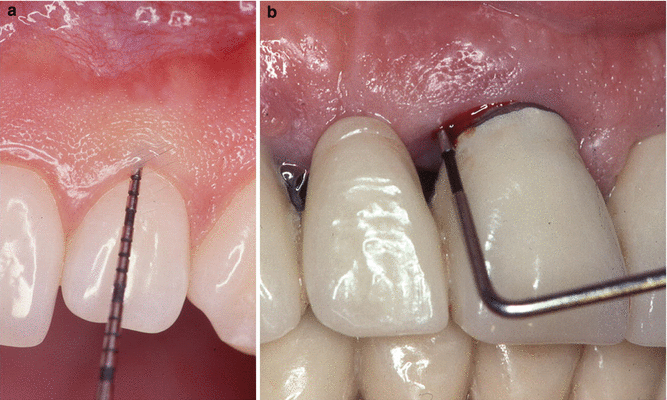
Fig. 7.5
The clinical evaluation of periodontal disease by probing. (a) Healthy gum and (b) diseased gum, bleeding upon probing (Reprinted with permission from the Journal of the California Dental Association, 2013 February; 41(2):119–24)
There are four stages in the progression of gum disease, each with its own characteristic signs and symptoms. These stages are gingivitis, early periodontal disease followed by moderate and severe periodontal disease. Gingivitis is the initial and reversible stage of gum disease caused by inflammation. Normal, healthy gums are pink, tight, and smooth, allowing food to pass and move into the space between our teeth and cheek. When a person has gingivitis, the gums become red and inflamed and bleed easily, which is particularly noticeable during brushing. When the gums are inflamed, food can be seen to accumulate around the gums and pack in and around the teeth. The characteristic redness and swelling of the gums is due to an increased blood supply, which is the body’s immune response against an influx of bacteria. If gingivitis is left untreated and uncontrolled, there is progression to early periodontal disease, which is irreversible. This stage is classically shown by 4–5 mm pockets, early bone loss, and the loss of attachment of the gums around the tooth, which may be seen by X-ray. The importance of the root of the tooth is that it receives its blood supply from the ligament. When the ligament is detached during periodontal disease, the blood supply is lost, causing the root to die. The root surface becomes rough and is prone to bacterial infection and accumulation over time. Moderate periodontal disease is characterized by 5–6 mm pockets as well as bone loss, which is visible on X-ray. Other signs include separation of the front teeth, pus, and bad odors, to name a few. Ultimately, this leads to severe periodontal disease characterized by pockets 6 mm and larger as well as little or no bone structure supporting the teeth.
Pathophysiology
Following microbial plaque formation, an acute exudative inflammatory response begins within a few days, causing gingival fluid and neutrophils to increase locally. Fibrin deposits destroy collagen at these initial stages. Early lesions are noticeable by the infiltration of lymphocytic cells as well as monocytes and plasma cells. Over time, these lesions become chronic with noticeable presence of plasma cells and B lymphocytes. With this progression, pockets are formed where the gingiva separates from the tooth. The depth of the pockets increases with brushing, flossing, and chewing. If continued inflammation persists, the periodontal ligaments break down, which leads to the destruction of the local alveolar bone, resulting to teeth falling out.
There appears to be a general consensus that periodontal destruction is host-mediated through the release of pro-inflammatory cytokines by tissues and immune cells in response to bacterial infection, products, or metabolites. Interleukin-1 beta (IL-1β[beta]) and tumor necrosis factor alpha (TNF-α[alpha]) have shown increased levels in periodontal sites during inflammation and tissue destruction. There is also a marked decrease in these biomarkers following periodontal therapy when gums return to a healthy state.
Current Diagnostic Strategies and Treatments for Periodontal Disease
One sign that dentists look for in periodontal disease is bleeding upon probing. A visual assessment is made of the gingival tissue and sulcus by pocket probing. The absence of bleeding is indicative of the absence of periodontal disease, whereas the presence of bleeding upon probing is used as a predictor of the disease. Currently, there are two salivary diagnostic kits that can detect the bacteria causing periodontal disease [99]. The first test identifies the type and concentration of specific periodontal pathogenic microbes in the patients’ saliva sample, which then allows the clinician to determine the appropriate antimicrobial therapy. The second test detects genetic susceptibility in individuals to periodontitis by analyzing genetic variations that affect the production of inflammatory cytokines, i.e., interleukin-1a and b. Both tests use DNA polymerase chain reaction (PCR) to analyze the samples and require a few days to process and analyze before the patient obtains the results. A pitfall of these combined methods is the duration for turnaround time and the fact that they lack the ability to determine when periodontal disease will occur.
Human saliva contains biomarkers that are specific for the unique physiological aspects of periodontitis (Table 7.3), and qualitative changes in the composition of these biomarkers could provide diagnostic opportunities [80, 81, 87]. As an example, saliva holds a number of periodontal proteomic markers from immunoglobulins to bone remodeling proteins. Immunoglobulins (Ig) are antibodies produced by the immune system, which help defend against a number of pathogens. They are not only present in blood but also in saliva. IgA, IgG, and IgM subtypes found in the mouth affect the microbes by interfering with the adherence of bacteria or by inhibiting their metabolism. Patients presenting with periodontal disease have higher salivary concentrations of these immunoglobulin subtypes. Post-periodontal treatment, these levels are notably reduced. Therefore, the use of IgA, in particular, has been brought to the fore, as it provides a clinical means to identify individuals who have the potential to develop periodontal disease as well as to determine the treatment response.
Table 7.3
Salivary biomarkers associated with periodontal disease
Biomarkers
|
Mode of action
|
Type of periodontal disease
|
Reference
|
---|---|---|---|
Immunoglobulins(IgA, IgM, IgG)
|
Interfere in adherence and bacterialmetabolism
|
Chronic, aggressive
|
|
Mucins
|
Interferes with bacterial colonization
|
Aggressive
|
|
Lysozyme
|
Regulates biofilm accumulation
|
Chronic
|
|
Lactoferrin
|
Inhibits microbial growth
|
Aggressive
|
|
Histatin
|
Neutralizes lipopolysaccharides
|
Chronic, aggressive
|
|
Peroxidase
|
Interferes with biofilm accumulation
|
Chronic
|
|
C-reactive protein
|
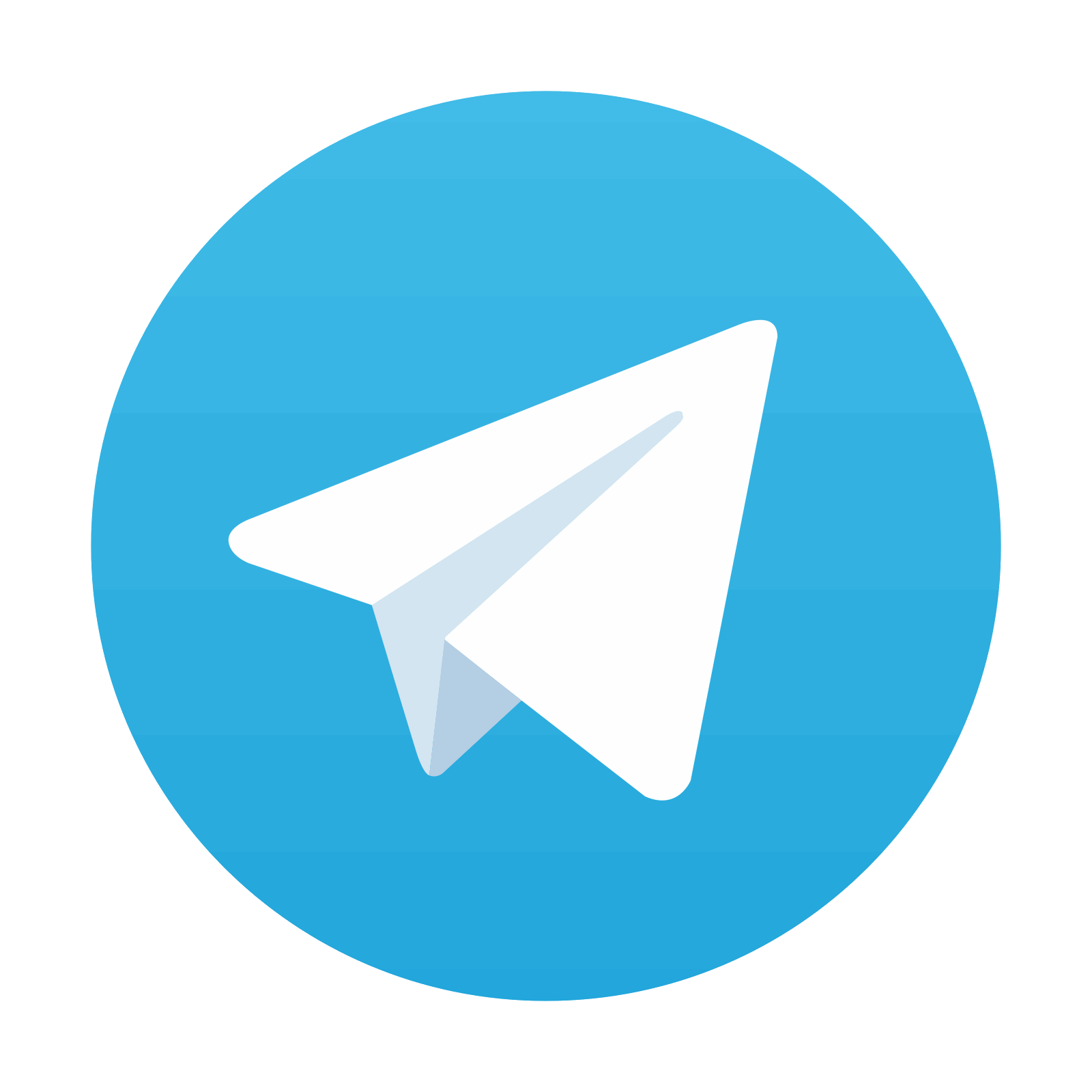
Stay updated, free dental videos. Join our Telegram channel

VIDEdental - Online dental courses
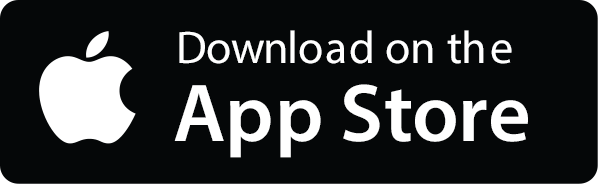
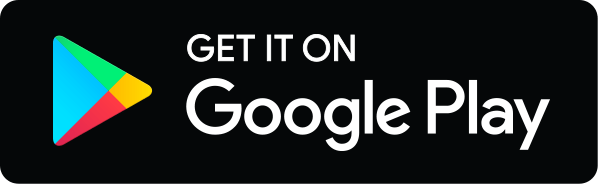