Abstract
Objective
The angiogenic differentiation of dental pulp stem cells (DPSCs) is important for tissue homeostasis and wound healing. In this study the influence of 2-hydroxyethyl methacrylate (HEMA) on angiogenic differentiation was investigated.
Methods
To evaluate HEMA effects on angiogenic differentiation, DPSCs were cultivated in angiogenic differentiation medium (ADM) in the presence or absence of non-toxic HEMA concentrations (0.1 mM and 0.5 mM). Subsequently, angiogenic differentiation was analyzed on the molecular level by qRT-PCR and protein profiler analyzes of angiogenic markers and flow cytometry of PECAM1. The influence of HEMA on angiogenic phenotypes was analyzed by cell migration and sprouting assays.
Results
Treatment with 0.5 mM HEMA during differentiation can lead to a slight reduction of angiogenic markers on mRNA level. HEMA also seems to slightly reduce the quantity of angiogenic cytokines (not significant). However, these HEMA concentrations have no detectable influence on cell migration, the abundance of PECAM1 and the formation of capillaries. Higher concentrations caused primary cytotoxic effects in angiogenic differentiation experiments conducted for longer periods than 72 h.
Significance
Non-cytotoxic HEMA concentrations seem to have a minor impact on the expression of angiogenic markers, essentially on the mRNA level, without affecting the angiogenic differentiation process itself on a detectable level.
1
Introduction
The pulp is the origin of dental pulp stroma/stem cells (DPSCs). These cells have mesenchymal stem cell properties. They are able to self-renewal and have the capability to differentiate in multiple lineages, like muscle, bone, neuronal and endothelial cells [ , ]. DPSCs also have a high proliferation rate and can produce colony-forming units [ ]. They play an important role in tissue homeostasis and wound healing [ ]. One important process for wound healing is angiogenesis [ ]. Angiogenesis means the formation of new blood vessels from existing blood vessels and is primarily carried out by endothelial cells. Dental stem cells support or initiate this process by secreting angiogenic factors [ ] or differentiating into endothelial cells [ ]. Dental composite materials could interfere with differentiation and thus wound healing. The monomer 2-hydroxyethyl methacrylate (HEMA) is widely used in dental composite materials and accounts for 35–50% of most adhesion promoters [ ] and is the most eluted monomer of primers and adhesives [ ]. It reduces the viscosity and increases the bond strength of bonding agents [ , ]. Approximately 33–75% of the methacrylate monomers are not incorporated into the polymer network [ , ]. Enzymes in the oral cavity also degrade the polymer [ ]. Due to its hydrophilicity and low molecular weight, free HEMA can diffuse into the pulp and cause adverse effects [ , ]. Local HEMA concentrations in the millimolar range may occur in the environment of dental resins (determined by in vitro studies) [ ]. A study from Kaga et al. showed that 1.5–2% of co-monomers elute from polymerized adhesives [ ]. Çetingüç et al., however, assumes that no toxic HEMA concentrations occur when used as dentin bonding agent [ ]. However, HEMA can be metabolized to more toxic epoxides, which can multiply the toxicity of HEMA [ ]. While HEMA-caused cytotoxicity has already been extensively investigated, studies on effects on angiogenic differentiation pathways, which could also occur at sub-toxic concentrations, are lacking. it has been shown that HEMA has an inhibitory effect on osteogenic differentiation of DPSCs [ ] and that the composite monomer TEGDMA can interfere with angiogenic differentiation of dental pulp stem cells [ ].
One of the most important angiogenic signaling molecules is vascular endothelial growth factor (VEGF). VEGF increases the formation of new capillaries and its expression is linked to factors such as hypoxia and shear stress. Another important angiogenesis marker is platelet endothelial cell adhesion molecule (PECAM1), which is located on endothelial cells and important for the cell junction integrity [ ]. It was shown that blocking of PECAM1 by anti-PECAM1 antibodies completely inhibits in vitro tube formation [ ]. In vitro tube formation is a very specific test for angiogenesis. All endothelial cells are able to form tubules in vitro [ ].
The aim of this study was to investigate the effect of sub-toxic HEMA concentrations on the angiogenic differentiation of DPSCs in vitro. For this purpose, the relative mRNA levels of VEGF, the corresponding receptors VEGFR-1 and -2, and PECAM1 (CD31) were determined by qRT-PCR. Various angiogenic marker proteins were analyzed using protein antibody arrays and PECAM1 via flow cytometry. Additionally, the influence of HEMA on the cell migration and tube formation of DPSCs was analyzed by microscopy. MTT-assays were carried out initially to define non-cytotoxic concentrations of HEMA for DPSCs.
2
Materials and methods
2.1
Isolation and cultivation of dental pulp stem cells (DPSCs)
The studies conducted were approved by the Ethics Committee of Hannover Medical School (No. 1096) and cell donation was carried out with the signed consent of the donor. The human DPSCs were extracted from wisdom teeth of a 19-year-old female donor. The pulp was cut into small pieces and the cells were isolated by enzymatic digestion. For this purpose, 3 mg/mL collagenase type I (Gibco, Grand Island, NY, USA) and 4 mg/mL dispase II (Sigma-Aldrich, Steinheim, Germany) were used and the cells were cultivated for 1 h in α-minimal essential medium (α-MEM; Gibco) at 37 °C. The cells were then filtered through a 70 μm cell strainer (EASYstrainer, Greiner bio-one, Frickenhausen, Germany) and multiplied in complete culture medium (CCM) at 37 °C and 5% CO 2 . The culture medium contains 15% fetal bovine serum (Biochrom, Berlin, Germany), 100 U/mL penicillin, 100 μg/mL streptomycin (Biochrom), 2.5 μg/mL amphotericin B (Capricorn Scientific Ebsdorfergrund, Germany) and 100 μM Lascorbic acid phosphate (Sigma-Aldrich). Subsequently, DPSCs were characterized by flow cytometry [ ]. Cells of the third passage were used and the cell culture was regularly checked for mycoplasma contamination.
2.2
MTT cell viability assay
Cytotoxicity was evaluated by the methyl-thiazolyl-tetrazolium (MTT) test. The test is based on the measurement of NAD(P)H-dependent oxidoreductases, which correlates with cell viability. 1 × 10 4 cells per well were seeded into a 96-well plate and allowed to adhere for 24 h. The cells were then treated with HEMA in CCM (0.1–8 mM) for 24 h, 48 h and 72 h. HEMA (VOCO, Cuxhaven, Germany) stock solutions were prepared in absolute ethanol (Baker Griesheim, Germany). The stock solutions were always freshly diluted in culture medium for each experiment. The final concentration of ethanol was 0.25% (v/v) for each treatment. The cells treated with 0.25% ethanol were used as solvent controls (ADM + EtOH). After the treatments, the medium was discarded and 100 μL of a MTT solution consisting of 0.5 mg/mL MTT (Sigma-Aldrich, Steinheim, Germany) dissolved in medium was added to each well. The cells were then incubated for 2 h at 37 °C and 5% CO 2 . The precipitated insoluble formazan was then dissolved in 100 μL DMSO with continuous stirring for 30 min. The light absorption was measured at 550 nm in a microplate reader (Spectra Max 250, MWG Biotech, Ebersberg, Germany). The experiment was performed at least three times independently with six technical repetitions each.
2.3
Induction of angiogenic differentiation and treatment with HEMA
For angiogenic differentiation, 6-well plates were coated with collagen I. Coating was performed according to Schertl et al. [ ]. For the first 24 h the cells were cultivated in CCM and then treated with 0.1 or 0.5 mM HEMA or equivalent volume of solvent. After additional 24 h, differentiation was started in angiogenic differentiation medium (ADM, Supplementary Data 2) and cells were cultivated for the time periods indicated in the respective experiments (max. 28 d). The medium was changed every 2–3 d.
2.4
Quantitative reverse-transcription polymerase chain reaction (qRT-PCR)
The quantitative reverse-transcription polymerase chain reaction was performed according to Schertl et al. [ ]. After total RNA isolation (RNeasy Plant Mini Kit, Qiagen, Hilden, Germany), DNA digestion was performed. Reverse transcription was done with Quantitec Reverse Transcription Kit (Qiagen, Hilden, Germany) using 1 μg of isolated RNA. The transcription of the angiogenic markers platelet endothelial cell adhesion molecule 1 (PECAM1), vascular endothelial growth factor (VEGF) and VEGF receptor 1 and 2 (VEGFR) were analyzed (primer: Supplementary Data 1). Additionally, the housekeeping genes beta actin (ACTB), beta-2-microglobulin (B2M), glyceraldehyde-3-phosphate dehydrogenase (GAPDH), 18S ribosomal RNA (RRN18S), succinate dehydrogenase flavoprotein subunit (SDHA2) and tyrosine 3-monooxygenase / tryptophan 5-monooxygenase activation protein zeta (YWHAZ) were analyzed. The two most stable genes were identified by geNORM (Vandesompele et al., 2002) and used for normalization. Fold changes were calculated by efficiency adjusted delta delta-CT method according to Pfaffl [ ].
2.5
Spheroid sprouting assays
The ability for capillary formation was investigated in Matrigel™ (Corning, New York, USA). After cultivating cells in angiogenic differentiation medium (ADM) for 14 d, 1.2 × 10 5 cells were seeded in 24-well plates coated with a thin layer of Matrigel™ (289 μL per well) and cultivated for 21 h. Microscopic images were taken with an inverted microscope (Olympus IMT-2, Hamburg, Germany), equipped with a digital camera (Olympus SC100). Capillary formation was quantified by counting the connections between spheroids. Subsequently, the amounts of connected spheroids were clustered in groups as followed: 2–4 spheroids, 5–7, 8–10 and more then 11 (11+) connected spheroids.
2.6
Protein antibody array
Antibody arrays (Proteome Profiler Human Angiogenesis Array Kit; R&D Systems, USA) were performed according to the manufactures instructions. After 21 d cells were lysed with cold lysis buffer (1% Igepal (Sigma-Aldrich, Steinheim, Germany), 20 mM Tris−HCl, 137 mM NaCl, 10% Glycerol, 2 mM EDTA) for 30 min. 30 μg of cellular protein (determined by Pierce BCA Protein Assay) were used for the assay. Secondary antibody was conjugated to horseradish peroxidase (HRP). Spots were detected by chemiluminescence spectrometry using the Fusion SL device (Vilber Lourmat, Germany). Data were analyzed by ImageLab analysis software (BioRad, USA). Background was subtracted and signals were normalized to reference spots.
2.7
Flow cytometric analysis of PECAM1
Cells were incubated in ADM for 20 days and then harvested with Accutase (Capricorn, Germany). Cells were washed, counted and aliquoted to one million cells per vial. The cells were centrifuged for 5 min (300 × g ) and re-suspended in 100 μL PBS + 3% bovine serum albumin (BSA). Phycoerythrin conjugated anti-PECAM1-Antibody (Biorbyt, England) was added according to the manufacture and incubated for 30 min at 4 °C in the dark. Stained DPSCs were then measured with a flow cytometer. The measurement was done with an LSR II (BD, USA) using a 532 nm Laser and 575/26 bandpassfilter. Data were analyzed with Flowing Software (Pertu Terho, University of Turku). Events were gated according to forward and sideward scattered light to determine viable cells. Mean signal intensities of the mentioned detector were used for analysis.
2.8
Scratch assay
Cells were incubated in ADM for 20 d and were treated with different concentrations of HEMA (0.1 or 0.5 mM HEMA) or Ethanol (solvent) during the whole differentiation and assay procedure. A 200 μL pipette tip was used to generate a scratch through the confluent cell layer. Cells were then washed with PBS and treated again with HEMA or Ethanol (solvent). Microscopic pictures were taken 0 h, 8 h, 24 h, 32 h, 48 h, and 54 h after applying the scratch. Data are presented as pixels/24 h.
2.9
Statistical analysis
All experiments were run in three independent biological experiments. Additionally, MTT and spheroid sprouting experiments were done with six technical replicates each. Results are represented as mean values ± standard deviation (SD). One-way ANOVA, followed by Dunnett’s multiple comparison test was performed and statistical significance was set for p < 0.05. For antibody array experiments ttests with significance set to p < 0.05 were performed. qRT-PCR experiments were run in duplicate and mean values of three independent biological replicates are shown. For statistical analysis, fold changes were log 2 transformed. All statistical calculations were carried out using GraphPad Prism 6.0 (GraphPad Software, Inc.; La Jolla, CA 92037 USA).
3
Results
3.1
HEMA induced cytotoxicity
An MTT assay was performed to determine the cytotoxicity after up to 72 h of cultivation with HEMA. The cytotoxicity of HEMA is concentration and time dependent ( Fig. 1 ). HEMA concentrations from 4 mM to 8 mM showed significant cytotoxic effects, when the cells were treated for 48 h (4 mM: 73.5 ± 6.9%; 6 mM: 59.1% ± 16.8%; 8 mM 45.9% ± 2.5%) or 72 h (4 mM: 70.5% ± 15.1%; 6 mM: 55.7% ± 11.9%; 8 mM 33.4% ± 11.0%). After 24 h, only DPSCs that were incubated with 8 mM HEMA, showed a significant decrease of cell viability. The EC 50 values (concentration that reduces the metabolic activity to 50% of the untreated control cells) were about 8 mM (48 h) and 6 mM (72 h). In addition, the culture was checked microscopically ( Fig. 2 ). After 7 d of culture, first cytotoxic effects were detected even at concentrations that were ‘sub-toxic’ in the MTT assay. Already at a concentration of 0.75 mM HEMA, cytotoxic effects could be detected microscopically over the differentiation period. When treated with 0.5 mM HEMA, no cytotoxic effects were visible after a differentiation period of 21 d. Therefore, 0.5 mM HEMA was used for any further experiment as highest ‘sub-toxic’ concentration.


3.2
Effect of HEMA on mRNA expression of angiogenic marker genes
The transcription of the angiogenic markers PECAM1 (CD31), VEGF, VEGFR-2 (KDR) and VEGFR-1 (FLT1) was investigated ( Fig. 3 ). Already after seven d a clear increase (2–15-fold) of all tested angiogenic markers in angiogenic medium was visible (except VEGFR-1). In CCM, no significant increase could be observed after seven d. The expression of VEGF and PECAM1 increased to their maximum level by day 21 and then the mean values decreased again. The maximum expression of VEGF receptors was reached after 28 d (VEGFR-2: 13-fold, ADM; VEGFR-1: 7-fold, CCM). The highest regulation in PECAM1 took place after 21 d (32-fold) and decreased to 22-fold after 28 d. VEGFA achieved a 6-fold upregulation compared to undifferentiated cells. If cells were treated with HEMA, a significant downregulation of PECAM1 transcription occured after 28 d. The mRNA level of PECAM1 decreased after 28 d down from 22-fold (SD: 12-fold) to 6-fold (SD: 4-fold) if treated with HEMA. Also VEGFA expression is significant downregulated after 14 d during HEMA treatment. For other time points and marker, there is just a non-significant tendency for downregulation.

3.3
Flow cytometric analysis of PECAM1
The relative PECAM1 amount on the cell surface was determined by flow cytometry ( Fig. 4 ). The average fluorescence signal of the undifferentiated cells (d 0) was 150 relative fluorescence intensity (RFI, SD: 198) and of cells grown in ADM for 21 days 401 RFI (SD: 280), which corresponds to an increase of 167%. Treatment with 0.1 mM HEMA had no effect and resulted in a signal intensity of 379 RFI (SD: 273). A HEMA concentration of 0.5 mM HEMA resulted in an increase of the mean intensity to 771 RFI (SD: 263), which equates to an increase of 92% compared to ADM and a significant increase of 384% compared to the undifferentiated control (d0).

3.4
Protein antibody array
Angiogenesis associated proteins were quantified by a protein antibody array, also known as Proteome Profiler™. 55 different angiogenesis-associated proteins were examined with the protein antibody array after 21 d of differentiation from the cell lysate ( Fig. 5 a). For clarity, a selection of the most important (according to Ucuzian et al. [ ]) and/or most strongly regulated markers is presented in Fig. 5 b and Table 1 . A complete overview of cytokine regulation compared to undifferentiated cells can be found in Supplementary Data 3. The up-regulation of the majority of the angiogenic marker compared to the undifferentiated control is striking. Amphiregulin stands out with a 32-fold higher signal in the ADM sample compared to undifferentiated control, followed by Interleukin-8, VEGF, FGF basic, and FGF-7 (32-fold, 13-fold, 8.5-fold and 5.5-fold). Afterwards, the proteins TIMP-1 and Serpin E1, which cannot be clearly assigned positively or negatively in their role in angiogenesis, give the strongest signals in comparison to the undifferentiated cells (5-fold each). The important angiogenic markers PDGF-AA (2-fold), PDGF-AB (1.5-fold) and angiopoietin-1 and -2 (1.4 and 1.3-fold) are clearly less upregulated. Some of the marker in the HEMA treated samples showed a tendency of a lower expression than in the ADM samples. HEMA induced the strongest repressive effects on interleukin-8 (0.5-fold compared to the solvent control/ADM + EtOH), FGF-7 (0.5-fold), and amphiregulin (0.6-fold) and showed the strongest increasing effects on coagulation factor III (1.8-fold), Serpin F1 (1.5-fold) and angiopoietin-1 (1.4-fold).

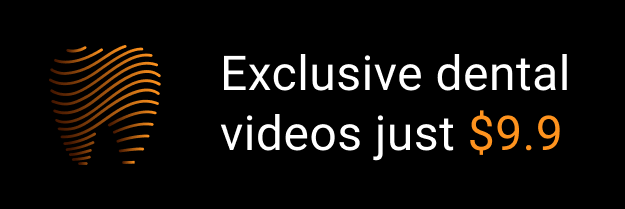