Highlights
- •
At elevated temperatures viscosity of highly viscous resin composites is reduced.
- •
Thermo-viscous material shows low viscosity with acceptable stickiness at elevated temperatures.
- •
Measuring unplugging work enables determination of stickiness.
- •
Stickiness increases at higher temperatures for conventional resin composites.
Abstract
Objective
To investigate the influence of pre-heating different classes of dental resin composites on viscosity and stickiness at five different temperatures.
Methods
Six flowable, five conventional packable, and one thermo-viscous bulk-fill resin composites were heated up to 54 °C in a plate-plate rheometer to determine their complex viscosity. Normal force measurements were carried out for the six packable materials to determine the unplugging force and unplugging work (stickiness) over the same temperature range. Data were analyzed using Kolmogorov–Smirnoff test, one-way ANOVA and Tukey Post Hoc test with α = 0.05 as level of significance.
Results
At 23 °C packable composites showed viscosity between 6.75 and 19.14 kPa s, while flowable composites presented significantly lower viscosities between 1.31 and 2.20 kPa s. Pre-heating led to a drop of 30–82% in the viscosity of packable materials. The thermo-viscous material dropped to the level of flowables at 45 and 54 °C thus behaving as a packable composite at room temperature with flowable-like viscosity at higher temperatures. No statistically significant differences for viscosity were observed among flowable composites at any temperature.
The unplugging force decreased for packable composites, while their unplugging work generally increased at elevated temperature. At 23 °C unplugging force was measured between 7.50 and 19.18 N, while pre-heating up to 54 °C led to values between 2.9 and 6.2 N. Regarding unplugging work at 23 °C the calculated values were between 3.0 and 8.9 × 10 −3 J and at 54 °C between 8.8 and 13.0 × 10 −3 J.
Significance
Pre-heating significantly reduced viscosity of highly viscous resin composites, while no influence was shown for flowable composites. In general stickiness, measured as unplugging work, increased at elevated temperatures. The thermo-viscous material showed low viscosity comparable to flowable composites at 45 and 54 °C, yet its stickiness did not increase significantly compared to the values at 23 °C.
1
Introduction
Depending on the shape of the cavity for direct resin composite filling therapy, it can be challenging to manage a complete sealing of the tooth substrate and to create an interface to the resin composite without gap formation . Secondary carries is highly associated with the restoration’s margins and possibly connected with the gaps between tooth structure and restoration , termed microleakage . To improve adaptation, flowable composites can be used as cavity liners . Low viscosity materials are able to increase the wetting effect to the cavity wall, improving marginal adaptation and reducing microleakage . Viscosity is highly dependent on the composition of the resin matrix, filler fraction, filler size distribution and silane treatment of the fillers, as well as the interaction between fillers and the matrix . However, those differences in composition are also reflected by inferior material properties as flowables are known to exhibit reduced flexural strength or elastic modulus compared with their packable counterparts .
A practical approach to improve adaptation is to lower the viscosity of packable resin composites during application without compromising their mechanical properties. Resin composites behave as non-Newtonian viscoelastic fluids, showing shear thinning behavior . As viscosity decreases under shear loading, resin composites become more fluid during adaptation to the tooth structure . A significant reduction of viscosity can also be achieved by pre-heating packable composites before application . At elevated temperatures, thermal vibrations force monomers of the matrix further apart and allow them to slide by each other more easily which lowers the internal friction . The influence of temperature on viscosity is explained by the Arrhenius equation ( η = A * e − E/RT ; η : temperature dependent complex viscosity; A : proportional coefficient; E : activation energy for flow; R : gas coefficient; T : temperature), which describes the correlation between fluid viscosity and temperature . Plotting the logarithm of η against the reciprocal temperature (1/ T ) results in a linear behavior for materials that follow the Arrhenius law .
A new thermo-viscous bulk fill material has been developed accordingly, which allows pre-heating up to 65 °C (dispenser) or even to 68 °C (caps warmer) before application . New monomer chemistries that may require heat resistant initiator systems become a challenge in this type of material . Whether a thermo-viscous resin composite can reach viscosity levels compared to that of flowable materials yet managing to maintain clinical acceptable handling properties in its pre-heated state, has not been fully elucidated in previous studies.
Stickiness, in context of a clinical scenario, is the ability of a resin based restorative or luting composite to adhere and stick to the surface of a dental placement instrument. For a dentist, this is a critical handling property, which may cause the ‘pull out’ of material from a cavity, in turn affecting form, shape and marginal integrity of restorations . Composites should not stick to the plunger while filling the cavity, because porosities and voids are more likely to be formed . A relation has been found between open margins and highly sticky materials . On the molecular level, the adhesion between a placement instrument and the restorative material is established via comparably weak dipole (van der Walls) interactions . Furthermore, wettability and unplugging force or work are expected to correlate with paste viscosity, in terms of reduced wetting at higher viscosities and reduced resistance to detachment. This is supported by the fact that interfacial interactions between fillers and monomers have an impact on viscoelastic properties of resin composites and in turn on their stickiness . Identifying the maximum unplugging force at detachment or the unplugging work (defined as the integrated area of force and displacement), are measurements previously employed to determine stickiness in the laboratory . Different settings were designed to provide reproducible results. Some studies lowered a flat-ended stainless steel probe or instrument into a resin composite portion , others noted individual qualitative assessment by dental practitioners that give a scale of composite stickiness as a description of handling behavior . To our knowledge, there is no previous work that has assessed the stickiness of dental resin composites with the use of a plate-plate rheometer. Reducing resin composite viscosity by pre-heating may improve wettability and result in higher energy for separation from the instrument . Thereby, changes in temperature have an influence on unplugging force, unplugging work reveals variations in molecular linkages that relate to the shear behavior .
Consequently, the aim of this study was to investigate the influence of temperature on the viscosity and stickiness of uncured packable and flowable resin composites, and how they compare to a new thermo-viscous bulk fill composite. We hypothesize that pre-heating is reducing viscosity of packable and flowable resin composites, and that a temperature increase will negatively affect stickiness of packable resin composites (unplugging force and unplugging work).
2
Materials and methods
The influence of pre-heating on viscosity and stickiness was investigated for six flowable, five packable, and one thermo-viscous bulk fill resin composites. Material composition, specifications and abbreviations are listed in Table 1 . Viscosity measurements were performed for all materials while stickiness was tested only for the packable and the thermo-viscous materials.
Material (code) | Manufacturer | Classification | Batch-nr. | Matrix | Fillers |
---|---|---|---|---|---|
CLEARFIL MAJESTY™ Flow (CMF) | Kuraray Noritake | Posterior region nano-hybrid flowable | B20052 | TEGDMA Hydrophobic aromatic dimethacrylate |
81 wt%/62 vol% -silanated barium glass fillers (average 3 μm) -silanated colloidal silica (average 20 nm) |
CLEARFIL MAJESTY™ Posterior (CMP) | Kuraray Noritake | Posterior region nano-hybrid packable | 7L0064 | BIS-GMA TEGDMA Hydrophobic aromatic dimethacrylate |
92 wt%/82 vol% -silanated glass ceramic fillers (average 1.5 μm) -surface treated alumina micro fillers (average 20 nm) -silanated silica filler |
Ecosite Elements HIGHLIGHTS (EEH) | DMG | Universal nano-hybrid flowable | 803736 | EBPADMA BIS-GMA TEDMA |
65 wt%/38 vol%(0.02 –0.7 μm) -Ba glass |
Ecosite Elements PURE (EEP) | DMG | Universal nano-hybrid packable | 803725 | EBPADMA BIS-GMA |
81 wt%/65 vol%(0.02 –0.7 μm) -Ba glass |
Filtek™ Supreme XTE (FSX) | 3M™ | Universal nano-hybrid packable | NA01835 | BIS-GMA UDMA TEGDMA BIS-EMA PEGDMA |
78.5 wt%/63.3 vol% -silica fillers (20 nm) -zirconia fillers (4–11 nm) -zirconia-silica-cluster (0.6–20 μm) |
Filtek™ Supreme XTE Flowable (FSF) | 3M™ | Universal nano-hybrid flowable | N998213 | BIS-EMA BIS-GMA EBADMA |
65 wt%/55 vol% silica-fillers <5% YbF 3 |
Grandio®SO (GSO) | VOCO | Universal nano-hybrid packable | 1851244 | BIS-GMA TEGDMA BIS-EMA |
89 wt%/73 vol% inorganic fillers -glass ceramic fillers (average particle size 1 μm) -functionalized siliciumdioxide nano particles (size 20–40 nm) |
Grandio®SO Flow (GSF) | VOCO | Universal nano-hybrid flowable | 1912388 | HEDMA BIS-GMA TEGDMA BIS-EMA |
81 wt%/65 vol% inorganic fillers -glass ceramic fillers (average particle size 1 μm) -functionalized siliciumdioxide nano particles (size 20–40 nm) |
Grandio®SO Light Flow (GSL) | VOCO | Nano-hybrid highly flowable | V76859 | BIS-GMA BIS-EMA TEGDMA Aliphatic dimethacrylate 1,6-Hexa-nediylbis-methacrylate |
76% wt inorganic fillers |
Venus® Diamond Flow (VDF) | Kulzer | Universal nano-hybrid flowable | K010213 | UDMA EBADMA TEGDMA |
65 wt%/41 vol% (0.02–5 μm) -Ba-Al-F silicate glass -10–25% YbF 3 -SiO 2 |
Venus® Pearl (VPE) | Kulzer | Universal nano-hybrid packable | K010054 | TCD-Urethane-acrylate UDMA TEGDMA Polymer |
59 vol% (particle size 5 nm-5 μm) -Barium aluminum boro fluor silicate glass -Silica |
VisCalor® bulk (VCB) | VOCO | Thermo-viscous bulk fill | V76291 | BIS-GMA Aliphatic dimethacrylate |
83 wt% inorganic fillers |
2.1
Viscosity
Viscosity is defined as the extension of internal friction in a fluid and is measured as force per unit area resisting uniform flow. Resin composites are non-Newtonian fluids showing viscoelastic behavior. This can be characterized by oscillating shear deformation tests in a rheometer. Thereby the contribution of the elastic part is described by the storage modulus G ′, that of the viscous part by the loss modulus G ″. tan δ is the ratio of loss to the storage modulus. The complex shear modulus ( G* ) uses the imaginary loss modulus ( iG ″) and is given by Eq. (1) :
G*=G′+iG″
The complex viscosity ( η *) is calculated (Eq. (2) ) upon the ratio of the complex shear modulus and the frequency of oscillation ( ω ):
η*=G*/ω
An empirical relation (Cox-Merz) often relates both types of viscosity . According to Cox-Merz, steady-state shear and complex viscosities are equal when the shear strain applied on the polymer is equal to the frequency of oscillation ( ω ) employed for testing the same polymer through oscillatory tests.
Viscosity measurements on the resin composites were performed using a rheometer (Physica MCR 301, Anton Paar, Graz, Austria) equipped with an aluminum plate-plate configuration in a temperature-controlled test chamber (Peltier, H-PTD200). The rheological properties of a viscoelastic material are independent of strain up to a critical strain level (linear viscoelastic region). A preliminary test was performed in order to measure the limit of the linear viscoelastic region (LVE region) and to choose the right range of amplitude for the next tests, without destroying the structure of the samples. A strain rate of 0.1–100% and a frequency of 1 Hz were used at five different temperatures (23, 30, 37, 45 and 54 °C) for each material (dynamic strain sweep). The results appear as a diagram with strain plotted on the x -axis and storage modulus G ′ and loss modulus G ″ plotted on the y -axis (Controlled Shear Deformation Graph). LVE region is calculated by analysis software.
Complex viscosity of all twelve resin composites (packables and flowables) were tested in oscillation using a parallel plate geometry with a 25 mm radius. The gap between the plates was set to 0.25 mm for all materials. Any excess of resin composite material or air bubbles arising around the circumference of the plate were manually removed prior to initiating the measurements. Testing was performed ( n = 10 per material) at five different temperatures (23, 30, 37, 45 and 54 °C).
2.2
Stickiness
Stickiness can be quantified by vertical detachment tests determining unplugging force or unplugging work. Testing was performed for the six packable materials with the same rheometer as used for viscosity measurements, but adjusted as follows: The upper 8 mm aluminum plate was covered with a stainless-steel surface and polished up to 4000 grit (0.1 μm roughness) to imitate the stainless-steel instruments dentists use in clinical practice. The rheometer was equipped with a load cell (50 N; accuracy ± 0.03 N) for measurement of normal forces to the plate.
The resin composite samples were placed in a gap of 1 mm between the plates which was automatically adjusted prior to each measurement to ensure applied force was zero at the beginning. Any excess material was removed before testing. The tensile loading rate for the pull-off test was 5 mm/s, which fits within the range used in previous studies . The unplugging force was measured until the upper plate and the resin composite were detached and the maximum value was recorded. As flowable materials would stick to both upper and lower plates, no separation force could be measured, and the reason why only the packable materials were tested in stickiness measurements. Based on the force-displacement graph, the work needed for separation (unplugging work) was calculated from the area under the curve. In case the resin composite was separated from the bottom plate and moved upwards with the upper plate, the measurement was considered invalid. All tests were performed for each temperature (23, 30, 37, 45, 54 °C) and material ( n = 10 per material).
2.3
Statistical analysis
Data were analyzed using statistical software (SPSS Statistics 21, IBM, Chicago, USA). Kolmogorov–Smirnov test was applied to check for a normal, Gaussian distribution. One-Way ANOVA and Student–Newman–Keuls Post Hoc test served to analyze the statistical differences at a level of significance of α = 0.05.
3
Results
3.1
Complex viscosity
Mean values for complex viscosity for each material at five different temperatures (23, 30, 37, 45, 54 °C) with standard deviations are shown in Table 2 and depicted in Fig. 1 . The results for complex viscosity were evaluated for following Arrhenius’ law. This can be valued by plotting the logarithm of the complex viscosity η against the reciprocal temperature (1/ T ). Fig. 1 shows, that all materials showed a mostly linear behavior over the tested temperatures in the graph. Activation energies “ E ”, calculated following Arrhenius’ equation ( η = A * e − E / RT , η : temperature dependent complex viscosity; A : proportional coefficient; R : gas coefficient; T : temperature) according to the trend lines’ gradients are shown in Table 2 .
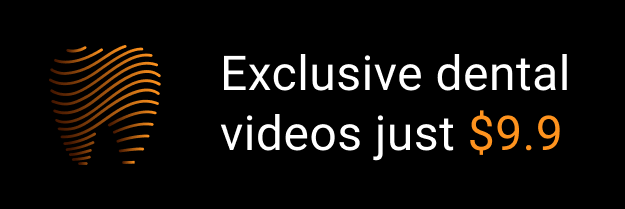