Introduction
Early Class III treatment with reverse-pull headgear generally results in maxillary skeletal protraction but is frequently also accompanied by unfavorable dentoalveolar effects. An alternative treatment with intermaxillary elastics from a temporary anchorage device might permit equivalent favorable skeletal changes without the unwanted dentoalveolar effects.
Methods
Six consecutive patients (3 boys, 3 girls; ages, 10-13 years 3 months) with Class III occlusion and maxillary deficiency were treated by using intermaxillary elastics to titanium miniplates. Cone-beam computed tomography scans taken before and after treatment were used to create 3-dimensional volumetric models that were superimposed on nongrowing structures in the anterior cranial base to determine anatomic changes during treatment.
Results
The effect of the intermaxillary elastic forces was throughout the nasomaxillary structures. All 6 patients showed improvements in the skeletal relationship, primarily through maxillary advancement with little effect on the dentoalveolar units or change in mandibular position.
Conclusions
The use of intermaxillary forces applied to temporary anchorage devices appears to be a promising treatment method.
Treatment of young Class III patients with maxillary deficiency is generally directed toward achieving positive overjet through a combination of dentoalveolar and skeletal effects. Protraction facemask therapy or reverse-pull headgear (RPHG) is perhaps the most common approach for early treatment of these patients. This approach is limited in that the forces are applied to the teeth, resulting in uncertain skeletal and often unwanted dentoalveolar effects. Even with appliance modifications to minimize tooth movement and maximize orthopedic correction, some dentoalveolar effects seem inevitable. For satisfactory clinical improvement, excellent compliance with a somewhat cumbersome extraoral appliance is required, and treatment regimens recommend wearing the appliance for 12 to 16 hours per day for 9 to 12 months.
The short-term outcomes of maxillary protraction treatment have been documented, and most investigations have described some limited orthopedic effect on the maxilla (2-3 mm of advancement on average), clockwise rotation of the mandible, and dentoalveolar changes consistent with treatment of Class III malocclusion (proclination of maxillary incisors and retroclination of mandibular incisors). Long-term follow-ups of maxillary protraction indicate a 25% to 33% chance of relapse to negative overjet after all mandibular growth is complete. Since dentoalveolar changes tend to be the most prone to relapse, it seems advantageous to minimize the dentoalveolar effects while maximizing the orthopedic correction. Furthermore, because a significant proportion of patients with maxillary deficient Class III skeletal malocclusion ultimately require orthognathic surgery, any treatment approach that could eliminate the need for, or reduce the extent of, future surgery would be of great benefit to these patients.
The use of temporary anchorage devices (TADs) in orthodontics has increased over recent years. One type of TAD, a modification of the titanium miniplate frequently used in orthognathic surgery for osteotomy or fracture fixation, has been used successfully as a skeletal anchorage device for various orthodontic applications. Most of these applications have focused on achieving dental movements, but recent case reports have demonstrated the use of TADs as an adjunct to orthopedic treatment. The advantages of absolute anchorage to aid in maxillary protraction was introduced in 1985 by Kokich et al, who applied protraction forces from a facemask to intentionally ankylosed deciduous canines serving as natural implants in a patient with maxillary deficiency. Later, Smalley et al experimented with osseointegrated Branemark-style implants for maxillary protraction in monkeys ( Macaca nemestrina ) and had dramatic skeletal results. More recent reports from Singer et al, Enacar et al, Hong et al, Kircelli and Pektas, and Kircelli et al demonstrated the potential for TADs as adjuncts to orthopedic maxillary protraction.
The purpose of this pilot study was to assess a new treatment approach for the correction of maxillary deficiency and describe the 3-dimensional (3D) skeletal and dental changes when TADs are used with intermaxillary elastics. The study required the development of a 3D analytic method to document the treatment changes for young growing patients.
Material and methods
This collaborative prospective study involved 3 centers (2 university departments of orthodontics and 1 private practice). The project was approved by a university committee for research on human subjects. This report describes the first 6 consecutive patients (3 boys, 3 girls; mean age, 11 years 8 months) undergoing the initial orthopedic phase of treatment. Criteria for participation in the study were 9-14 years of age at the start of treatment, skeletal Class III due primarily to maxillary deficiency (determined by clinical examination including profile evaluation), Class III dental occlusion determined by the permanent first molars or overjet ≤0 mm, and sufficient dental development, to avoid injury to unerupted mandibular permanent canines during surgical placement of the miniplates. All 6 patients were at prepubertal cervical vertebral maturation stages. The treatment protocol at all sites was based on the clinical experience of the orthodontist (H.J.D.) who developed this technique.
In the surgical procedure, 4 miniplates were placed in each patient—1 in each infrazygomatic buttress of the maxilla and 1 in the anterior mandible between and inferior to the left and right permanent lateral incisor and canine. Flaps were reflected in these sites, and the devices were secured by using titanium miniscrews after pilot hole preparation. The modified titanium miniplates incorporated an intraoral attachment with a locking fixation screw to allow customizable traction hooks ( Fig 1 ). In all sites, the miniplates were placed with the attachment arm exiting through attached tissue at or near the mucogingival junction. All mucoperiosteal flaps were secured with 4/0 resorbable sutures. All surgical procedures for these 6 patients were done by the same experienced oral surgeons. The surgical sites were allowed to heal for 2 to 3 weeks before orthopedic loading. The surgical procedures are well tolerated by patients.
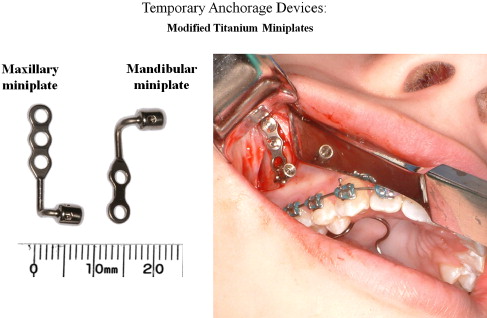
In the orthopedic protocol, the miniplates were loaded 3 weeks after surgery. One elastic was placed on each side to give vectors of force downward and forward for the maxilla and backward and upward (counterclockwise) for the mandible ( Fig 2 ). The elastics were chosen to provide an initial force of approximately 150 g to each side, increased to 200 g after 1 month of traction and to 250 g after 2 months. The forces were measured with the patient in maximum intercuspation by using a Correx force gauge (Haag-Streit, Bern, Switzerland). The patients were instructed to wear the elastics 24 hours per day. The elastics were replaced at least once a day. On the day of loading, oral hygiene instructions were reiterated with particular emphasis on brushing the tissues around the miniplates with a soft toothbrush. The mean duration of orthopedic traction to the maxilla was 12.5 months, with a range of 9 to 14 months. The decision to discontinue orthopedic treatment was made when the clinician judged that adequate positive overjet was achieved.
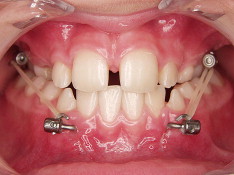
To visualize the treatment changes in 3 dimensions, cone-beam computed tomography (CBCT) scans were taken immediately after placement of the miniplates (T1), and, at approximately 1 year (T2), or the conclusion of the orthopedic treatment, whichever came first. The scans were acquired with an iCat machine (Imaging Sciences International, Hatfield, Pa) with a 40-second scan and a 16 × 22-cm field of view.
The data from each CBCT scan were saved as digital imaging and communications in medicine (DICOM) files—the standard for distributing any medical images regardless of the machine used for acquisition. Before image analysis, the data were processed by using the methods of Cevidanes et al but modified for growing patients. This process involved the following steps.
- 1.
Segmentation is the process of defining the shape of structures in each orthogonal slice of the CBCT data (axial, coronal, and sagittal) by outlining the structures in each cross section. The process was completed by using the ITK-SNAP software, a freely available open-source program. Segmentation produces a true 3D surface model, and this was created from both the T1 and T2 scans of each patient ( Fig 3 ).
Fig 3 Segmentation process: defining anatomic boundaries in all 3 planes to create 3D surface model with the open-source ITK-SNAP program. - 2.
Before superimposition of the 2 time points, the models had to be registered in a common coordinate system, because their initial orientation reflected merely how the patient was positioned in the scanner. Registration was completed with a novel sequence of fully automated voxel-wise rigid registration at the anterior cranial fossa in the Imagine 1.2.1 Pipeline Software developed at the University of North Carolina. This method, developed by Cevidanes et al, masks anatomic structures altered by treatment or growth to avoid observer-dependent reliance on subjectively defined anatomic landmarks. In this study, the initial and final 3D models were registered on anterior cranial fossa structures, specifically the endocranial surfaces of the cribiform plate region of the ethmoid bone and the frontal bone. These regions were chosen because of their early completion of growth.
- 3.
Visualization and assessment of changes were done only after registration, when the 2 models can be superimposed to assess change. The T1 and T2 registered models were cropped to yield 4 models: soft-tissue model, hard-tissue skull model (including the maxilla, mandible, and cranial base), a maxilla and mandible only model (with the cranial base cropped away), and a cranial base only model (with the maxilla and mandible cropped away). Three-dimensional surfaces were created for all models and loaded into the superimposition program, CMFApp (developed by the Institute for Surgical Technology & Biomechanics, Bern, Switzerland), which uses the iterative closest point method for posttreatment assessment. The initial and registered final models were superimposed, and the treatment changes were expressed via color maps that represent the closest-point surface distance from the final model to the initial one. Areas on the red end of the spectrum have positive mean surface-distance values and represent outward movement. Areas on the blue end of the spectrum have negative mean surface-distance values and represent inward movement. Areas that are green showed little or no change in surface distance between the initial and final models ( Fig 4 ). For additional quantitative assessment of the changes between the T1 and T2 models, the isoline tool was used. It allows the user to define a surface-distance value that is then expressed as a contour line (isoline) that corresponds to regions having a surface distance equal to or greater than the defined value. The color map allows for both visual and quantitative assessments of treatment changes.
Fig 4 Three-dimensional superimposition visualization tools. With the color map tool, areas on the red end of the spectrum have positive values of mean surface distance between the T1 and T2 models, and represent surfaces with outward movement. Areas on the blue end of the spectrum have negative values of mean surface distance between the T1 and T2 models, and represent surfaces with inward movement. Areas that are green have little or no change in surface distance between the T1 and T2 models. With the semitransparency tool, the T1 model is solid red, and the superimposed T2 model is a semitransparent gray mesh to allow qualitative assessment of change.
An alternative way of displaying the treatment change is to superimpose both 3D models, modifying the final model to be semitransparent so that the underlying initial model can be visualized ( Fig 4 ). This tool provides a qualitative assessment of the areas and magnitudes of change.
The reliability of the 3D superimpositions was tested by 3 examiners (G.C.H., M.C., L.C.), each of whom created models from T1 and T2 for the first 3 patients. Minimum surface-distance changes in several anatomic regions were compared across examiners for each patient. The values were all less than or equal to the 0.5-mm voxel resolution of the CBCT volume. That is, in each case, the differences in measurements were equal to or smaller than the resolution of the image.
The DICOM files were also used to create synthetic lateral cephalograms for both T1 and T2. These were created by using imaging software (version 10.1, Dolphin Imaging, Chatsworth, Calif), which uses an algorithm that recreates perspective (vs orthogonal) projections. The software allows the user to specify where the central ray of the imaginary x-ray beam is focused. In each case, the beam was centered on porion to most closely match the traditional cephalometric patient positioning. All cephalograms were digitally traced 3 times by the same examiner (G.C.H.) using the Dolphin program. The cephalometric measures selected were those most commonly reported in the facemask literature. The linear distances between A-point at T1 and T2 were evaluated by using the Dolphin x-y coordinate system, with sella as the coordinate center of the T1 tracing. Measurement accuracy was assessed by using intraclass correlation coefficients, which were between 0.91 and 0.98 for all measurements.
Results
Each patient was evaluated with focus on the following anatomic regions: (1) anterior surface of the maxilla in the region encompassing A-point, (2) zygomatic processes of the maxilla, (3) anterior mandible in the region of pogonion, (4) anterior and posterior surfaces of the condyles, (5) inferior borders of the mandible, and (6) glenoid fossae. The skeletal changes between T1 and T2 in the areas of interest are shown as color maps or semitransparencies in Figures 5 through 9 .
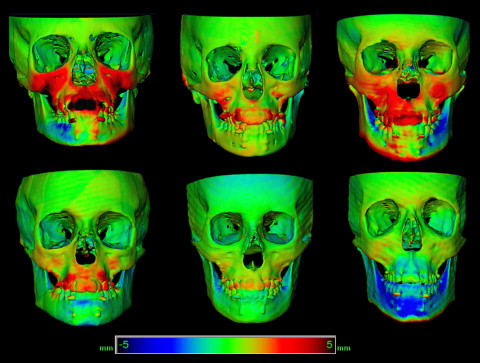
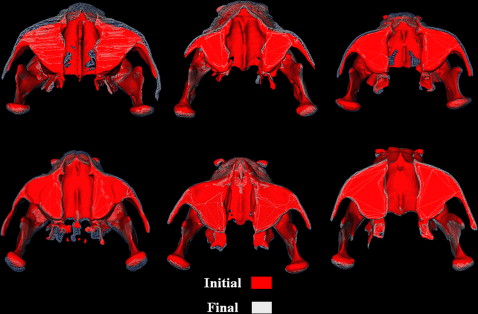
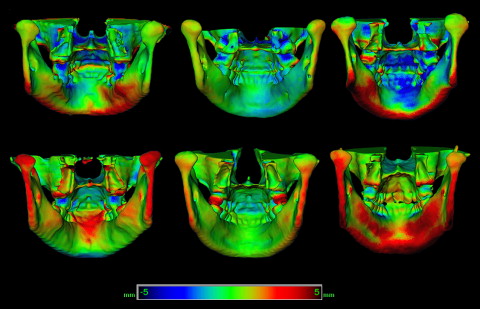
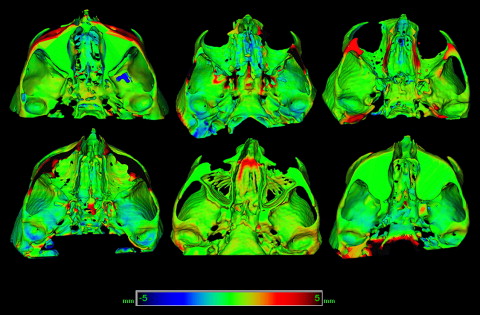
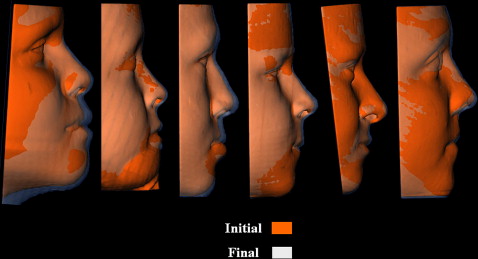
Figures 5 and 6 show the skeletal changes between T1 and T2 in the maxilla as color maps and semitransparencies. All patients showed a positive (outward) movement in the zygomatic process region, and all but 1 patient showed a positive change in the anterior maxillary region. The maxillary teeth also moved forward but to a variable extent. Overjet was positive in all patients at T2 but was not necessarily overcorrected.
The changes in the anterior mandibular region were more variable in both magnitude and direction ( Fig 5 ). There were small individual differences in surface distances between the left and right condyles, but all patients showed a positive change on the posterior surfaces and a negative (inward) change on the anterior surfaces ( Figs 6 and 7 ). The inferior border of the mandible showed positive change for all patients ( Fig 7 ).
With the maxilla and mandible cropped away, the inferior surface of the cranial base, including the glenoid fossae, can be visualized. Three patients showed little change in the glenoid fossae, but 3 showed slight negative changes on the posterior and superior surfaces, and 1 showed a slight positive change on the anterior surfaces ( Fig 8 ).
The soft tissues in the upper lip and nasal regions showed positive changes for all patients but with considerable variations in magnitude ( Fig 9 ).
The synthetic cephalograms were used to provide comparisons to existing databases of skeletal and dental changes. Because of the small sample size, individual, rather than aggregated, changes are given in Tables I and II . SNA increased in 5 of the 6 patients, and ANB increased in all patients. The changes in SNB and mandibular plane angle (SN-Go-Gn) showed greater variations ( Table II ). The maxillary incisor angulation, assessed by the U1-SN angle, increased in 3 patients and decreased in 3 patients. Mandibular incisor angulation assessed by the IMPA increased in all patients ( Table II ). In every patient, there was forward horizontal movement of A-point ( Table II ).
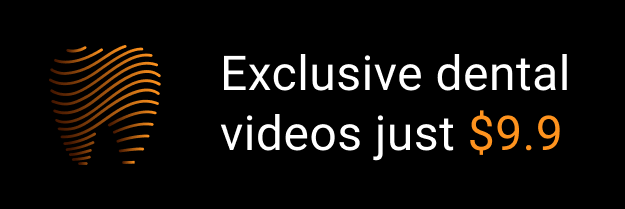