Abstract
The aim of this study was to evaluate the accuracy of three-dimensional (3D) soft tissue simulation of nose width changes following bimaxillary osteotomies and to identify patient- and surgery-related factors that may affect the accuracy of simulation. Sixty patients (mean age 26 years) who underwent bimaxillary osteotomies participated in this study. Cone beam computed tomography scans were acquired preoperatively and at 1-year postoperative. The 3D hard and soft tissue rendered preoperative and postoperative virtual head models were superimposed, after which the maxilla and mandible were segmented and aligned to the postoperative position. The postoperative changes in alar width were simulated using a mass tensor model (MTM)-based algorithm and compared with the postoperative outcome. 3D cephalometric analyses were used to quantify the simulation error. The postoperative alar width was increased by 1.6 ± 1.1 mm and the mean error between the 3D simulation and the actual postoperative alar width was 1.0 ± 0.9 mm. The predictability was not correlated to factors such as age, sex, alar cinch suture, VY closure, maxillary advancement, or a history of surgically assisted rapid maxillary expansion. The MTM-based simulation model of postoperative alar width change was found to be reasonably accurate, although there is room for further improvement.
The nasolabial region is the central aesthetic unit of the face and is considered to be one of the most important determinants of facial aesthetics. To achieve a good functional as well as aesthetic result, it is important to understand the effects that orthognathic surgery will have on the soft tissue within the nasolabial region. This is particularly the case when displacement of the maxillary complex is involved, such as in bimaxillary surgery. Various studies have reported that the alar width is subject to the greatest change postoperatively, whereas the nasal tip is the least affected in the nasolabial region. The frequently reported postoperative widening of the alar base leads to an increased soft tissue nose base width, which may be undesirable and unaesthetic. To counteract this excessive increase in alar width, different suturing methods can be applied, such as alar cinch, VY closure, and modified alar cinch.
Three-dimensional (3D) soft tissue simulation is a useful tool to preview the outcome of orthognathic surgery, for both the surgeon and the patient, especially in an era in which shared decision-making is becoming more and more important in treatment planning. Several computer-based strategies have been adopted to perform 3D virtual soft tissue simulations. Mollemans et al. found that the highest accuracy was obtained using a finite element model (FEM) or a mass tensor model (MTM). The MTM seemed to be more suitable for clinical use because of a significant time gain in simulation.
Over the years, many studies have reported the simulated hard and soft tissue changes and their relationship to orthognathic surgery. However, none of these simulation studies has focused on the nasolabial region after bimaxillary surgery, despite its prominent role in facial aesthetics. It remains unclear whether the 3D soft tissue simulation is able to predict the most important change to the nose – alar widening.
The aim of this study was to evaluate the accuracy of the 3D soft tissue simulation of changes in alar width following bimaxillary osteotomies.
Materials and methods
Patients
Patients with non-syndromic dysgnathia requiring bimaxillary osteotomies who underwent these procedures in the department of oral and maxillofacial surgery of the study medical centre in Nijmegen, Netherlands, between 2007 and 2011, were enrolled in the study. The inclusion criterion was the availability of cone beam computed tomography (CBCT) scans taken before and at least 6 months after surgery. The exclusion criteria were a previous history of bilateral sagittal split osteotomy (BSSO) or previous bimaxillary surgery, surgery other than bimaxillary osteotomy performed simultaneously, the use of a chin support during CBCT scanning, the presence of orthodontic labial appliances in the postoperative CBCT scan, the absence of upper and/or lower incisors, and extensive restorative dental treatment during the postoperative follow-up period.
Surgical procedure
All operations were performed or supervised by an experienced surgeon. After nasotracheal intubation, the mucobuccal fold of the maxilla and the mandibular ramus region was infiltrated with local anaesthetic (articaine; Ultracain DS Forte). The Le Fort I procedure started with an incision in the gingivobuccal sulcus from canine to canine. After elevation of the mucoperiosteum and nasal mucosa, the osteotomy was made with a reciprocal saw. The lateral nasal walls and nasal septum were osteotomized with nasal osteotomes. The piriform aperture and nasal spine were rounded. After mobilization of the maxilla, it was positioned in the planned position using an acrylic inter-occlusal wafer. Fixation was performed with four 1.5-mm miniplates (KLS Martin, Tuttlingen, Germany), one paranasal and one on the buttress on each side. The mucosa was closed with a 4–0 Vicryl suture (Ethicon; Johnson and Johnson Medical, Norderstedt, Germany). No alar cinch was performed for patients who were operated on before 2010, whereas all patients who were operated on from 2010 onwards received an alar cinch suture. The alar cinch suture was performed through the intraoral incision using a 2–0 Vicryl suture passing through the fibromuscular tissue of the alar base from anterior to posterior on both sides. The two free ends of the suture were tied together in the centre, restricting the width of the alar base. Following the Le Fort I osteotomy, a BSSO was performed according to the Hunsuck modification. After completion of the osteotomies, the distal segment of the mandible was placed in the planned position using the second prefabricated inter-occlusal splint and stabilized with intermaxillary fixation (IMF). The proximal segments were gently pushed backwards and upwards to seat the condyles in the glenoid fossa. The mandibular segments were fixed with two titanium miniplates (one on each side) and monocortical screws (Champy 2.0 mm; KLS Martin, Tuttlingen, Germany). After the release of IMF, the final occlusion was checked. Depending on the stability of the occlusion, the inter-occlusal splint was left in place and tight elastics were used in the first week after surgery. Class II guiding elastics were used after the first week, in conjunction with the postoperative orthodontic treatment.
Data acquisition
Preoperative and postoperative CBCT imaging data were obtained 2 weeks prior to and at least 6 months after bimaxillary surgery using a standard CBCT scanning protocol (i-CAT 3D Imaging System; Imaging Sciences International Inc., Hatfield, PA, USA) in ‘extended field’ mode (field of view 16 cm in diameter/22 cm in height; scan time 2 × 20 s; voxel size 0.4 mm). All patients were scanned while seated in a natural head position. They were asked to swallow, relax their lips and facial muscles, and to keep their eyes open. The CBCT data acquired were saved in DICOM format and exported into Maxilim software (Medicim NV, Mechelen, Belgium). A 3D virtual augmented head model was rendered in Maxilim.
Simulation of the soft tissue profile
The preoperative and postoperative 3D virtual head models were matched using voxel-based registration on an unaltered sub-volume that consisted of the cranial base, forehead, and zygomatic arches. Virtual Le Fort I and BSSO osteotomies were made on the preoperative 3D virtual head model according to the actual osteotomies performed during surgery.
In Maxilim, a soft tissue simulation was carried out based on the simulated skeletal movements using an MTM soft tissue simulation algorithm. In order to exclude discrepancies between the planned skeletal movement and the actual displacement of the bimaxillary complex at surgery, the virtually osteotomized maxilla and mandible were aligned with the position of the maxilla and mandible in the postoperative scan using surface-based registration, as described in a previous study. In this way, the simulated skeletal movements duplicated the actual jaw displacements during surgery. Thereafter the result of the soft tissue simulation could be compared with the actual postoperative soft tissue profile ( Fig. 1 ).

Analysis of the soft tissue simulation
The accuracy of the soft tissue simulation was evaluated by 3D cephalometric analysis using three soft tissue landmarks (subnasale, alare left (al l ), and alare right (al r )) and three hard tissue landmarks (upper incisor landmark ( I sup ), A point (A), and nasion). Euclidean distances were computed for all corresponding landmarks between the soft tissue simulation and the actual postoperative result as a measure of the accuracy of soft tissue simulation ( Fig. 2 ). The Euclidean distances between the corresponding landmarks (upper incisor and nasion) in the pre- and postoperative scans were also calculated, to assess the actual surgical movements.

Statistical analysis
Statistical data analyses were performed with IBM SPSS software version 21.0.1 (IBM Corp., Armonk, NY, USA). The 3D cephalometric data were used to compute the mean amount of surgical advancement and simulation error of the postoperative alar width changes, expressed in terms of the mean absolute error, standard deviation, and range.
The postoperative alar width was compared with the simulated alar width using the paired Student t -test and Pearson correlation. To identify the potential risk factors for large simulation errors and postoperative alar widening, linear regression was performed, involving factors such as age at surgery, sex, amount of surgical advancement at I sup and A point, alar cinch suture, and VY closure. Analysis of variance (ANOVA) was used to evaluate the role of previously performed surgically assisted rapid maxillary expansion (SARME) and large advancements ( I sup >4 mm) on the accuracy of the alar width simulation.
Results
The study population consisted of 60 patients, 45 females (75%) and 15 males (25%). The mean age at surgery was 26 years (range 15–58 years). The mean follow-up time was 14 months (range 6–35 months). Twenty-six patients underwent SARME prior to the bimaxillary surgery. A summary of the patient characteristics at surgery is presented in Table 1 . The alar cinch suturing technique was applied on 22 patients and VY closure was applied on 14 patients. Twelve patients had VY closure and alar cinch as the suturing technique.
Number | Mean ± SD | Range, min–max | |
---|---|---|---|
Age, years | 26 ± 9.9 | 15 to 58 | |
Sex | |||
Male | 15 | ||
Female | 45 | ||
Follow-up (months) | 14 ± 6 | 6 to 35 | |
Advancement of I sup (mm) | 2.7 ± 1.9 | −0.7 to 8.7 | |
Alar width preoperative (mm) | 34.8 ± 2.9 | 27.6 to 42.9 | |
Alar width simulation (mm) | 35.9 ± 3.1 | 29.0 to 44.6 | |
Alar width postoperative (mm) | 36.2 ± 3.1 | 30.0 to 45.1 | |
Maxillary advancement of A point (mm) a | −0.31 ± 1.98 | −5.90 to 3.70 | |
Maxillary vertical A point displacement (mm) b | 1.61 ± 1.64 | −2.30 to 5.60 |
a Maxillary advancement at A point: a positive value means that the maxilla was positioned more posteriorly than the preoperative position; a negative value means that the maxilla was positioned more anteriorly than the preoperative position.
b Maxillary vertical A point displacement: a positive value means that the maxilla was positioned more caudal compared to the preoperative position; a negative value means that the maxilla was positioned more cranial compared to the preoperative position.
Postoperative changes in the alar base
The alar width increased by a mean 1.4 mm, from 34.8 ± 2.9 mm prior to surgery to 36.2 ± 3.1 mm, and the mean absolute difference between alar width postoperative and preoperative was 1.6 ± 1.1 mm at 14 months after surgery. Linear regression analysis demonstrated that the postoperative alar width widening was positively correlated to the amount of maxillary advancement, whereas variables including age, sex, alar cinch, VY closure, and a history of SARME were not correlated with a postoperative increase in alar width ( Table 2 ). For every 1 mm of maxillary advancement, the alar width increased by 0.24 mm. A weak to moderate correlation was found between the alar width change and maxillary advancement measured at point A, as seen in Fig. 3 .
Predictor | B | (95% CI) | P -value |
---|---|---|---|
Age | 0.02 | (−0.17 to 1.69) | 0.16 |
Sex | −0.06 | (−0.65 to 0.53) | 0.83 |
Alar cinch suture | −0.06 | (−0.69 to 0.57) | 0.86 |
VY closure | −0.33 | (−1.05 to 0.40) | 0.37 |
Advancement I sup | 0.24 | (0.10 to 0.37) | <0.01 |
SARME in history | −0.45 | (−0.96 to 0.07) | 0.09 |
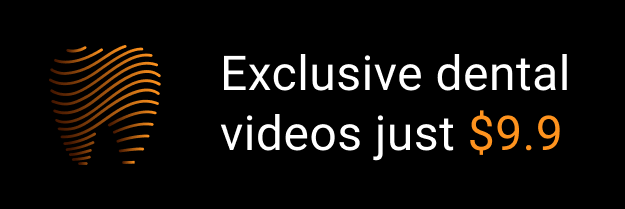