Fig. 5.1
MicroCT scan of pre- and post-instrumentation in a lower molar. Note the amount of walls not touch by the instruments. Lateral view of representative 3D reconstructions of the internal anatomy of a mesial roots of a mandibular molar, before (in green) and after (in red) canal preparation C Coronal, M Middle, A Apical, (Courtesy of Dr Gagliardi, Versiani and Sousa-Neto)
Also, common instrumentation techniques accumulate debris in isthmus areas. Paque et al. showed explained that when rotary files are used in canal with a round cross section, the dentine particles that are cut from the canal wall are carried coronally by the flutes of the file, in a manner similar to that of a common mechanical spiral drill. This removal is apparently less effective when the file has no dentine wall on one side, as is the case of a canal adjacent to an isthmus. Rather than being carried coronally or being contained and packed in the file’s flute space, the debris was most probably actively packed into the area with the least resistance, namely into the isthmus [62].
Dentin Structure
Physiologically and anatomically, the dentin is a complex structure. Type I collagen is the major protein of intertubular dentin (90 %), whereas no collagen fibrils are observed in the peritubular dentin. The structure is a porous configuration with dentinal tubules that allow bacterial invasion and adherence, making dentin disinfection a challenging step (Fig. 5.2).
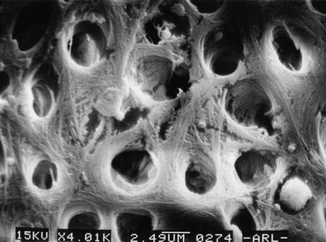
Fig. 5.2
SEM of dentin structure showing irregularities of dentin tissue
Dentin Constituents
The effectiveness of antimicrobial irrigants is known to be compromised under in vivo conditions [77]. In recent studies it has been reported that dentin powder, serum albumin, and dentin matrix can inhibit the antibacterial effect of commonly used irrigants [29, 66, 67]. Interestingly, it has been also reported that even the antibacterial effect of advance disinfection techniques like chitosan nanoparticles and photoactivation disinfection can also be neutralized by the dentin constituents [77].
The microbiological challenge is well covered in Chap. 1. It is important to understand that the endodontic problem is a biofilm-related diseases and access, disruption, or penetration of the biofilm should be our disinfection aim [43].
Endodontic irrigants have three major objectives: chemical, biological, and mechanical. Mechanical objectives include to rinse out debris and lubricate the canal; chemical objectives include to soften and dissolve organic and inorganic tissues, prevent the formation of a smear layer during instrumentation, and dissolve smear layer once it has formed; and biological objectives are related to their antiseptic and nontoxic effects such as efficacy against anaerobic facultative microorganisms (planktonic and biofilms), ability to inactivate endotoxin, nontoxic and noncaustic, and little potential to cause anaphylaxis.
The ideal irrigating solution to disinfect the root canal system should be a biocompatible bactericidal agent, tissue solvent, lubricant, and smear layer remover capable of physically flushing debris, with sustained effect but without affecting the physical properties of the dentin.
The irrigating solutions in endodontics can be classified as antimicrobial solutions, chelating solutions (strong or weak), combinations (antibacterial and chelating solutions combined), and solutions with detergent.
Antimicrobial effects: antiseptic solutions, topical antibiotics, bacteriostatic solutions, and bactericidal solutions.
Antiseptic Solutions
Sodium Hypochlorite
Surveys from around the world [17, 23, 94] reported that sodium hypochlorite is the most common irrigating solution used in endodontics. Figure 5.3 shows the percentage of responders who utilize each irrigant as their primary disinfectant agent in a survey by the American Association of Endodontists. It is an effective antimicrobial and proteolytic agent [48, 49], excellent organic tissue solvent [60], and lubricant with fairly quick effects. NaOCl is both an oxidizing agent and a hydrolyzing agent. Commercial sodium hypochlorite solutions are strongly alkaline and hypertonic and typically have nominal concentrations of 10–14 % available chlorine.
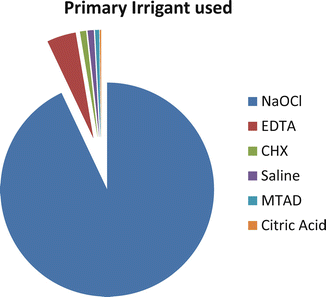
Fig. 5.3
Percentage of responders who utilize each irrigant as their primary disinfectant agent (Reproduced with permission JOE [17])
Mode of Action
Sodium hypochlorite has a pH of 11. Figure 5.4 shows the schematic interaction of the mechanism of action of NaOCl (Reproduced with permission from Estrela et al., Brazilian Endodontic Journal).
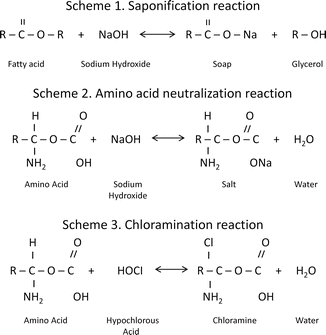
Fig. 5.4
Schematic drawing of NaOCl mechanism of action (Reproduced with permission from [20])
Estrela [20] reported that sodium hypochlorite exhibits a dynamic balance:
-
1: Saponification reaction:Sodium hypochlorite acts as an organic and fat solvent that degrades fatty acids and transforms them into fatty acid salts (soap) and glycerol (alcohol), reducing the surface tension of the remaining solution.
-
2: Neutralization reaction:Sodium hypochlorite neutralizes amino acids by forming water and salt. With the exit of hydroxyl ions, the pH is reduced.
-
3: Hypochlorous acid formation:When chlorine dissolves in water and it is in contact with organic matter, it forms hypochlorous acid. It is a weak acid with the chemical formula HClO that acts as an oxidizer. Hypochlorous acid (HOCl−) and hypochlorite ions (OCl−) lead to amino acid degradation and hydrolysis.
-
4: Solvent action:Sodium hypochlorite also acts as a solvent, releasing chlorine that combines with protein amino groups (NH) to form chloramines (chloramination reaction). Chloramines impede cell metabolism; chlorine is a strong oxidant and inhibits essential bacterial enzymes by irreversible oxidation of SH groups (sulfhydryl group) [20].
-
5: High pH:Sodium hypochlorite is a strong base (pH > 11). The antimicrobial effectiveness of sodium hypochlorite, based on its high pH (hydroxyl ion action), is similar to the mechanism of action of calcium hydroxide. The high pH interferes in cytoplasmic membrane integrity due to irreversible enzymatic inhibition, biosynthetic alterations in cellular metabolism, and phospholipid degradation observed in lipidic peroxidation [20].
Concentration
In the literature, it can be found that NaOCl can be used in a concentration that ranges from 0.5 to 6 %. It was proven that the lower and higher concentrations are equally efficient in reducing the number of bacteria in infected root canal system but the tissue-dissolving effect is directly related to the concentration [26].
Grossman observed pulp tissue dissolution capacity and reported that 5 % sodium hypochlorite dissolved this tissue in between 20 min and 2 h. The dissolution of bovine pulp tissue by sodium hypochlorite (0.5, 1.0, 2.5, and 5.0 %) was studied in vitro under different conditions (Estrela). It was concluded that:
1.
The velocity of dissolution of the bovine pulp fragments was directly proportional to the concentration of the sodium hypochlorite solution and was greater without the surfactant.
2.
Variations in surface tension, from beginning to end of pulp dissolution, were directly proportional to the concentration of the sodium hypochlorite solution and greater in the solutions without surfactant. Solutions without surfactant presented a decrease in surface tension and those with surfactant an increase.
3.
In heated sodium hypochlorite solutions, dissolution of the bovine pulp tissue was more rapid.
4.
The greater the initial concentration of the sodium hypochlorite solutions, the smaller the reduction of its pH (Estrela).
Volume
Volume is more critical for disinfection than its concentration. Frequent exchange with fresh NaOCl is important and the use of large amount of irrigant compensates for the low concentration. It should be kept in mind that the NaOCl will inactivate its components very fast, so fresh irrigating solution should be added to the canal system constantly. (Please see chapter on irrigation dynamics to learn more about the volume.)
Time
How long does NaOCl need to kill bacteria? This question can be misinterpreted in the literature. Some articles will show bacterial killing in 30 min when 0.5 % NaOCl is used, while higher concentrations will need only 30 s to do the same job. Interpretation of results needs to be taken with caution because it will depend on the methods used to test the time. It is important to remember that the presence of organic matter, inflammatory exudates, tissue remnants, and microbial biomass consumes NaOCl and weakens its effect.
The chlorine ion, which is responsible for the dissolving and antibacterial capacity of NaOCl, is unstable and consumed rapidly during the first phase of tissue dissolution, probably within 2 min [57], which provides another reason for continuous replenishment. This should especially be considered in view of the fact that rotary root canal preparation techniques have expedited the shaping process. The optimal time that a hypochlorite irrigant at a given concentration needs to remain in the canal system is an issue yet to be answered [96].
Effect on the Dentin
As it was stated before, the dentin is composed of 22 % organic material by weight. Most of this consists of type I collagen, which contributes considerably to the mechanical properties of the dentin. NaOCl solutions may affect mechanical dentin properties via the degradation of organic dentin components.
Depth of Penetration
The depth of NaOCl penetration varied between 77 and 300 μm, and it depends on concentration, time, and temperature [99]. Figure 5.5 illustrates a microscopic view of stained root section treated by 1 % sodium hypochlorite for 2 min (Published with permission).
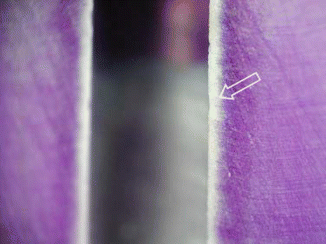
Fig. 5.5
A microscope view of stained root section treated by 1 % sodium hypochlorite for 2 min (arrow) (Reproduced with permission [99])
Effect on Biofilms
Clegg et al. [12] demonstrated that 6 % NaOCl was the only agent capable of both physically removing artificial biofilm and killing bacteria. There was a dose-dependent effect of NaOCl against bacteria, as higher concentrations were more antibacterial. Figure 5.6 illustrates the effect of different irrigants on dentin biofilm elimination. In summary, 3 % and 6% NaOCl showed absence of biofilm, 1 % NaOCl showed disruption of biofilm, and 2 % CHX showed intact biofilm (Fig. 5.6).

Fig. 5.6
(a) Scanning electron micrograph (SEM) of bacteria-free dentin on negative control specimen (original magnification ×3,000). (b) SEM of positive control reveals cocci, rods, and filamentous organisms (original magnification ×5,000). (c) SEM of dentin section treated with 6 % NaOCl. No bacteria are visible (original magnification ×5,000). (d) SEM of dentin section treated with 2 % CHX. The biofilm is intact with no visible disruption (original magnification ×5,000) (Reproduced with permission from JOE [12])
Limitations
Unfortunately, even though NaOCl has many ideal properties, it has some limitations such as being toxic [39, 48] (see more details in Chap. 7), nonsubstantive, ineffective in smear layer removal and corrosive. It may cause discoloration [40] and has unpleasant odor. When NaOCl is used as a final rinse, bonding of the sealer to the dentin may be altered [72].
Clinical Recommendation
NaOCl in concentrations between 2.5 and 6 % should be used during the whole cleaning and shaping procedure. Pulp chamber should be used as a reservoir of fresh irrigant. Once the mechanical preparation is finished and a master apical file is determined, the protocol of irrigation should start with the activation of fresh NaOCl in each canal [27].
Chlorhexidine Gluconate (CHX) [6]
Molecular Structure
CHX is a strongly basic molecule with a pH between 5.5 and 7 that belongs to the polybiguanide group and consists of two symmetric four-chlorophenyl rings and two biguanide groups connected by a central hexamethylene chain. CHX digluconate salt is easily soluble in water and is very stable [25].
Mode of Action
Chlorhexidine, because of its cationic charges, is capable of electrostatically binding to the negatively charged surfaces of bacteria [14], damaging the outer layers of the cell wall and rendering it permeable [33, 36, 37]. CHX is a wide-spectrum antimicrobial agent, active against gram-positive and gram-negative bacteria and yeasts [16].
Depending on its concentration, CHX can have both bacteriostatic and bactericidal effects. At high concentrations, CHX acts as a detergent and exerts its bactericidal effect by damaging the cell membrane and causes precipitation of the cytoplasm. At low concentrations, CHX is bacteriostatic, causing low-molecular-weight substances (i.e., potassium and phosphorous) to leak out from the cell membrane without the cell being permanently damaged.
Substantivity
Due to the cationic nature of the CHX molecule, it can be absorbed by anionic substrates such as the oral mucosa and tooth structure [54, 73, 92]. CHX is readily adsorbed onto hydroxyapatite and teeth. Studies have shown that the uptake of CHX onto the teeth is reversible [34]. This reversible reaction of uptake and release of CHX leads to substantive antimicrobial activity and is referred to as substantivity. This effect depends on the concentration of CHX. At low concentrations of 0.005–0.01 %, only a constant monolayer of CHX is adsorbed on the tooth surface, but at higher concentrations, a multilayer of CHX is formed on the surface, providing a reservoir of CHX which can rapidly release the excess into the environment as the concentration of CHX in the surrounding environment decreases [19]. Time and concentration of CHX can influence the antibacterial substantivity and the conclusions are inconsistent. Some studies demonstrated that 4 % CHX has greater antibacterial substantivity than 0.2 % after 5 min application (332). Other studies stated that CHX should be left for more than 1 h in the canal to be adsorbed by the dentin [50]. Komorowski et al. [45] suggested that a 5-min application of CHX did not induce substantivity, so the dentin should be treated with CHX for 7 days. However, when Paquette et al. [63] and Malkhassian et al. [55] in their in vivo studies medicated the canals with either liquid or gel forms of CHX for 1 week, neither of them could achieve total disinfection. Therefore, residual antimicrobial efficacy of CHX in vivo still remains to be demonstrated.
Chlorhexidine as an Endodontic Irrigant
CHX has been extensively studied as an endodontic irrigant and intracanal medication, both in vivo (Barbosa, Linkgog, Manzur, Paquette, Malkhassian) and in vitro [4, 5, 9, 10, 51, 56].
The antibacterial efficacy of CHX as an irrigant is concentration dependent. It has been demonstrated that 2 % CHX has a better antibacterial efficacy than 0.12 % CHX in vitro ([10]). When comparing its effectiveness with NaOCl, controversial results can be found. NaOCl has an obvious advantage over CHX with the dissolution capacity of organic matter that CHX lacks; therefore, even though in vitro studies suggest some advantages with the use of CHX, as soon as organic and dental tissue is added, NaOCl is clearly preferable.
The antibacterial effectiveness of CHX in infected root canals has been investigated in several in vivo studies. Investigators [70] reported that 2.5 % NaOCl was significantly more effective than 0.2 % CHX when the infected root canals were irrigated for 30 min with either of the solutions.
In a controlled and randomized clinical trial, the efficacy of 2 % CHX liquid was tested against saline using culture technique. All the teeth were initially instrumented and irrigated using 1 % NaOCl. Then either 2 % CHX liquid or saline was applied as a final rinse. The authors reported a further reduction in the proportion of positive cultures in the CHX group. Their results showed a better disinfection of the root canals using CHX compared to saline as a final rinse [95].
In a recent study, the antibacterial efficacy of 2 % CHX gel was tested against 2.5 % NaOCl in teeth with apical periodontitis, with the bacterial load assessed using real-time quantitative polymerase chain reaction (RTQ-PCR) and colony-forming units (CFU). The bacterial reduction in the NaOCl group was significantly greater than the CHX group when measured by RTQ-PCR. Based on culture technique, bacterial growth was detected in 50 % of the CHX group compared to 25 % in the NaOCl group [93]. On the other hand, another study based on this culture technique revealed no significant difference between the antibacterial efficacy of 2.5 % NaOCl and 0.12 % CHX liquid when used as irrigants during the treatment of infected canals [80].
In a recent systematic review, Ng et al. [59] demonstrated that abstaining from using 2 % CHX as an adjunct irrigant to NaOCl was associated with superior periapical healing.
Unlike NaOCl, CHX lacks a tissue-dissolving property. Therefore, NaOCl is still considered the primary irrigating solution in endodontics.
Allergic Reactions to Chlorhexidine
Allergic responses to CHX are rare, and there are no reports of reactions following root canal irrigation with CHX [2, 39]. The sensitization rate has been reported in several studies to be approximately 2 % [47]. However, some allergic reactions such as anaphylaxis, contact dermatitis, and urticaria have been reported following direct contact to mucosal tissue or open wounds [18, 65, 74, 81].
Limitations
The limitations of using CHX as a primary and sole endodontic irrigant are the following: the inability to dissolve organic matter, no action on smear layer, and minor effect on biofilm disruption.
Clinical Recommendations
The clinical recommendation to use CHX during endodontic treatment:
1.
In teeth with open apices or perforation where there is a risk to extrude NaOCl.
2.
When maximal antimicrobial effect is desirable as a final rinse after EDTA to further facilitate disinfection and to improve dentin bonding (where relevant) [30].
Decalcifying Agents
Debris is defined as dentin chips or residual vital or necrotic pulp tissue attached to the root canal wall. Smear layer was defined by the American Association of Endodontists in 2003 as a surface film of debris retained on the dentin or other surfaces after instrumentation with either rotary instruments or endodontic files; it consists of dentin particles, remnants of vital or necrotic pulp tissue, bacterial components, and retained irrigants. While it has been viewed as an impediment to irrigant penetration into dentinal tubules, there is still a controversy about the influence of smear layer on the outcome of endodontic treatment. Some researchers emphasize the importance of removing the smear layer to allow irrigants, medications, and sealers to penetrate into the dentinal tubules and improve disinfection. On the other hand, other researchers focused on keeping the smear layer as a protection for bacterial invasion, apical and coronal microleakage, bacterial penetration of the tubules, and the adaptation of root canal materials. The majority of the conclusions on smear layer are based on in vitro studies. A recent clinical study by Ng et al.[59] found that the use of EDTA significantly increased the odds of success of retreatment cases by twofold.
The chelating agents can be classified as strong or weak. Strong chelating agents are EDTA, citric acid, and chitosan nanoparticles, while weak chelating agent is HEBP or etidronate.
Ethylenediaminetetraacetic Acid
Ethylenediaminetetraacetic acid, widely abbreviated as EDTA, is an aminopolycarboxylic acid, and a colorless, water-soluble solid. EDTA is often suggested as an irrigant because it can chelate and remove the mineralized portion of the smear layer. It is a polyaminocarboxylic acid with the formula [CH2N(CH2CO2H)2]2. Its prominence as a chelating agent arises from its ability to sequester di- and tricationic metal ions such as Ca2+ and Fe3+. After being bound by EDTA, metal ions remain in solution but exhibit diminished reactivity.
History
The compound was first described in 1935 by Ferdinand Munz, who prepared the compound from ethylenediamine and chloroacetic acid. Chelating agents were introduced into endodontics as an aid for the preparation of narrow and calcified root canals in 1957 by Nygaard-Østby [38]. Today, EDTA is mainly synthesized from ethylenediamine (1, 2-diaminoethane), formaldehyde (methanal), and sodium cyanide [38].
Mode of Action
On direct exposure for extended time, EDTA extracts bacterial surface proteins by combining with metal ions from the cell membrane which can eventually lead to bacterial death [38]. Chelators such as EDTA form a stable complex with calcium. When all available ions have been bound, equilibrium is formed and no further dissolution takes place; therefore, EDTA is self-limiting [38].
Applications in Endodontics
EDTA alone normally cannot remove the smear layer effectively; a proteolytic component, such as NaOCl, must be added to remove the organic components of the smear layer [22]. For root canal preparation, EDTA has limited value alone as an irrigation fluid [22]. EDTA is normally used in a concentration of 17 % and can remove the smear layer when in direct contact with the root canal wall for less than 1 min.
Although citric acid appears to be slightly more potent at similar concentration than EDTA, both agents show high efficiency in removing the smear layer. In addition to their cleaning ability, chelators may detach biofilms adhering to root canal walls [28]. This may explain why an EDTA irrigant proved to be highly superior to saline in reducing intracanal microbiota despite the fact that its antiseptic capacity is relatively limited [28].
The effect of chelators in negotiating narrow, tortuous, calcified canals to establish patency depends on both canal width and the amount of active substance available, since the demineralization process continues until all chelators have formed complexes with calcium [38, 98]. Therefore, studies should be read with caution because one study can show demineralization up to a depth of 50 μm into the dentin [38], but other reports demonstrated significant erosion after irrigation with EDTA [89, 91]. The sequence in which root canal wall dentin is exposed to NaOCl and EDTA has an impact on the level of dentin erosion on the main root canal wall.
In the study reported by Qian et al. [69] no erosion was detected when demineralizing agents were used as a final rinse after NaOCl. However, the erosion of peritubular and intertubular dentin was detected when EDTA was used first followed by 5.25 % NaOCl.
Interaction Between CHX and NaOCl
The combination of NaOCl and CHX produces a change of color and a precipitate. The reaction is dependent of the concentration of NaOCl. The higher the concentration of NaOCl, the larger the precipitate is if 2 % CHX is used [7] (Fig. 5.7). Furthermore, concerns have been raised that the color change may have some clinical relevance causing staining of the tooth. Also the resulting precipitate might interfere with the seal of the root canal obturation. Basrani et al. [7] evaluated the chemical nature of this precipitate and reported the formation of 4-chloroaniline (PCA). Furthermore, a recent study [44] (Fig. 5.8) using TOF-SIMS analysis showed the penetration of PCA inside dentinal tubules. PCA has been shown to be toxic in humans with short-term exposure, resulting in cyanosis, which is a manifestation of methemoglobin formation. The interaction should be avoided by using EDTA or other irrigants after NaOCl and before CHX or alternatively, the canals can be dried using paper points before the final rinse [98].
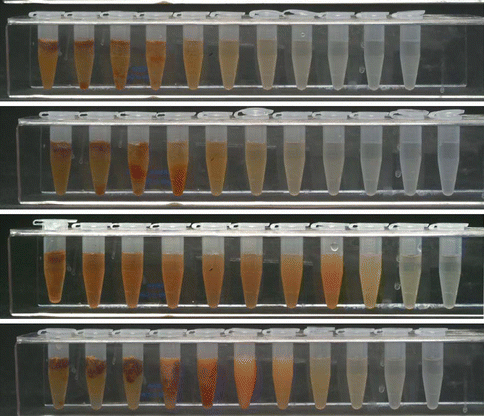
Fig. 5.7
Interaction between different concentrations of NaOCl and 2.0 % CHX. Note that the higher the concentration of NaOCl, the higher the amount of precipitate [7]
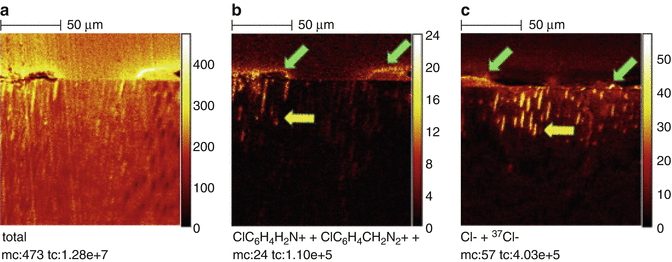
Fig. 5.8
Dentin treated with CHX and NaOCl and analyzed by High-spatial-resolution TOF-SIMS images of ion distribution in longitudinal sections of dentin: Pulp space is on topmost and dentin bottom-most in each image. Note irregular precipitate on surface (green arrows), the extension of PCA and CHX breakdown products, in addition to chlorine, into Dentinal tubules (yellow arrows). (a) ‘‘Total’’ shows raw image; ClC6H4 H2N+ + ClC6H4CH2N2+ show distribution of PCA and CHX breakdown products, and Cl _+ 37Cl_ show distribution of chlorine. (b) Positive ion of CHX group. (c) Negative ion of CHX group [44]
Interaction Between CHX and EDTA
The combination of CHX and EDTA produces a white precipitate, so a group of investigators [70] did a study to determine whether the precipitate involves the chemical degradation of CHX. The precipitate was produced and redissolved in a known amount of dilute trifluoroacetic acid. Based on the results, CHX was found to form a salt with EDTA rather than undergoing a chemical reaction (Fig. 5.9).
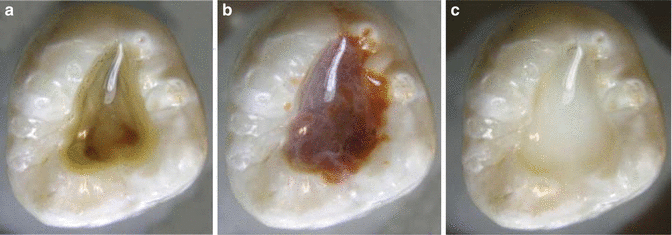
Fig. 5.9
Endodontic access cavities containing CHX mixed with various irrigants. (a) Water, (b) NaOCl, and (c) EDTA. Note that NaOCl and EDTA cause CHX to form a precipitate (Reproduced with permission from [70])
Interaction Between EDTA and NaOCl
Investigators [24] studied the interactions between EDTA and NaOCl. They concluded that EDTA retained its calcium-complex ability when mixed with NaOCl, but EDTA caused NaOCl to lose its tissue-dissolving capacity, with virtually no free chlorine detected in the combinations. Clinically, this suggests that EDTA and NaOCl should be used separately. In an alternating irrigating regimen, copious amounts of NaOCl should be administered to wash out remnants of the EDTA. In modern endodontics, EDTA is used once the cleaning and shaping is completed for around 1 min. It can be ultrasonically activated for better penetration in dentinal tubules. It should be taken into consideration that a rise on the temperature of EDTA is not desirable. Chelators have a temperature range wherein they can work at their best. When EDTA is heated from 20 to 90°, the calcium-binding capacity decreases [97].
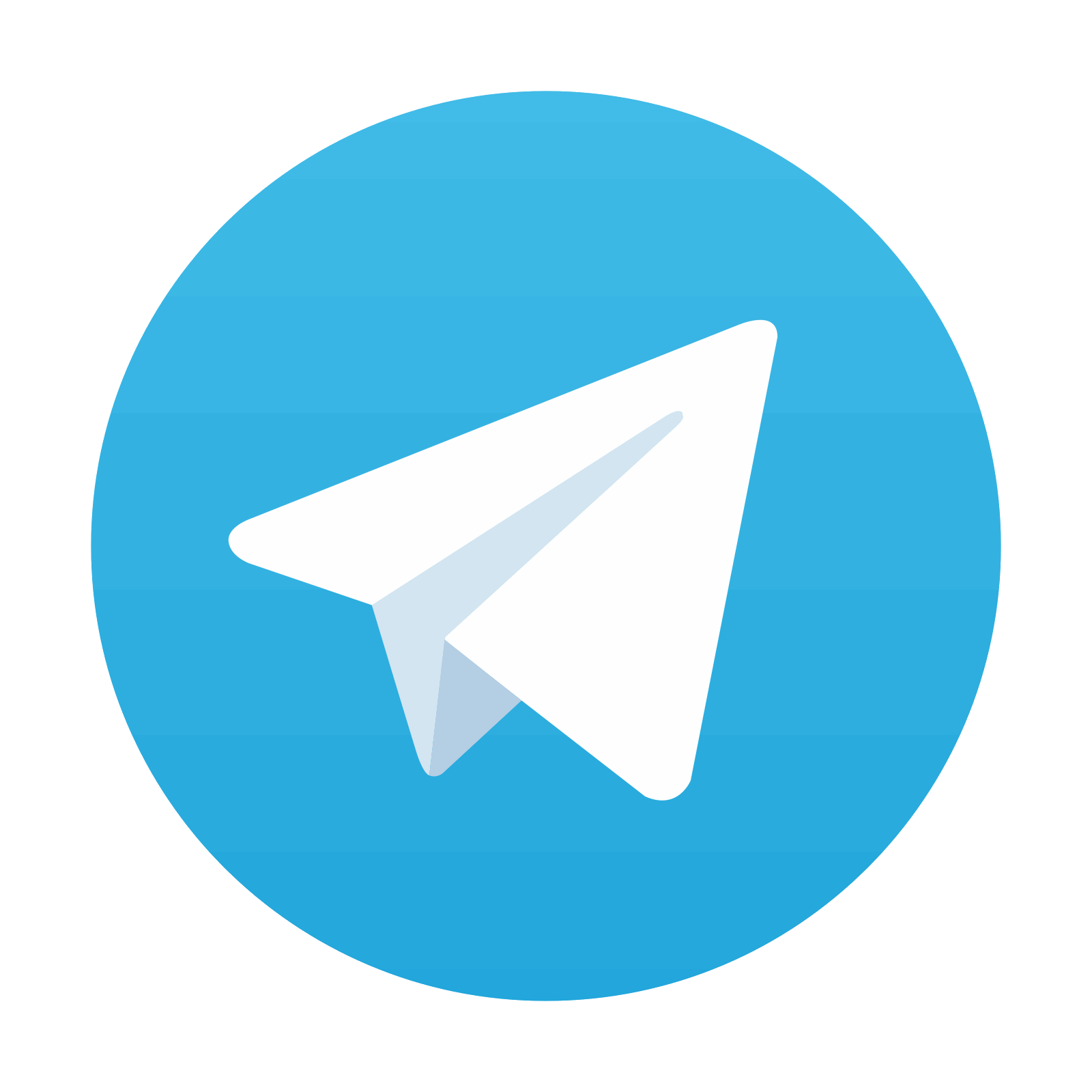
Stay updated, free dental videos. Join our Telegram channel

VIDEdental - Online dental courses
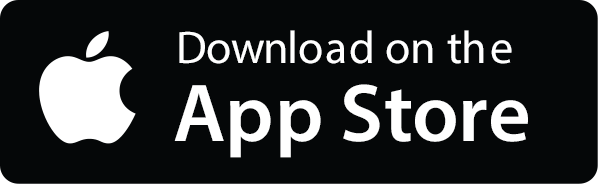
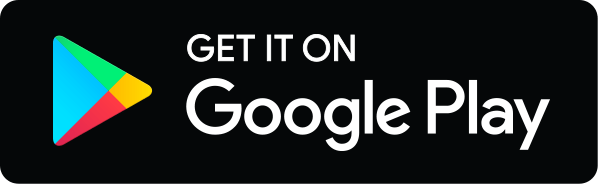