, Hai-Yang Yu2, Jing Zheng1, Lin-Mao Qian1 and Yu Yan3
(1)
Tribology Research Institute, Southwest Jiaotong University, Chengdu, People’s Republic of China
(2)
West China College of Stomatology, Sichuan University, Chengdu, People’s Republic of China
(3)
Institute of Advanced Materials and Technology, University of Science and Technology, Beijing, People’s Republic of China
Abstract
In restorative dentistry, metals and alloys and ceramics and composites are generally applied to restorations and implants [1–7]. Different forming/processing methods and the main clinical applications of dental restorative materials are shown in Table 6.1. Considering the complex interoral environment and biomechanics, the wear processes of artificial dental materials are very complicated and normally include abrasion, attrition, corrosion, fretting wear, and fatigue [8, 9]. These processes occur in various combinations to cause surface loss of materials in the mouth. Excessive wear may lead to premature failure and the replacement of dental restorations and implants. The wear resistance of artificial dental materials is clinically important for clinical longevity, aesthetics, and resistance to dental plaque [8–10]; therefore, a large number of studies have been carried out on their tribological properties. Recreating function and aesthetics are the two practical goals of restorative treatments, but the inadequate wear resistance of either the restoration or the implant may cause obvious defects in its anatomic shape due to excessive wear by friction during chewing, changing the shape and functions of teeth, bone, and masticatory muscle, further affecting the fitness of the stomatognathic system. Therefore, it is necessary to remember that the choice of material depends on a number of factors, such as corrosion behavior, mechanical properties (including strength and wear resistance), cost, availability, biocompatibility, and aesthetic values.
In restorative dentistry, metals and alloys and ceramics and composites are generally applied to restorations and implants [1–7]. Different forming/processing methods and the main clinical applications of dental restorative materials are shown in Table 6.1. Considering the complex interoral environment and biomechanics, the wear processes of artificial dental materials are very complicated and normally include abrasion, attrition, corrosion, fretting wear, and fatigue [8, 9]. These processes occur in various combinations to cause surface loss of materials in the mouth. Excessive wear may lead to premature failure and the replacement of dental restorations and implants. The wear resistance of artificial dental materials is clinically important for clinical longevity, aesthetics, and resistance to dental plaque [8–10]; therefore, a large number of studies have been carried out on their tribological properties. Recreating function and aesthetics are the two practical goals of restorative treatments, but the inadequate wear resistance of either the restoration or the implant may cause obvious defects in its anatomic shape due to excessive wear by friction during chewing, changing the shape and functions of teeth, bone, and masticatory muscle, further affecting the fitness of the stomatognathic system. Therefore, it is necessary to remember that the choice of material depends on a number of factors, such as corrosion behavior, mechanical properties (including strength and wear resistance), cost, availability, biocompatibility, and aesthetic values.
Table 6.1
Current artificial dental materials
Material type
|
Forming/processing method
|
Typical application
|
|
---|---|---|---|
Metals and their alloys
|
Amalgam
|
Low copper
|
Posterior restoration
|
High copper
|
Posterior restoration
|
||
Noble alloys
|
Gold (nonheat-treatable)
|
Restoration of single teeth
|
|
Gold (heat-treatable)
|
Fixed bridges
|
||
Palladium–silver
|
Restoration of single teeth
|
||
Base alloys
|
Nickel
|
Restoration of single and missing teeth, partial denture frameworks
|
|
Cobalt partial
|
Denture framework and implants
|
||
Iron
|
Orthodontic appliances and endodontic instruments
|
||
Titanium
|
Implants
|
||
Ceramics
|
Feldspathic porcelain
|
Condensed and sintered
|
Veneers, inlays, anterior crowns
|
Leucite-reinforced porcelain
|
Pressure molded
|
Veneers, inlays, anterior and posterior crowns
|
|
Silicate-reinforced porcelain
|
Pressure molded
|
Anterior and posterior crowns, anterior bridges
|
|
Al2O3-reinforced porcelain
|
Condensed and sintered
|
Anterior crowns
|
|
Alumina core ceramic
|
Dry-pressed and sintered
|
Anterior and posterior crowns, anterior bridges
|
|
Glass-infiltrated core ceramic
|
Slip cast, sintered, and infiltrated with glass
|
Anterior and posterior crowns, anterior bridges
|
|
Machinable ceramic
|
Milled or ground
|
Veneers, inlays, anterior and posterior crowns
|
|
Zirconia core ceramic
|
Dry-pressed and sintered
|
Posterior crowns and bridges
|
|
Unfilled polymers and composites
|
Lightly filled or unfilled composite
|
Adhesives
|
|
Macrofill composite
|
|||
Microfill composite
|
Homogeneous microfill
|
Restorations and crowns of anterior and posterior teeth, inlays, onlays, veneer
|
|
Heterogeneous microfill
|
|||
Hybrid composite
|
Composed 70–80 % glass fillers and 20–30 % nanofillers polyacid-modified
|
||
Compomers fibrous composite
|
Carbon fibers for the core and glass fibers for sheathing
|
Obturation materials for dental caries
|
|
Dental post, orthodontic arch-wires and brackets
|
|||
Glass ionomer
|
Resin-modified
|
Adhesives, obturation materials for dental caries
|
|
Metal-reinforced
|
Adhesives, obturation materials for dental caries
|
6.1 Wear Behavior of Resin-Based Restorative Materials
Dental composites are key materials for dental restorations due to their aesthetics, chemical inertness, biocompatibility, and convenient clinical manipulation. They are used to restore the function, integrity, and morphology of missing tooth structure. Generally, dental composites are divided into two types: direct dental restorative composites and indirect dental restorative composites. The former is utilized in a single procedure in the mouth by placing soft and malleable filling materials into the prepared tooth and building up the tooth before the material sets. Indirect dental restorative composites, which are laboratory-processed to be utilized for indirectly fabricated inlay, onlay, and crown restorations [11], are becoming increasingly important in dentistry, with expanding applications resulting from processing and materials innovations [12, 13]. Some of them have shown clinical advantages, particularly a substantially higher mechanical resistance than other currently available composites [14, 15]. Many of the improvements in the indirect dental composites—particularly those stemming from the filler particle type, the increased degree of cure (DC) of monomers, or an improved filler/matrix adhesion—are based on materials property measurements [13, 16, 17]. Resin-based composite materials have gained in popularity in restorative dentistry recently because the color resembles natural tooth color. In addition, the acid-etch technique brings a number of conveniences for the composite restoratives to reconstruct the shape of teeth. However, remarkable improvements in the component elements and processing techniques fail to avoid the excessive wear of composite resins during mastication, and this wear rate is far more significant than that of metals or ceramics, which is supposed to limit the wide use of composite materials in indirect restoration.
Generally, the wear process of composite materials is related to the material’s characteristics and oral condition. Material factors usually include the characteristics, content, and distribution of filler; the nature of the matrix; and the interfacial bond strength between the filler and the matrix.
Filler characteristics, such as size, shape, hardness, and brittleness, play an important part in the wear process of dental composites. Compared to composites containing large filler particles (>1 μm), microfilled and small-particle hybrid composites were frequently mentioned to have a tendency to display better abrasion resistance because of their smoother surface, decreased interparticle spacing, and decreased friction to food particles [18]. In contrast to sharp and pointed particles, the presence of spherical and irregular particles could benefit both the composite wear and the opposing enamel wear [19]. Hard filler particles could protect the soft matrix and enhance the material’s overall resistance to abrasion [20]; however, the hardness of filler particles must not be higher than that of human enamel hydroxyapatite crystals. Under high stress, brittle filler particles will easily fracture and cause rapid abrasion of the material itself but have a less antagonistic engagement. It is well accepted that a smooth surface provides reduced frictional wear at the occlusal contact area, which will lessen the wear of both composite and antagonistic enamels. Whenever filler particles protrude and are big and extremely hard, there can be high antagonistic wear rates, which could cause catastrophic loss of the tooth substance in time [20, 21].
Obviously, the ability of a resin composite to resist abrasive action depends on filler–matrix interactions. Therefore, the filler content and distribution and interparticle spacing significantly influence the physical properties and thus the wear resistance of dental composites [20]. The wear resistance of microfilled composites was remarkably enhanced by the filler volume, with an increase from 25 to 30 vol%. Filler particles situated very close to each other can protect the softer resin matrix from abrasives, thus reducing wear. The use of finer particles for a fixed-volume fraction of filler resulted in decreased interparticle space and reduced wear [22]. Resin composites with a high filler content and good filler distributions, such as Filtek P60, were reported to possess better wear resistance than nano-filled composites, like VITA ZETA and VITA LC, with a relatively low filler weight.
As for the resin matrix, cure conditions play an essential role in its composition and further affect the nature of the matrix. When the two main components of a chemical-cured composite resin interact with each other, the intermingling step introduces desirable air within it easily, and inevitably; after curing, the air is turned into scattered tiny pores, which decrease the hardness of the resin matrix and exert bad effects on its wear resistance. Conversely, due to the exhaust-gas disposal and no intermingling step, the porosity of light-cured composites is significantly lower than that of chemical-cured composites, which can improve the strength of the matrix and the wear resistance of composites.
Another key factor in the wear of composites is a good interfacial bond between the filler and the matrix. Good filler–matrix adhesion was indicated to enhance the stress-transfer ability, protect the matrix, and interfere with crack propagation at higher filler levels and accordingly reduce wear [20, 23]. Silane coupling agents are thought to play a major role in enhancing the adhesion of the interface between the inorganic filler and the organic resin. It is important that the microfillers need to be kept well dispersed to achieve the full effect of protection. In addition, contamination of the filler might disturb silanization and reduce filler–matrix coupling. Another advantage of the use of a coupling agent in dental composites is that it, at least to some extent, protects the filler against hydrolytic degradation.
Oral factors, in particular, the oral chemical environment, have a significant effect on the wear process and in vivo degradation of composite restorations. The resin matrix, prone to aging as a polymer material, can be softened and fillers can be leached out when composites are exposed to certain chemicals/food-simulating liquids [20, 24] and significant temperature/stress changes, thus reducing the resistance against wear to a considerable extent. A high degree of cure of the composite matrix could decrease its degradation in long-term exposure to water. It should be noted that there is a rising failure rate of posterior resin composite restorations due to interproximal wear [20, 23]. Furthermore, wear of crowns and bridges made of composites is generally greater than that of fillings if they are analyzed after longer periods of service [25].
Zheng et al. [26] investigated the influence of the oral environment on the tribological behavior of resin teeth sliding against a TA2 titanium ball. They found that with the normal force increasing, the wear mechanism of resin teeth changed from slight abrasive wear to severe adhesive wear, and the wear resistance was reduced. Temperature fluctuation in the oral cavity could affect the durability of the resin complex. In general, the temperature inside the oral cavity is 35.2 °C ± 2.1 °C [27], and it could fluctuate within the range of approximately 0–60 °C. Zheng et al. found that thermal cycling between 0 °C and 60 °C can significantly reduce the durability of resin materials [26].
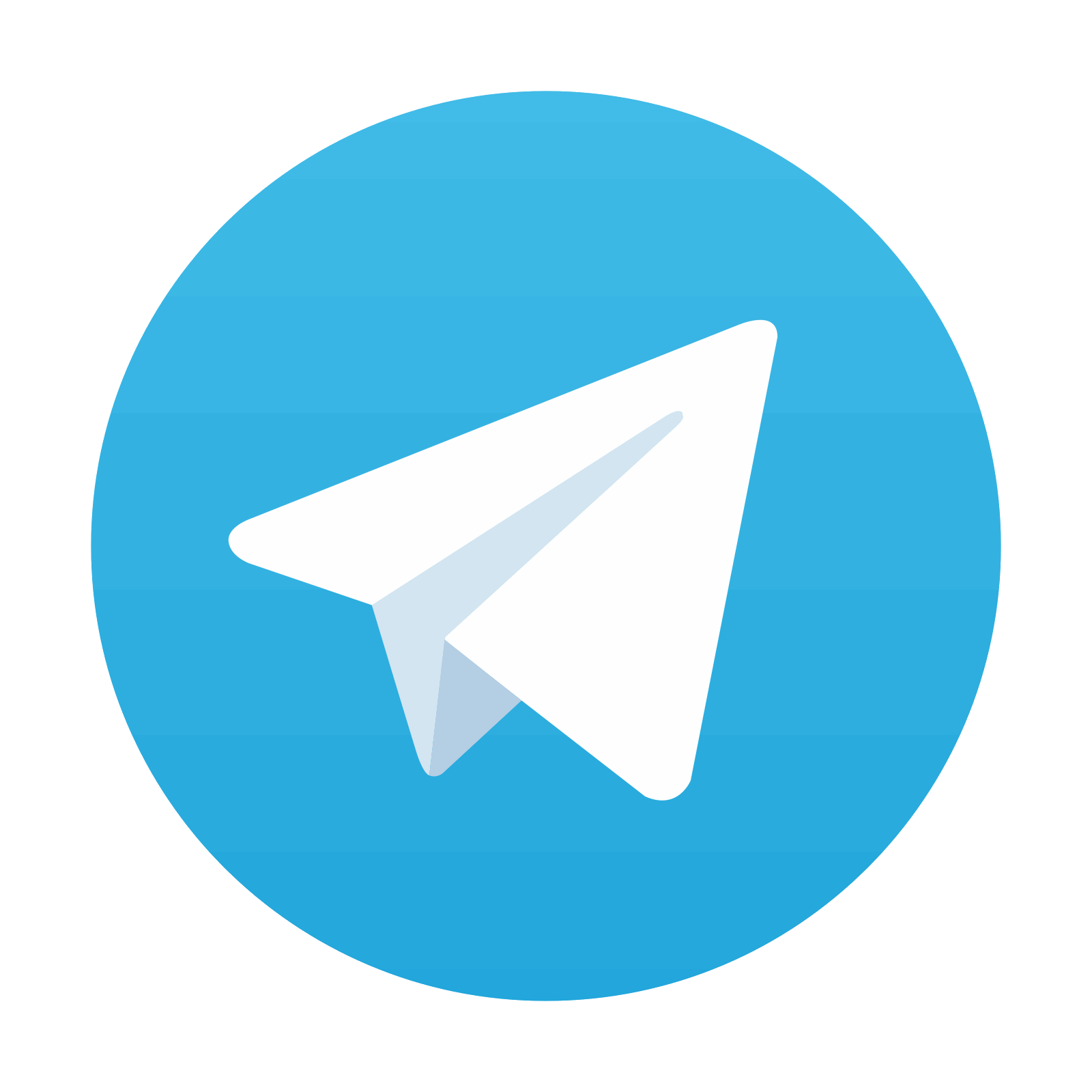
Stay updated, free dental videos. Join our Telegram channel

VIDEdental - Online dental courses
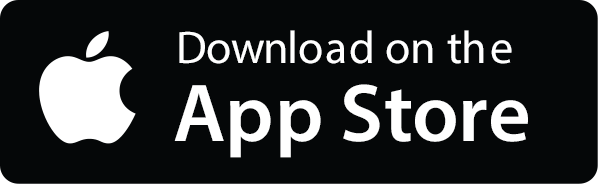
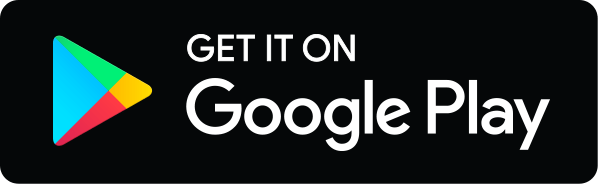