6
Vasoconstrictors
Introduction
Blood vessel constrictors, the second most important drugs in local anesthesia, enhance the efficacy and safety of the primary drug, the local anesthetic itself.
Two families of blood vessel constrictors or vasoconstrictors are presently used in dentistry:
- Sympathomimetics or catecholamines, so‐called because they share a catechol core, including the natural hormones and neurotransmitters epinephrine and norepinephrine, and the synthetic drug levonordefrin.
- Felypressin, a polypeptide derived from the posterior pituitary hormone vasopressin.
The most effective and by far most commonly used vasoconstrictor is epinephrine, oddly enough the first vasoconstrictor ever to be used.
Advantages
As noted, vasoconstrictors heighten local anesthetic effects and safety.
- In dentistry that enhanced efficacy and potency translate into a higher percentage of successful anesthetization of dental pulp, the most difficult tissue to numb (Table 6.1). The mass‐volume‐time effect attributable to the vasoconstrictor’s ability to hold the local anesthetic in place raises the potency of lower doses (1–2% for action comparable to 6–7%).
- Vasoconstrictors lengthen anesthesia duration in both pulp and soft tissues (Table 6.1), likewise as a result of the mass‐volume‐time effect.
- Both epinephrine (Curtis et al. 1966; Meyer and Allen 1968; Hecht and App 1974; Sveen 1979; Buckley et al. 1984; Moore et al. 2007) and felypressin (Shanks 1963; Light et al. 1965; Fisher et al. 1965) reduce hemorrhaging in surgeries by constricting blood vessels. An additional advantage is that they shorten the duration of oral surgeries by affording the surgeon a wider field of view (Hecht and App 1974; Buckley et al. 1984; Moore et al. 2007).
- Vasoconstrictors reduce the toxicity of local anesthetics.
- Experimental studies in animals have shown that the subcutaneous injection of a local anesthetic in conjunction with a vasoconstrictor such as epinephrine (Campbell and Adriani 1958; Henn 1960; Henn and Brattsand 1966) or felypressin (Akerman 1969) lowers the toxicity of the former (Table 6.2). The subcutaneous injection of 2% lidocaine in rats has been shown to disappear in 2 hours, whereas if administered with epinephrine it lasts more than 4 hours (Sung and Truant 1954).
- Clinical studies have found that adding a vasoconstrictor lowers the anesthetic peak (Bromage and Robson 1961; Braid and Scott 1965; Lund and Cwik 1965; Cannell and Beckett 1975a; Perovic et al. 1980) (Table 6.3) and delays its appearance in plasma (Bromage and Robson 1961; Braid and Scott 1965; Lund and Cwik 1965) (Figure 6.1). This is because the slower absorption of the anesthetic (Campbell and Adriani 1958; Lund and Cwik 1965; Cannell and Beckett 1975a) reduces its capacity to reach toxic levels in the blood by giving the body more time to break the drug down.
- The greater potency afforded local anesthetics by vasoconstrictors (see (1) above) also enhances safety indirectly because it enables clinicians to inject smaller quantities to achieve the same effect.
Disadvantages
Vasoconstrictors are characterized by two major drawbacks.
- They heighten the toxicity of intravascularly injected local anesthetics (hence the importance of aspirating after every injection). Experimental studies with animals show that if administered intravenously, anesthetic solutions are more toxic if they bear vasoconstrictors than if they do not (Table 6.2), i.e. exactly the opposite behavior as observed in subcutaneous injections.
- Counterindications and interactions are discussed in Chapter 10.
Table 6.1 Anesthetic efficacy of upper lip and maxillary lateral incisor pulp (measured with electric pulpometer), with and without vasoconstrictors.
Variable | 2% lidocaine | 3% prilocaine | 2% mepivacaine |
---|---|---|---|
No vasoconstrictor | |||
Pulpal anesthesia | 58% | 85% | 83% |
Duration pulpal anesthesia | 6 min | 11 min | 13 min |
Duration lip anesthesia | 67 min | 86 min | 82 min |
Reference | Annex 21 | Berling and Björn (1960) Berling (1966) |
Berling (1958) |
Epinephrine 1:100 000 | |||
Pulpal anesthesia | 95% | 100% | 93% |
Duration pulpal anesthesia | 45 min | 26 min | 35 min |
Duration lip anesthesia | 190 min | 135 min | 160 min |
Reference | Annex 21 | Berling and Björn (1960) | Annex 21 |
Felypressin | 0.01 IU/ml | 0.03 IU/ml | |
Pulpal anesthesia | 82% | 88% | — |
Duration pulpal anesthesia | 15 min | 25 min | — |
Duration lip anesthesia | 141 min | 180 min | — |
Reference | Berling (1966) | Annex 21 |
IU/ml, international units per milliliter.
Table 6.2 Lethal doses (LD50, mg/kg) after subcutaneous or intravenous injection of anesthetic with or without epinephrine.
Subcutaneous injection | Intravenous injection | |||||
---|---|---|---|---|---|---|
Anesthetic | Animal | No epinephrine | With epinephrine | No epinephrine | With epinephrine | Reference |
Procaine | Mouse | — | — | 56 | 22 | Keil and Vieten (1952) |
Mouse | 620 | 670 | 59 | 17 | Henn (1960) | |
Lidocaine | Mouse | — | — | 30 | 14 | Keil and Vieten (1952) |
Mouse | 314 | 317 | 30 | 9 | Henn (1960) | |
Rat | — | — | 28 | 16 | Hardin et al. (1981) | |
Rat | — | — | 28 | 18 | Yagiela (1985) | |
Mepivacaine | Mouse | 285 | 320 | 40 | 20 | Henn (1960) |
Mouse | — | — | 40 | 23 | Henn and Brattsand (1966 | |
Tetracaine | Mouse | — | — | 9.5 | 4.2 | Keil and Vieten (1952) |
Mouse | 62 | 101 | 8 | 2.4 | Henn and Brattsand (1966) | |
Conclusion | Higher toxicity with NO epinephrine, subcutaneous administration | Higher toxicity WITH epinephrine, intravenous administration |
Using felypressin as the vasoconstrictor delivers very similar results (Akerman 1969).
Table 6.3 Peak blood level of local anesthetic (ng/ml) and time to appearance (minutes) after perioral injection of 1.8‐ml cartridge solutions of anesthetic with and without epinephrine and levonordefrin.
Quantity | No vasoconstrictor | With vasoconstrictor a | |||||
---|---|---|---|---|---|---|---|
Anesthetic a | mg | Number of cartridges | ng/ml | Minutes | ng/ml | Minutes | Reference |
Lidocaine | 36 | 1 | 310 | 15 | 220 | 30 | Goebel et al. (1979, 1980a,b) |
Mepivacaine | 36 | 1 | 400 | 10 | 370 | 30 | Goebel et al. (1979, 1980a,b) |
Lidocaine | 80 | 2.2 | 1000 | 10 | 800 | 30 | Cannell and Beckett (1975a) |
Lidocaine | 160 | 4.5 | 1450 | 10 | 1150 | 60 | Cannell and Beckett (1975b) |
a 2% lidocaine with 1:80 000 (12.5 μg/ml) epinephrine; 2% mepivacaine with 1:20 000 (50 μg/ml) levonordefrin.
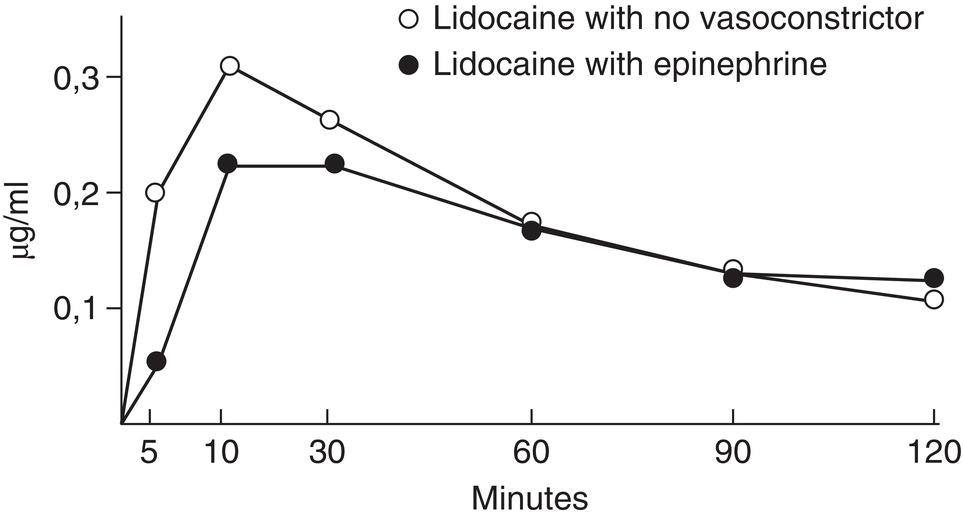
Figure 6.1 Lidocaine vein blood levels with no vasoconstrictor and with epinephrine.
Source: Data from Goebel et al. (1980b).
Dilutions and Concentrations
Vasoconstrictor dilutions are measured as a ratio of parts per thousand, written as, for instance, 1:1000. Doses may also be expressed in milligrams (mg) or micrograms (μg). Concentrations are expressed in milligrams or micrograms per milliliter (mg/ml, μg/ml) or percentage (%). Some examples are listed below.
- A 1:1000 dilution is 1 g (1000 mg) of drug in 1000 ml of solution. Hence:
- 1:1000 = 1 mg/ml = 1000 μg/ml = 0.1%
- One liter (1000 ml) contains 1000 mg (1 g) of drug
- A 1:100 000 dilution is 0.01 g (10 mg) of drug in 1000 ml of solution, whereby:
- 1:100 000 = 0.01 mg/ml = 10 μg/ml = 0.001%
- One liter (1000 ml) contains 10 mg (0.01 g) of drug
- A 1:200 000 dilution is 0.005 g (5 mg) of drug in 1000 ml of solution, whereby:
- 1:200 000 = 0.005 mg/ml = 5 μg/ml = 0.000 5%
- One liter (1000 ml) contains 5 mg (0.005 g) of drug
Table 6.4 lists examples of the vasoconstrictor concentrations most commonly used in dentistry, along with the maximum doses allowed.
Catecholamines
These vasoconstrictors derive their name from the fact that they consist of a catechol group (a benzene ring with two adjacent hydroxy groups) and an amine side chain (Figure 6.2). They are also known as sympathomimetic vasoconstrictors because they act on the autonomic sympathetic nervous system or adrenergic amines because some of them (epinephrine and norepinephrine) are released by the adrenal medulla or are artificial derivatives of such substances (levonordefrin).
Vasoconstrictor sympathomimetics administered in dentistry anesthetic solutions are characterized by three properties.
- In their basic form catecholamines are not water soluble. As hydrochloric salts they, like local anesthetics, dissolve in water, but are unfortunately not stable in that medium. They are consequently used as bitartrates, i.e. the bitartrate salts such as epinephrine bitartrate resulting from the reaction with two molecules of tartaric acid, which are both stable and water soluble (Smith 1920; Bonica 1959).
- They are combined with sulfites, the antioxidizing action of which lengthens catecholamine life because these vasoconstrictors are highly vulnerable to oxidation (Milano et al. 1982; Klein 1983).
- Vasoconstrictor solutions must have a low pH of 2.7–5.5 (USP 38 2015) because otherwise they are oxidized and degraded (Fyhr and Brodin 1987): at a pH >6 epinephrine breaks down in a matter of hours (De Jong and Cullen 1963).
Table 6.4 Maximum doses established for vasoconstrictors and routine concentrations and dilutions.
Vasoconstrictor | Maximum dose μg (mg) | Parts per thousanda | Percentage (%) | μg/ml | mg/ml | μg per 1.8‐ml cartridge | |
---|---|---|---|---|---|---|---|
Sympathomimetic | Levonordefrin | 1000 (1)1 500 (0.5)7 |
1:20 0001 | 0.005% | 50 | 0.05 | 90 |
Norepinephrine | 330 (0.33)1 | 1:30 0002 | 0.00333% | 33 | 0.033 | 59.4 | |
Epinephrine | 200 (0.2)3 | 1:50 0002,3 | 0.002% | 20 | 0.02 | 36 | |
1:80 000 | 0.00125% | 12.5 | 0.0125 | 22.5 | |||
1:100 000 | 0.001% | 10 | 0.01 | 18 | |||
1:200 000 | 0.0005% | 5 | 0.005 | 9 | |||
Felypressin | 7.02 (0.00702)4 | 1:185 00006 | 0.00000054% | 0.545 | 0.00054 | 0.972 | |
(0.39 IU)* | (0.03 IU) | (0.054 IU) |
*IU, international units.
aReferences: 1, Jastak and Yagiela (1983); 2, ADA‐AHA (1964); 3, Report of the Special Committee of the New York Heart Association (1955); 4, Dunlop Committee (Oliver 1974; Roberts and Sowray 1987); 5, Berling (1966), Goldman and Evers (1969); 6, Barnard et al. (1987); 7, Bennett (1984).
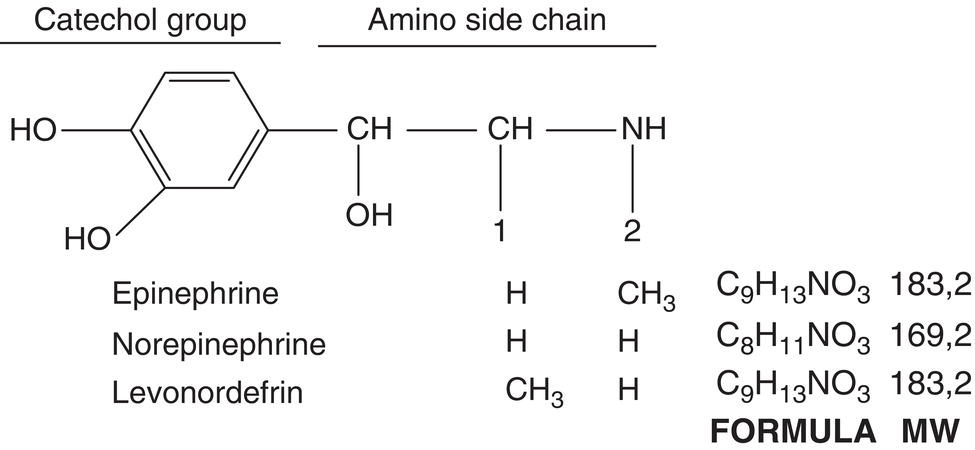
Figure 6.2 Chemical structure common to all catecholamines, showing catechol group and amino side chain: vasoconstrictor drugs differ in side‐chain terminations.
Source: American Dental Association (1984).
Isomers and Catecholamines
The three vasoconstrictive sympathomimetic amines used with local anesthetics in dentistry are isomeric or enantiomeric, i.e. they may present as either of two molecular configurations that differ in the positions of their atoms or atomic groups. As a result, a plane of polarized light can rotate in one of two opposite directions, hence the name optical isomers.
Unlike local anesthetics, most of which adopt racemic forms (DL or ± or RS), i.e. 50% of their molecules are dextrorotated (D or + or R, rectus) and 50% levorotated (L or – or S, sinister), only levorotated catecholamines are used as vasoconstrictors, resulting in a more potent effect (as well as higher toxicity). Levo epinephrine is 15–20 times more potent and more toxic than the dextro form (Table 6.5), while racemic forms are half as potent and toxic.
Hereafter, all references to epinephrine and norepinephrine are understood to be to L‐epinephrine and L‐norepinephrine, while nordefrin is referred to throughout as levonordefrin.
Adrenergic Receptors
Dale (1906) was the first researcher to realize that epinephrine action is mediated by two receptors, although it was Raymond Ahlquist who actually typified the two types of adrenoreceptors: alpha (α) or stimulant and beta (β) or inhibitory receptors (Alquist 1948).
Each type was later subdivided, β receptors into β1 receptors, located primarily in the heart as well as in adipose tissue and the intestine, and the more widely distributed β2 receptors. Their purpose is to relax the smooth muscle in blood vessels and bronchi (Lands et al. 1967).
The α receptors were found to consist, firstly, of post‐synaptic α1 receptors located in the tunica media (edge of the tunica adventitia). Their vasoconstrictive action is triggered by the exogenous catecholamines carried in the bloodstream and the norepinephrine released by nerve endings. The pre‐synaptic α2 receptors in the sympathetic nerve endings block the release of norepinephrine on activation. When located in the tunica intima of vessels they constrict the blood vessels on activation, similar to the response to exogenous catecholamines carried in the bloodstream (Langer 1974; Langer and Hicks 1984).
Today, information deriving from the isolation of the pure proteins comprising adrenoreceptors and functional pharmacological studies suggests the existence of subtypes of α1 (α1a, α1b, α1c, α1d) and α2 (α2a, α2b, α2c) (Ruffolo Jr et al. 1991; Bylund et al. 1994; Hieble 2000; Goldstein 2001), as well as further β receptors, such as β3 (Goldstein 2001; Bylund et al. 1994; Coman et al. 2009) and β4 (Hieble 2000). The identification of adrenoreceptor subtypes and the characterization of their functions may clear the way for the design of future drugs with an optimal pharmacological spectrum (Bylund et al. 1994; Hieble 2000).
Table 6.5 Potency and toxicity of levo over dextro isomers of epinephrine, norepinephrine, and levonordefrin.
Catecholamine | Δ potency levo > dextro | Reference | Δ toxicity levo > dextroa | Reference |
---|---|---|---|---|
Epinephrine | 15–20 | Cushny (1909) Welsh (1955) Hondrum et al. (1993) |
15–20 | Launoy and Menguy (1920) Launoy and Menguy (1922) Hondrum et al. (1993) |
20 | Tye et al. (1967) | |||
Norepinephrine | 25–33 | Tainter et al. (1949) | 10–15 | Hoppe et al. (1949) |
27 | Luduena et al. (1949) | |||
12–18 | Luduena et al. (1949) | |||
10–15 | Welsh (1955) | |||
40 | Tye et al. (1967) | |||
Nordefrin | 100–200 | Luduena et al. (1958) | 10 | Hoppe and Seppelin (1953) |
35 | Tye et al. (1967) | Luduena et al. (1958) |
a Toxicity can be verified in Annex 18.
Adrenoreceptors are transmembrane receptors with an alpha‐helix structure that span the cell membrane seven times. They are G protein‐coupled receptors, i.e. they activate the G proteins, intracellular “switches” that help regulate cell function (Ostrowski et al. 1992). The various parts of this helix structure are depicted schematically in Figure 6.3 (Wolfe and Molinoff 1988; Ruffolo Jr et al. 1991; Ostrowski et al. 1992; Coman et al. 2009).
- The transmembrane segments or domains are the seven “pieces” that span the cell membrane. They are shown as tubes because each one is two to four amino acids thick and labeled with indoarabic numerals (Figure 6.3).
- Six hydrophilic connecting loops connect the seven transmembrane domains, three of which are extracellular (E) and the other three intracellular or cytoplasmic (C). They are shown as lines because each chain is just one amino acid thick.
- The COOH terminal is intracellular and the NH2 terminal is extracellular.
- The extracellular segment has two glycosilation zones (Y).
Each adrenoreceptor, with around 400–560 amino acids, has a molecular weight of 45–70 kDa (Ruffolo Jr et al. 1991; Ostrowski et al. 1992; Coman et al. 2009). Adrenoreceptors differ primarily in the composition of their C3 and cytoplasmic terminal zones (Ruffolo Jr et al. 1991).
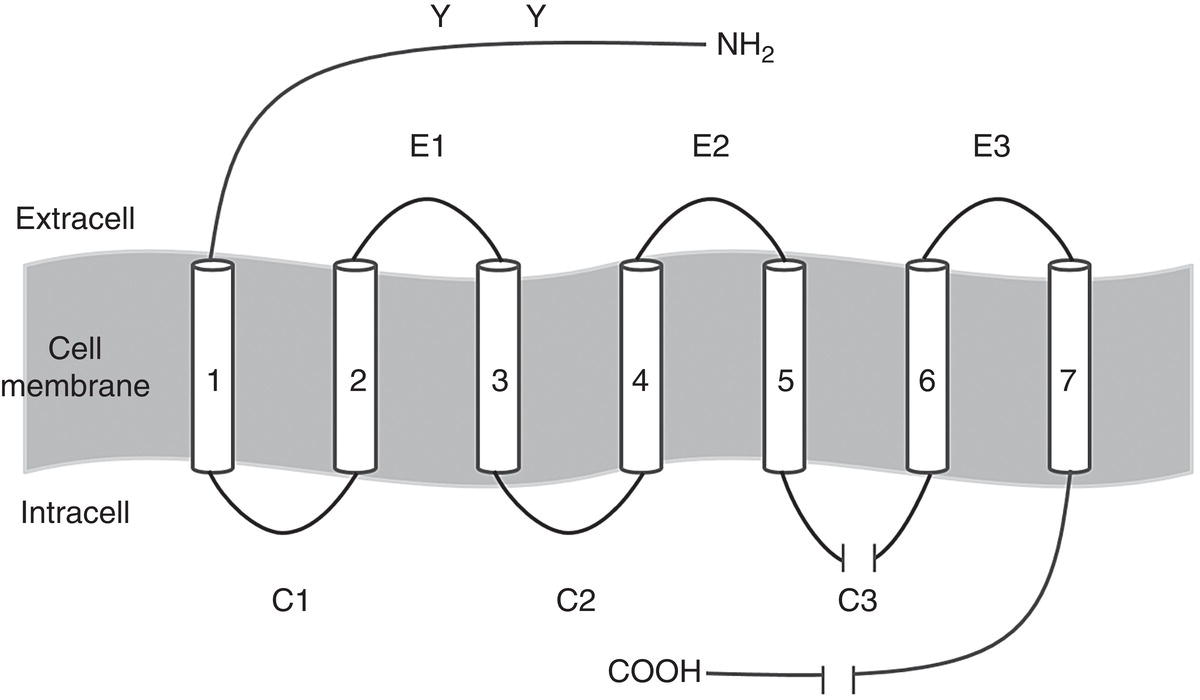
Figure 6.3 Basic structural model common to adrenoreceptors.
Table 6.6 Vasoconstrictor affinity for adrenoreceptors and relative potency (values for levo isomers).
Vasoconstrictor | Adrenoreceptor | Relative potency (%) | |||
---|---|---|---|---|---|
α1 | α2 | β1 | β2 | ||
Epinephrine | + | + | + | + | 100 |
Norepinephrine | + | + | + | − | 25 |
Levonordefrin | − | + | + | − | 15 |
+, stimulates receptor; − does not stimulate receptor.
Today catecholamines are believed to bind to the extracellular portion of the receptors between transmembrane domains 3 and 4 (Ostrowski et al. 1992). The lipid solubility coefficient is consequently unimportant in vasoconstrictor sympathomimetics because contact is made on the surface of the membrane and not, as in local anesthetics, inside the sodium channel.
Not all receptors are stimulated by vasoconstrictive sympathomimetics, nor do all these vasoconstrictors exhibit the same potency. Table 6.6 summarizes their properties: as epinephrine is the most potent catecholamine and stimulates all four main receptors (α1, α2, β1, and β2), it is assigned an activity of 100%. It is fourfold more potent than norepinephrine (Furchgott 1972) and six to seven times more potent than levonordefrin (Robertson et al. 1984). Adrenoreceptor distribution and the major effects of their stimulation are given in Table 6.7 (Keiser 2001; Goldstein 2001). As these receptors tend to seek equilibrium (receptor dynamics), when large quantities of catecholamines are present in the blood for lengthy periods of time they adapt by becoming less sensitive to stimulation. In contrast, when the receptors are not exposed to catecholamines they tend to be overstimulated by adrenergic amines (Brown and Rhodus 2005).
Systemic Effects
Of the many effects induced on the body by vasoconstrictive sympathomimetics (Keiser 2001; Goldstein 2001), the ones of greatest interest to dental clinicians are discussed below.
Heart
The heart bears adrenoreceptors α1, α2, β1, and β2. Receptors α1 and α2 constrict the coronary vessels while receptor β2 induces vasodilation. Receptors β1 and β2 raise heart frequency and strength.
Table 6.7 Adrenoreceptors: distribution and key responses.
Receptor | Location | Response |
---|---|---|
α1 | Heart | ↑ Contraction strength |
Vessels, smooth muscle | Contraction | |
Liver | Glycogenolysis, neoglucogenesis | |
Genitourinary smooth muscle | Contraction | |
Uterus | Pregnancy → contraction | |
Sudoriparous glands | ↑ Perspiration | |
α2 | Vessels, smooth muscle | Contraction |
Pancreas (β cells) | ↓ Insulin secretion | |
Platelets | Platelet aggregation | |
Nerve endings | ↑ Norepinephrine release | |
β1 | Heart | ↑ Contraction strength and frequency |
Juxtaglomerular cells | ↑ Renin secretion | |
β2 | Heart | ↑ Contraction strength and frequency |
Vessels, smooth muscle | Relaxation | |
Liver | Glycogenolysis, neoglucogenesis | |
Pancreas | ↑ Insulin secretion | |
Bronchi, smooth muscle | Relaxation | |
Skeletal muscle | Glycogenolysis and K+ capture | |
Genitourinary smooth muscle | Relaxation | |
Uterus | Pregnant or otherwise → relaxation | |
β3 | Adipose tissue | Lipolysis |
As epinephrine stimulates the four types of receptors it raises cardiac frequency or heart rate (tachycardia) and contraction strength (β1 and β2), and generates an oxygen deficit because the effect of β2‐induced vasodilation is offset by α1 and α2 vasoconstriction.
Norepinephrine, and to a lesser extent levonordefrin, induce coronary vessel vasoconstriction by stimulating α1 and α2, and although they stimulate β1, as they do not bind to receptor β2 the vasodilation effect is minor. The concomitant rise in blood pressure triggers the baroreflex, a feedback mechanism involving the baroreceptors located in the carotid sinus and aortic arch that ultimately reduces heart rate (bradycardia) (Annex 15).
Circulatory System
The arteries and veins of organs, muscles, viscera (lungs, kidneys, genitourinary tract, etc.) have α1, α2, and β2 receptors. As α1 and α2 receptors prevail in most of these vessels, they are constricted by sympathomimetic amines, raising (primarily systolic) blood pressure and peripheral resistance. Skeletal muscle and some abdominal viscera vessels bear readily stimulated vasodilating β2 receptors that tend to lower (primarily diastolic) blood pressure.
Epinephrine stimulates α1 and α2 receptors, inducing vasoconstriction and raising (primarily systolic) blood pressure. It also stimulates vasodilating β2 receptors in skeletal muscle, which tends to lower (primarily diastolic) blood pressure; by balancing systolic and diastolic, it tends to stabilize blood pressure (Annex 15). Systolic and diastolic blood pressure rise simultaneously only when large quantities of epinephrine are injected, stimulating skeletal muscle α receptors (Campbell 1977).
By stimulating only the α receptors, norepinephrine, and less intensely levonordefrin, induce a general rise in both systolic and diastolic blood pressure, given the absence or weakness of the compensatory vasodilating effect induced by β2 (Annex 15).
Respiratory Tract
Here the prevalent effect is receptor β2 stimulation, with relaxation of the bronchial muscles and increased air ingress in the lungs attendant on bronchodilation. That effect is induced by epinephrine almost exclusively because it is the sole catecholamine that stimulates these receptors effectively (Himms‐Hagen 1972).
Endocrine System and Metabolism
Catecholamines stimulate bodily metabolism by raising the number of nutrients to the heart and skeletal muscle, increasing oxygen consumption by 15–30% via the β receptors to prepare the body for the fight‐or‐flight response.
Catecholamines activate glycogenolysis in the skeletal muscles (β2) and glycogenolysis and neoglucogenesis in the liver (α1, β2), releasing glucose into the blood and raising blood glucose levels, a condition that may be highly detrimental to uncontrolled diabetics. By stimulating the β2 receptors in the pancreas, catecholamines intensify insulin release and subsequent glucose entry into the cells, although high levels of epinephrine activate the α2 receptors, notably reducing insulin release (Table 6.7) (Annex 16).
In adipose tissue, via α2, β1, and especially β3 receptors, sympathomimetics induce lipolysis, with the release of glycerol, free fatty acids, and even ketone bodies.
Another effect of stimulating β2 receptors stimulation is hypokalemia, a reduction in plasma potassium levels. While under normal circumstances these actions are of no consequence, in patients taking digoxin (a cardiac stimulant) they may favor heart arrhythmias (Meechan and Rawlins 1988; Meechan et al. 1991).
Uterus
This organ has α1 receptors that stimulate contraction and β2 receptors that favor relaxation, although it is highly impacted by the menstrual cycle and pregnancy.
By acting on both types of receptors (contraction‐simulating α1 and relaxation‐stimulating β2), epinephrine has a neutral effect on the uterus, inhibiting contraction during pregnancy.
As norepinephrine acts primarily on α1 contraction‐stimulating receptors, it intensifies uterus contraction during pregnancy and with it the risk of premature labor or miscarriage (Stepke et al. 1994). As levonordefrin acts on neither receptor, it barely affects the uterus.
Vasoconstrictive Effect
Like the vessels in the skin and mucosa, those in oral tissue (gums, alveolar mucosa, submucosa, periodontium, etc.) have α1 and α2 receptors (Goldstein 2001; Keiser 2001). That, together with the paucity of β receptors, explains why the vasoconstrictive effect of sympathomimetic amines prevails in these areas. These pharmaceuticals induce vasoconstriction defined by a series of special characteristics.
- They act on the entire microcirculatory system, contracting arterioles, meta‐arterioles, precapillary sphincters, and venules (Berde and Cerletti 1964; Altura et al. 1965; Burcher et al. 1977; Olgart and Gazelius 1977).
- They penetrate tissues, in particular the vasa nervorum of nerve trunks, very effectively due to their low molecular weight (Burcher et al. 1977; Olgart and Gazelius 1977).
- The vasoconstrictive effect is immediate (Burcher et al. 1977; Lindorf 1979), with the effect of epinephrine peaking in 3 minutes and lasting around 60 minutes (Lindorf 1979).
- Hypoxia occurs in infiltrated tissue usually due to the rise in local oxygen consumption and decline in oxygen supply due to intense vasoconstriction (Klingenström and Westermar 1964). That in turn induces rebound vasodilation, mediated primarily by epinephrine and norepinephrine (Klingenström and Westermar 1964), which induces a response in 2–3 hours (Lindorf 1979).
Epinephrine has a vasoconstrictive effect four times more potent than that of norepinephrine (Furchgott 1972) and six to seven times more potent than that of levonordefrin (Robertson et al. 1984) (Table 6.6).
Catecholamine Metabolism
Neural sympathomimetic amines (epinephrine and norepinephrine) are released into the circulatory system in two ways.
- Norepinephrine originates primarily in sympathetic nerve endings, with only small amounts released by the adrenal medulla (Landsberg and Young 1980; Goldstein 2001).
- Epinephrine is supplied almost exclusively by the adrenal medulla (Kopin 1989; Goldstein 2001). Of the catecholamines released by the medulla, around 80–85% are epinephrine and the rest norepinephrine.
In the circulatory system 50–60% of these amines bind to plasmatic proteins, primarily albumin (Landsberg and Young 1980), and have a very short (1 minute) mean half‐life in the plasma (Lund 1951; Whitby et al. 1961). They are inactivated in two ways (Landsberg and Young 1980; Trendelenburg 1988; Goldstein 2001):
- Uptake 1. After the post‐synaptic release of norepinephrine, the noradrenergic nerve endings recapture over two‐thirds of the neurotransmitter (Kopin 1989; Eisenhofer et al. 2004), storing it in cytoplasmic vesicles for reuse or metabolizing it by monoamino‐oxidase (MAO)‐mediated deamination in the ribosome membrane. Small amounts of exogenous epinephrine and norepinephrine administered with local anesthetics are also metabolized along this pathway.
- Uptake 2. Non‐nervous cells originating primarily in the liver, but also in the kidneys, lungs, intestines, and other organs, capture circulating catecholamines, which they metabolize by a number of pathways.
- As noted, deamination is mediated by ribosomal MAO, converting catecholamines into aldehydes.
- O‐methylation is catalyzed by the cytoplasmic enzyme catechol‐O‐methyltransferase (COMT). COMT acts directly on the catecholamines or their MAO‐deaminated metabolites by methylizing one of the catechol‐OH groups to produce new methoxy derivatives. This is the predominant catabolic pathway for exogenous catecholamines (Eisenhofer et al. 2004
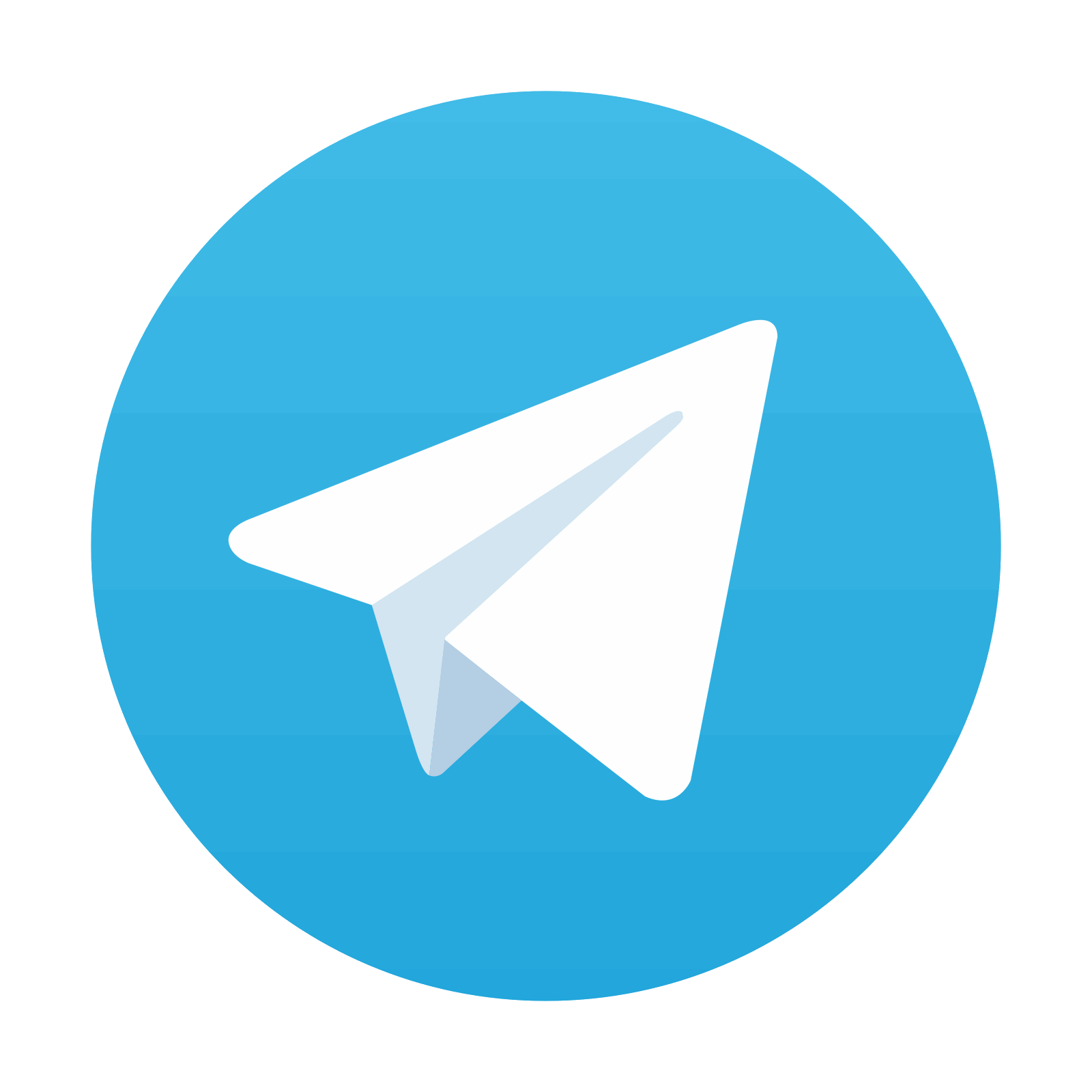
Stay updated, free dental videos. Join our Telegram channel

VIDEdental - Online dental courses
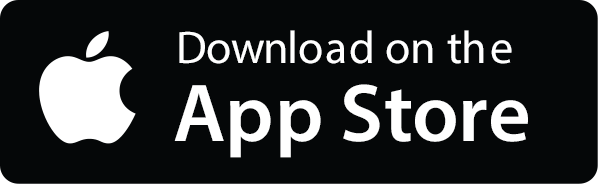
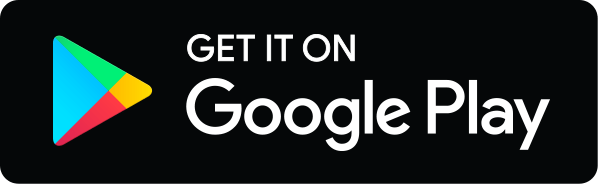