Fig. 4.1
The landmarks in the development of salivary diagnostics. (1) Salivary rheumatoid-specific antibody test is used for rheumatism diagnosis, whereas salivary uric acid test is used for gout diagnosis and monitoring. (2) The disclosure of serum components in saliva opened doors to the development of diagnostic test for oral and systemic diseases through saliva specimen. (3) MyPerioPath® is a salivary test that identifies the type and concentration of specific perio-pathogenic bacteria that are known to cause periodontal disease. MyPerioID® salivary test identifies individual genetic susceptibility to periodontal disease. (4) OraRisk® HPV test recognizes in saliva the type(s) of oral HPV that could potentially lead to oral cancer. (5) Salivary tumor marker panels were developed to aid in specific cancer diagnosis. (6) There is a specific salivary DNA test for a set of bacteria that correlates with pancreatic cancer. CF cystic fibrosis, CRP C-reactive protein, Igs immunoglobulins, HIV human immunodeficiency virus, HPV human papillomavirus, SalEst test salivary estradiol test
In 2002, the application of salivary diagnostics for oral and systemic diseases received a major boost as a result of funding support by the National Institute of Dental and Craniofacial Research (NIDCR). This initiative was designed to establish collaborative research between engineers and scientists to develop point-of-care diagnosis platforms for rapid detection and analysis of salivary biomarkers [10]. Therefore, to support clinical applications of saliva, efforts have been conducted in the development of five salivary areas: genomics, transcriptomics, proteomics, metabolomics, and microbiomics [11]. Salivary metabolome offers a promising clinical strategy by characterizing the association between salivary analytes and a particular disease. In 2010, Sugimoto et al. [12] identified oral, breast, and pancreatic cancer-specific profiles using capillary electrophoresis time-of-flight mass spectrometry (CE-TOF-MS). Regarding salivary microbiome, in 2012, Farrell et al. [13] observed variations of salivary microbiota related to pancreatic cancer and chronic pancreatitis. As a result of the advancements in novel technological means (e.g., quantitative real-time polymerase chain reaction (qRT-PCR), mass spectrometry approaches, bioinformatics tools, and microfluidic techniques), point-of-care (POC) devices and rapid tests for saliva have been integrated as part of disease diagnosis, clinical monitoring, and patient care management [14]. There are already two US Food and Drug Administration (FDA)-approved products (Fig. 4.1) that use saliva for diagnostic purposes: for human immunodeficiency virus (HIV) antibody detection (OraQuick ADVANCE Rapid HIV-1/2 Antibody Test, OraSure Technologies) and for the measurement of estradiol (SalEst, Biex Inc.) for the prediction of premature birth [11]. In addition, several saliva laboratory-based tests are available to assess the presence of drugs, hormones, viruses, and other microbial components (Fig. 4.1). For instance, to assess periodontal status of a patient in a periodontitis setting, there are tests for periodontal pathogens (MyPerioPath, OralDNA Labs, Brentwood, Tenn.) and for assessing the patient’s genetic risk of developing the disease (MyPerioID PST, OralDNA Labs). Another important salivary test is the OraRisk HPV test (OralDNA Labs), which identifies the type or types of oral human papillomavirus (HPV), a mucosal virus that may give rise to oral cancers [3, 15].
The importance of salivary proteome, transcriptome, and genome in the discovery, development, and validation of disease biomarkers will be critically analyzed in more detail as follows.
Genomics and Transcriptomics: DNA and RNA Analysis
Salivary genomics and transcriptomics arise as interesting research fields once saliva provides a convenient source of microbial and human DNA and RNA, owning highly informative and discriminatory evidences of physiological and pathological conditions. This awareness coupled with the ability to harness genomic and transcriptomic information by high-throughput technology platforms, such as microarrays and next-generation sequencing, positions salivary genomics and transcriptomics in the detection of specific disease states [16, 17].
Methodologies Used in Salivary Genomics and Transcriptomics
The starting point in the genomic and transcriptomic analysis of human saliva is sample collection followed by the extraction of salivary RNA or DNA. The extraction of the nucleic acids comprises cell disruption with a buffer that includes a nonionic detergent, and the resultant lysate is used for the isolation of DNA or RNA—most often by organic and/or solid-phase extraction. There are several kits available that allow this extraction (e.g., Oragene self-collection and QIAamp viral RNA kits), and more interestingly, there already exist kits that perform the RNA and DNA co-extraction (e.g., Promega DNA IQ™ System) [18–21]. The co-extraction of the two nucleic acids minimizes sample requirements and can eliminate the need for two separate extractions when the aim of the study is genomic and transcriptomic analysis [18]. For RNA profiling, the supernatant phase of saliva (CFS) is typically used instead of whole saliva (WS) since saliva naturally contains microorganisms, oral epithelial cells, and extraneous substances that are minimized in CFS samples [22, 23]. Salivary DNA or RNA often contains large amounts of bacterial nucleic acids, and the relative proportion of human DNA or RNA might be difficult to determine [24].
After the isolation of the nucleic acids, it is important to assess the integrity and quality of the sample—typically by reverse transcription followed by polymerase chain reaction (PCR) or quantitative PCR (RT-PCR or RT-qPCR) [20, 25]. This step also allows the amplification of DNA and RNA, which is important for further studies. In order to identify the genome or transcriptome profile of the isolated samples, two major technologies are employed: next-generation sequencing (NGS) or microarray platforms [24, 26–28]. NGS is a term used to describe a number of different modern sequencing technologies (e.g., Roche 454 sequencing) that grant the sequence of DNA and RNA more quickly and economically than the previously used Sanger sequencing. Moreover, the recent advances in NGS technologies engender an exhaustive analysis of genomes and transcriptomes [29].
In the last years, efforts have been made to improve the current standard operating procedures either on salivary genomics or salivary transcriptomics. One of the promising advances relies in a streamlined direct saliva transcriptome analysis (DSTA), which is characterized by the use of CFS instead of isolated mRNA for saliva transcriptomic detection. All the following procedures (including processing, stabilization, and storage of samples) are performed at ambient temperature without a stabilizing reagent [30]. With these new technologies available, the salivary DNA and RNA became interesting samples for biomarker researches and biomedical applications.
Biomedical Applications of Salivary Genomics
DNA holding the information of an individual’s genome, oral microbiota, and infecting DNA viruses are present in saliva and also reflect the presence or absence of specific gene mutations and methylation status. Thus, salivary genome analysis can provide insights into the presence of pathogens and alterations in gene profiles that directly reflect pathological conditions such as cancer [31]. Over the last decade, it has been proved that genomic DNA extracted from saliva may be used in a clinical and research environment since salivary DNA is equivalent in quantity and purity to those obtained from blood [32–34].
Salivary DNA has been investigated to assist clinicians in the early diagnosis of patients with a family history of diseases such as Parkinson’s and other life-threatening diseases, including certain types of cancer and cystic fibrosis [35]. One of the major aims of salivary genomics is to find a gene or a gene mutation or another type of alteration (such as aberrant methylation) underlying a specific disorder (genotyping analysis and mutational screening of disease-causing genes).
On the other hand, salivary DNA can be used to identify specific bacterial species that triggers an inflammatory response [36]. Thus, DNA tests can identify the bacterial targets for therapy and assist in monitoring the concentration of these pathogens during therapy. For instance, the possibility of quantification of bacteria through salivary DNA (Table 4.1) without cultivation of samples, which is laborious and lengthy, represents a useful and practical diagnosis approach of infectious diseases. Nevertheless, salivary DNA is mainly applied in pharmacogenomic and epidemiologic studies and for forensics [16].
Table 4.1
Salivary DNA markers identified for several oral and systemic diseases
Pathophysiological condition
|
Type of sample
|
Methodology
|
Major findings
|
Refs.
|
---|---|---|---|---|
Dental caries
|
Unstimulated WS
|
DNA extraction kit + real-time PCR
|
Increased Streptococcus mutans genomic DNA
|
[37]
|
Oral squamous cell carcinoma (OSCC)
|
Unstimulated WS
|
DNA extraction kit + PCR + DNA sequencing
|
Mutation of p53 codon 63
|
[38]
|
Pityriasis rosea
|
Unstimulated WS
|
DNA extraction kit + nested PCR
|
Presence of HHV-6 and HHV-7 viral DNA
|
[39]
|
Head and neck squamous cell carcinoma (HNSCC)
|
Unstimulated WS
|
Cloned p53 sequences amplified by PCR + radiolabeled oligonucleotide probes
|
Mutations in p53 gene; promoter hypermethylation patterns: p16; MGMT; DAP-K; increased mtDNA content
|
|
DNA extraction kit + PCR
|
||||
Real-time qPCR
|
||||
Pancreatic cancer
|
Unstimulated WS
|
DNA extraction kit + microarray + qPCR
|
Atopobium parvulum; Granulicatella adiacens; Neisseria elongata; Prevotella nigrescens; Streptococcus australis; Streptococcus mitis bacterial DNA specific variation
|
[13]
|
The search for genetic determinants underlying common diseases such as cardiovascular diseases, cancer, osteoporosis, and diabetes can also be revolutionized through large population-based epidemiologic studies utilizing salivary DNA instead of blood DNA [43]. Salivary DNA markers have become appealing since the presence of tumor markers in saliva has been reported (Table 4.1), such as aberrant methylation of tumor suppressor genes in cancer cells [31].
Biomedical Applications of Salivary Transcriptomics
Alongside DNA, RNA can also be found in saliva because salivary RNAs are protected by specific mechanisms against the saliva nucleases including association with macromolecules (such as mucins and salivary chaperone Hsp70) and exosomes [20, 44, 45]. Even more interestingly, saliva contains not only transcripts of genes related to immune defense, digestion, and electrolyte and water metabolism as expected but also transcripts of most metabolic processes in the body [26]. Therefore, the transcriptomic signature holds valuable information to discover novel biomarkers for oral and systemic diseases. In 2004, the human salivary transcriptome in CFS was discovered by applying microarray technology [26].
It was discovered that RNA in saliva exists from partial to full-length forms and it is of vital importance to understand the characteristics of salivary RNA network in order to use RNA markers in clinical diagnosis and surveillance. Furthermore, different types of RNA coexist in saliva leading to the saliva RNA interactome concept. They all have implicated roles in gene regulation, and therefore, a complete characterization of mRNAs, microRNAs (miRNAs), small nucleolar RNAs (snoRNAs), and other RNA species found in saliva should be made in order to understand their impact and interplay in biological and pathological processes [16, 26]. The salivary mRNAs are of extreme importance because viral mRNA can be detected in saliva, which indicates viral infections [46]. miRNAs have gained increased attention in the salivary diagnostics field due to their roles in cell growth, differentiation, apoptosis, host-pathogen interactions, stress responses, and immune function. These short RNA transcripts are differentially expressed in several cancer cell types compared with normal cells and may accurately distinguish poorly differentiated tumors [31]. Consequently, miRNA cancer biomarkers are potentially very powerful in cancer diagnoses using salivary tests. The utility of salivary RNAs in biomedical applications is being explored, and candidate markers for several diseases have been found (Table 4.2).
Table 4.2
Salivary RNA markers identified for several oral and systemic diseases
Pathophysiological condition
|
Type of sample
|
Methodology
|
Major findings
|
Refs.
|
---|---|---|---|---|
Breast cancer
|
Unstimulated CFS
|
RNA extraction and amplification kits + microarray + RT-qPCR
|
Upregulated: CSTA; TPT1; IGF2BP1; GRM1; GRIK1; H6PD; MDM4; S100A8
|
[47]
|
Esophageal cancer
|
Unstimulated CFS
|
RNA extraction and amplification kits + microarray + RT-qPCR
|
Upregulated: miRNA-10b; miRNA-144; miRNA-21; miRNA-451
|
[48]
|
Malignant parotid gland tumors
|
Unstimulated CFS
|
RNA extraction and amplification kits + microarray + RT-qPCR
|
Upregulated: hsa-miRNA-132; hsa-miRNA-15b; mmu-miRNA-140; hsa-miRNA-223
|
[49]
|
Oral squamous cell carcinoma (OSCC)
|
Unstimulated CFS
|
RNA extraction and amplification kits + microarray + qPCR
|
Upregulated: DUSP1; H3F3A; IL1B; IL8; OAZ1, S100P; SAT
|
[50]
|
Resective pancreatic cancer
|
Unstimulated CFS
|
RNA extraction and amplification kits + microarray + qPCR
|
Upregulated: KRAS, MBD3L2, ACRV1
|
[51]
|
Downregulated: DPM1
|
||||
Sjögren’s syndrome (SS)
|
Stimulated CFS
|
RNA extraction and amplification kits + microarray + real-time qPCR
|
Upregulated: G1P2; proteasome subunit β(beta) type 9; guanylate-binding protein 2, IFN-induced protein 44; β(beta)2-microglobulin
|
[52]
|
Sleep deprivation
|
Unstimulated CFS
|
RNA extraction and amplification kits + microarray + qPCR
|
Upregulated: salivary amylase
|
[53]
|
In the near future, focus should be given to specific salivary RNA types, such as miRNAs and snoRNAs, to achieve more specificity and sensitivity in clinical diagnostics and to ensure a complete profile of the transcriptomic signature in certain pathophysiological conditions. For instance, salivary miRNAs found in exosomes seem to hold great interest as biomarkers for autoimmune disorders in order to reduce the probability of false-negative results [54].
The future of salivary genomics and transcriptomics relies not only in the optimization and standardization of methodological protocols but also in the enlargement of studied populations in cross-sectional longitudinal studies to validate and translate DNA and RNA markers for routine clinical analysis.
Salivary Proteomics
The term “proteomics” concerns the study of the entire protein asset of a specific biofluid, cell type, or tissue. It represents a tool for a better understanding of protein function and how it interacts with other proteins, envisioning the assessment of health or disease states [55]. Saliva proteome holds several essential roles, such as digestive processes, antimicrobial activity, ion storage, microorganism aggregation, tooth surface protection, lubrication, and buffering actions [56]. A life cycle of a salivary protein comprises the basic cellular process of protein biosynthesis and posttranslational modifications—PTMs (comprehending glycosylation, phosphorylation, proteolysis, and sulfation)—prior to and after secretion of the protein into the oral cavity. Therefore, the salivary proteome is highly dynamic and variable depending on time, nature, and number of agents capable of inducing protein modifications [57].
Proteomic studies allow the analysis of salivary composition, investigating the major families of salivary proteins, the different contributions of salivary glands, and the physiological and pathological modifications of saliva. Currently, the salivary proteome is essentially characterized with more than 3,000 different proteins already identified [55]. The major salivary protein families involve salivary proline-rich proteins (PRPs), histatins, statherin, cystatins, and α(alpha)-amylases. Whereas these protein classes can be considered specific of saliva, there are many other proteins present in whole saliva that are common to other organs and biofluids such as mucins; lysozyme; lactoferrin; agglutinin; peroxidase; carbonic anhydrase; serum proteins like salivary IgA, IgG, and albumin; proteins of the S100 class and other calcium-binding proteins; defensins; and thymosin β(beta)4 [57, 58]. Remarkably, approximately 20 % of total salivary proteins are also seen in plasma and show comparable functional diversity and disease linkage [59]. This fact abets the potential of salivary proteomics in the diagnosis and monitoring not only of oral diseases but also of systemic conditions.
Salivary proteomics aims to discriminate between healthy and pathological states through the identification of proteins that are uniquely correlated to a specific state [60]. The understanding of each protein’s role in the oral cavity will contribute to improving the scientific knowledge of several oral and systemic diseases’ pathogenesis.
Methodologies Used in Salivary Proteomics
The application of salivary proteins in the biomedical field as disease markers has only been possible due to the combination of conceptual innovations and technical breakthroughs in separation techniques, mass spectrometry, and bioinformatics for data analysis and integration. Proteomic approaches can be divided into four main classes: structural, expression, functional, and targeted proteomics [61]. All these types of proteomics can be applied to saliva in order to find patterns of salivary proteins that can be used for diagnosis, prognosis, monitoring, and therapeutic targets of human diseases. On one hand, the structural characterization (structural proteomics) of proteins and their interactions and assemblies may be important for drug targets [62]. On the other hand, the quantification (expression proteomics) and functional characterization (functional proteomics) of salivary proteins can assist in a specific and reliable diagnosis. Targeted proteomics is a more recent approach and focuses on the study of only one or a preselected group of proteins in order to evaluate its importance in a certain physiological or pathological event. Regarding salivary proteomics, all these approaches can be employed to enhance the knowledge of disease pathogenesis and to find salivary biomarkers.
Overall, proteome characterization is achieved through different tools classified in top-down or bottom-up proteomics. In top-down proteomics approach, proteins are analyzed without proteolytic digestion. Hence, intact proteins are introduced into the mass spectrometer (MS), and after ionization the ions generated are subjected to separation, fragmentation, and automated interpretation of MS data, yielding both the molecular weight of the intact protein and the protein fragmentation ladders [63]. In contrast, the bottom-up proteomics approach involves enzymatic and/or chemical cleavage of protein-originating peptides. The peptide analysis requires chromatographic or electrophoretic strategies to decrease the complexity of peptide mixtures before MS analysis [64]. In order to characterize the salivary proteome, both approaches have been widely applied to saliva, contributing toward demystifying its complexity.
The salivary proteomics workflow is divided in sample preparation, protein separation, characterization, and functional validation. The first step in the salivary proteome characterization is sample preparation that encompasses all the experimental steps performed to obtain a protein extract. Secondly, the separation of proteins is required, and the major approaches utilized can be divided into gel-based approaches (e.g., 2-dimensional gel electrophoresis (2-DE)) and gel-free-based approaches (e.g., liquid chromatography). After the separation step, the proteins are analyzed by mass spectrometry, commonly matrix-assisted laser desorption/ionization time-of-flight with tandem mass spectrometry (MALDI-TOF-MS/MS) or electrospray ionization with tandem mass spectrometry (ESI-MS/MS) [55]. The main strategies for protein identification by MS comprise database searching, de novo sequencing, and peptide sequence tag [65]. Database searching with MASCOT [66] and SEQUEST [67] is the most popular. Protein quantification in gel-free-based approaches is based on labeling methods, the most common being the isobaric tagging for relative and absolute quantitation (iTRAQs) or label-free methods based on the comparison of related peak areas between MS runs [68, 69]. The last step of a proteomic workflow stands in functional validation through standard methods as western blot and enzyme-linked immunosorbent assay (ELISA). With the plethora of technologies available for proteomic studies, the choice of the methodological strategy is based on sample amount and on the goal of the study. If the aim is the characterization of salivary glycoproteome or phosphoproteome in a certain pathological condition, such as periodontitis, enrichment strategies should be employed.
Biomedical Applications of Salivary Proteomics
The comprehensive analysis of human saliva proteome, through the application of the different methodologies described previously, will contribute to the understanding of disease pathogenesis and might provide a foundation for the recognition of potential disease biomarkers. Indeed, clinical proteomics tries to highlight the relationships between proteins and defined physiological conditions connecting these biomolecules to disease diagnosis, monitoring of therapies, therapeutic outcomes, and disease progression [59]. Indeed, several protein candidate markers have been described in several oral and systemic diseases as dental caries, periodontitis, Sjögren’s syndrome (SS), oral squamous cell carcinoma (OSCC), head and neck squamous cell carcinoma (HNSCC), oral lichen planus (OLP), autism spectrum disorders, diabetes, breast cancer, and lung cancer (Table 4.3).
Table 4.3
Salivary protein markers identified for several oral and systemic diseases
Pathophysiological condition
|
Type of sample
|
Methodology
|
Major findings
|
Refs.
|
---|---|---|---|---|
Aggressive periodontitis
|
Unstimulated WS
|
2DE-ESI-MS/MS
|
Increased: albumin; Ig γ(gamma)2 chain C region; Ig α(alpha)2 chain C region; vitamin D-binding protein; amylase; zinc α(alpha)2 glycoprotein
|
[70]
|
Decreased: lactotransferrin; elongation factor 2; 14-3-3 sigma; SPLUNC-2; carbonic anhydrase VI
|
||||
Autism spectrum disorders
|
Unstimulated WS
|
RP-HPLC-ESI-MS
|
Hypophosphorylation: histatin 1; statherin; entire and truncated isoforms of aPRPs
|
[71]
|
Breast cancer
|
Unstimulated WS
|
Enzyme-linked immunosorbent assay
|
Increased: c-erbB-2; carbonic anhydrase VI
|
|
2DE-MALDI-MS/MS
|
||||
Chronic periodontitis
|
Unstimulated WS
|
2DE-MALDI-MS/MS and LC-ESI-MS/MS
|
Increased: albumin; hemoglobin; Ig proteolysis
|
[73]
|
Decreased: cystatin
|
||||
Cystic fibrosis
|
Unstimulated WS
|
RIA and RRA assays
|
Present a less efficient form of salivary EGF
|
[74]
|
Dental caries
|
Unstimulated WS
|
2DE-MALDI-MS/MS
|
Increased: amylase, Ig A, lactoferrin
|
|
IEF-SDS-PAGE-MALDI-TOF/TOF
|
Decreased: lipocalin, cystatins S; cystatins SN; statherin; truncated cystatin S
|
|||
Gingivitis
|
Unstimulated WS
|
2DE-MALDI-MS/MS and LC-ESI-MS/MS
|
Increased: albumin; amylase
|
[77]
|
Head and neck squamous cell carcinoma (HNSCC)
|
Unstimulated WS
|
SDS-PAGE-MALDI-MS/MS
|
Increased: annexin A1; β(beta)- and γ(gamma)-actin; cytokeratins 4 and 13; zinc finger proteins; P53 pathway proteins; β(beta)-fibrin; S100A9; transferrin; Ig heavy chain constant region γ(gamma); cofilin-1; α(alpha)-1B-glycoprotein; complement factor B
|
|
Stimulated WS
|
2DE-MALDI-TOF-MS
|
Decreased: cystatin S; parotid secretory protein; poly-4-hydrolase β(beta)-subunit
|
||
SDS-PAGE-LC-MS/MS
|
||||
Lung cancer
|
Unstimulated WS
|
2DE-(DIGE)-MALDI-MS/MS
|
Increased: annexin A1; HBA2; cystatins D and S; zinc α(alpha)2 glycoprotein; human calprotectin
|
[81]
|
Decreased: carbonic anhydrase VI; lipocalin; S100A9; S100A8
|
||||
Oral lichen planus (OLP)
|
Unstimulated WS
|
2DE-MALDI-TOF-MS
|
Increased: urinary prekallikrein
|
[82]
|
Decreased: PLUNC
|
||||
Oral squamous cell carcinoma (OSCC)
|
Unstimulated WS
|
2DE-MALDI-TOF-MS
|
Increased: transferrin; truncated cystatin SA-1; S100A9, profiling, CD59, catalase; M2BP; soluble CD44; IL-8; IL-8; IL-1β
|
|
Stimulated WS
|
SELDI
|
|||
RP-LC-MS/MS
|
||||
ELISA (sandwich type)
|
||||
Multiplex bead-based flow cytometric assay
|
||||
Real-time qPCR + ELISA
|
||||
Periodontitis
|
Unstimulated WS
|
2DE-MALDI-MS/MS
|
Increased: S100A8; S100A9; S100A6; albumin; Ig γ(gamma)2 chain C region; Ig α(alpha)2 chain C region; vitamin D-binding protein; amylase; zinc α(alpha)2 glycoprotein; MMP-8; IL-1β(beta)
Decreased: lactotransferrin, elongation factor 2, 14-3-3 sigma, SPLUNC-2; carbonic anhydrase VI
|
|
2DE-ESI-MS/MS
|
||||
Enzyme-linked immunosorbent assay
|
||||
Rheumatoid arthritis
|
Unstimulated WS
|
2DE-MALDI-MS/MS
|
Increased: GRP78/BiP
|
[91]
|
Sjögren’s syndrome (SS)
|
Unstimulated WS
|
2DE-MALDI-TOF-MS
|
Increased: β(beta)2-microglobulin; lactoferrin; Ig kappa light chain; polymeric Ig receptor; lysozyme C; cystatin C; α(alpha)-enolase; E-FABP; amylase; α(alpha)-enolase; actin; carbonic anhydrases I and II
|
|
SELDI + 2DE-MALDI-TOF-MS
|
Decreased: carbonic anhydrase VI; amylase precursor; carbonic anhydrase VI; G3PDH; SPLUNC-2; cystatins (S, SA, C, SN, D); carbonic anhydrase VI
|
|||
Parotid saliva
|
2DE-LC-ESI-MS/MS
|
|||
Unstimulated WS
|
||||
Type 1 diabetes
|
Unstimulated WS
|
RP-HPLC-ESI-MS
|
Increased: α(alpha)-defensins 1, 2, and 4; S100A9
|
[95]
|
Decreased: statherin, histatins 1 and 5
|
||||
Type 2 diabetes
|
Unstimulated WS
|
2DE-LC-MS/MS
|
Increased: A1AT, cystatin C, α(alpha)2-macroglobulin; transthyretin
|
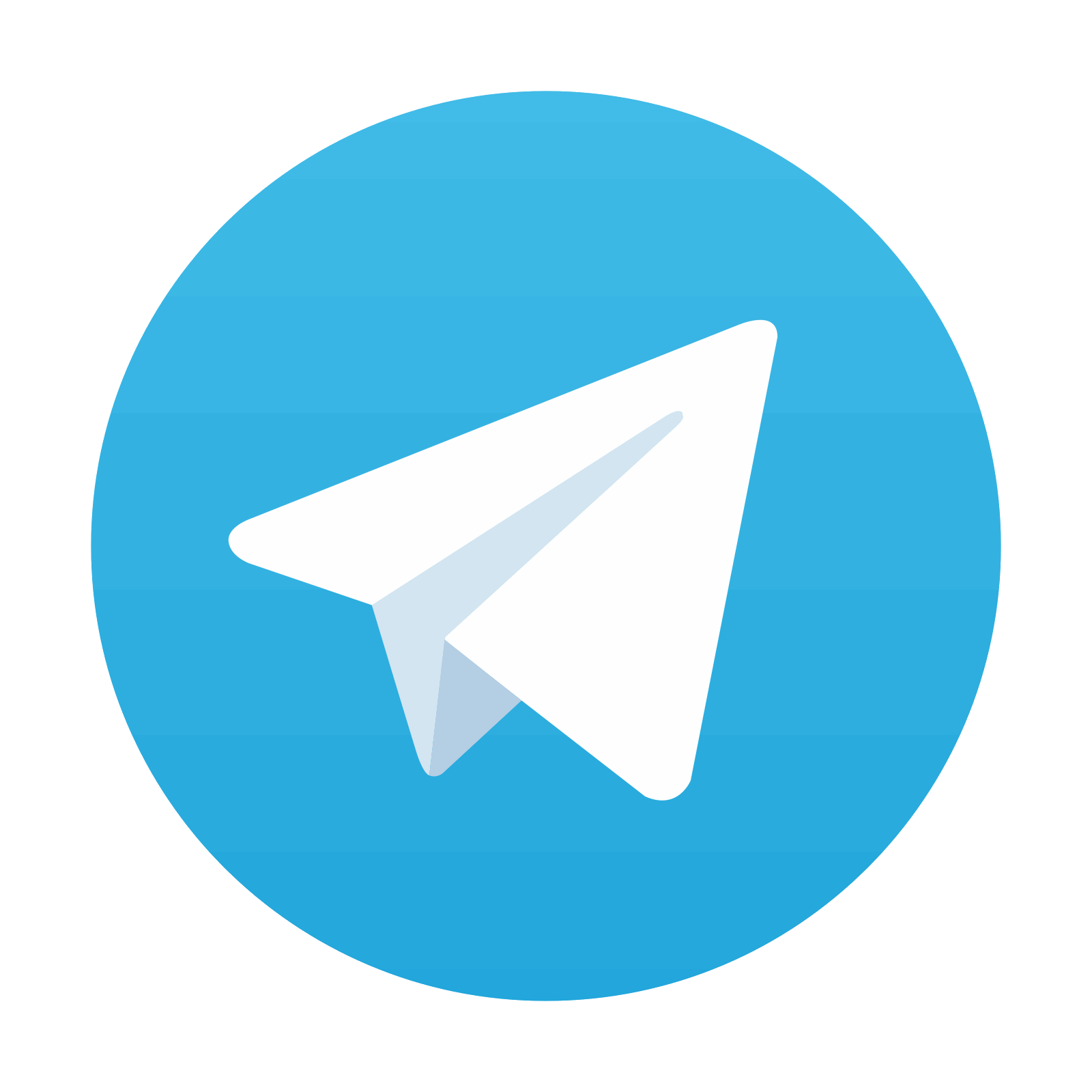
Stay updated, free dental videos. Join our Telegram channel

VIDEdental - Online dental courses
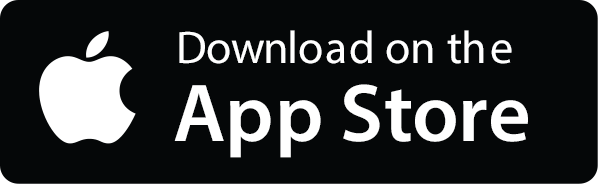
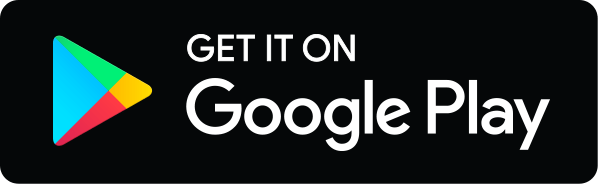