Abstract
Objective
To explore experimental RBCs derived from a successful commercially available RBC (Grandio) to investigate resin monomer blend and filler parameters (volume fraction, density and diameter) on RBC performance.
Method
Six experimental RBCs modified from a commercial RBC were tested. The three-point flexure strength ( σ 3 ) and modulus ( E ) data was determined for groups of 20 bar-shaped specimens, prepared in a custom-made knife-edge split aluminum mold and irradiated using a modification of the ISO 4049 protocol. The biaxial flexure strength (BFS) and top and bottom Vickers hardness number (VHN) determination was performed on disc-shaped specimens ( n = 20). Normal distribution of the σ 3 , E , BFS and top and bottom VHN data was verified using the Shapiro–Wilk’s test. Paired groups were compared using independent samples t -test for the individual tests investigated ( σ 3 , E , BFS and top and bottom VHN) at a significance level of P < 0.05.
Results
A significant decrease in the mean σ 3 ( P < 0.011) and mean E ( P < 0.001) were identified on increasing the filler fraction (from 71.4 to 74.5 vol%) or increasing the mean filler diameter (from 1.5 to 2.5 μm). Increasing the filler density resulted in a significant increase in the mean σ 3 ( P < 0.001), mean E ( P < 0.001) and mean top VHN ( P ≤ 0.001). Replacing the monomeric blend with an ormocer blend significantly reduced the mean σ 3 , E , BFS and top and bottom VHNs (all P < 0.001).
Significance
For each RBC monomeric resin and filler (type, density and diameter) combination employed, a finite filler volume fraction exists. Operating at the finite filler volume fraction increases the difficulty in improving the mechanical properties of the RBCs tailored from a commercial product.
1
Introduction
Cost-effective and predictable dental treatment is central to the achievement of patient satisfaction regardless of whether the treatment is paid privately by the patient or provided by a third party such as the General Dental Services (GDS) in England and Wales . In a systematic review of restoration longevity from 195 randomized clinical trials, quasi-experimental designs and non-experimental studies , dental amalgam was identified to be the material of choice due to the durability of the silver–tin alloy composition in the oral environment . Recent prospective clinical studies have shown the performance of dental amalgam to be superior to comparable with and inferior to resin-based composites (RBCs). Variations between studies were a result of differences in study population (primary or permanent teeth), restoration size (small or extensive), patient caries risk (low or high), sample size and study location . A representative sample of over 80,000 adult GDS patients treated between 1991 and 2001 was recently reported and items gleaned from 719,009 payment claims submitted by GDS dentists to the Dental Practice Board (DPB) in Eastbourne, England were examined . The results confirmed the view that dental amalgam was the material of choice with single surface (mesio-occlusal or distal-occlusal) dental amalgam restorations having a survival probability of 58% after ten years and a median survival time of over eleven years . A limitation of the study was that it was not possible to compare the performance of dental amalgam and RBCs as the GDS regulations preclude the use of RBCs in load-bearing surfaces of posterior teeth . Accordingly, the RBCs were placed in Class III, IV and V cavities with an associated survival probability at ten years of 43% . It is suggested that the amalgamated results for the 147,087 mesio-occlusal or distal-occlusal dental amalgam and 127,375 RBC restorations reported for practitioners with a range of experiences provide a more realistic overview of how restorations perform in the ‘real-world’ of clinical dentistry than the previously reported studies .
The introduction of a novel RBC composed of bisphenol-A and glycidyl methacrylate (BisGMA) resin and quartz or aluminosilicate glass filler in 1958 revolutionized restorative dentistry . However, the necessity for the admixture of a lower molecular weight monomer, triethyleneglycol dimethacrylate (TEGDMA), was required for a successful RBC owing to the unsuitability of BisGMA to allow for the incorporation of large filler volume fractions . Additionally, the glass fillers were coated with a silane coupling agent to provide an adhesive bond between the powdered inorganic filler and the resin matrix . The mechanical and physical properties of the difunctional BisGMA monomer were reported to be superior to methylmethacrylates because of the larger molecular size and the rigid aromatic backbone and the capability of forming stable hydrogen bonds through its hydroxyl group , giving rise to the development of the first generation of commercially available RBCs. Today, BisGMA is employed almost extensively in dental RBC matrix formulations.
Since Bowens’ original RBC formulation, the development of RBCs has been considerable with variations in monomeric resin formulations and filler constituents at the forefront of RBC research. Today, restorative dentistry boasts low-shrink non-methacrylate monomeric resin formulations and filler technologies that encompass nanotechnology . Much of the experimentation on RBCs has been performed by materials scientists using dental products or self-manufactured RBCs . The general ‘rules of thumb’ for RBCs, identified through experimentation, dictate that increasing filler volume fraction increases the mechanical properties , decreasing filler particle size reduces the filler volume fraction allowable and the filler is dominant over the resin in terms of mechanical properties . In the current study an alternative approach was undertaken to investigate structural parameters and determine RBC performance by exploring experimental RBCs provided by a dental manufacturer .
The interpretation of standard mechanical property test results for RBC materials is complex and a function of the discriminatory power of the testing methodology employed. The three-point and biaxial flexure strength tests employ distinctive specimen geometries (bar- and disc-shaped, respectively) and therefore the RBC is subjected to stress distributions characteristic to each testing methodology prior to and during fracture. The bar-shaped specimens are subjected to flexural loading in the three-point flexure strength test and the modulus of rupture is calculated from the load to failure using simple beam theory . The major advantage is that a state of pure tension is established on the bottom surface of the specimen under test and the modulus of rupture may be equated to the tensile strength of the material because fracture initiates on the bottom surface of the brittle specimen in tension and no plastic yielding occurs . However, the stress in the loaded section is not uniform varying from a maximum compressive stress at the top surface, to zero at the neutral plane, along the length of the specimen, and to a maximum tensile stress at the bottom surface which accentuates the effect of surface condition on the measured three-point flexure strength . As a result, the reported three-point flexure strengths are generally in excess of the true tensile strength of the material .
The biaxial flexure strength testing methodology centrally loads a disc with a spherical ball indentor on a knife-edge ring support so that the maximum tensile stresses occur beneath the indentor at the center of the specimen and decrease rapidly with increased radial distance from the center of the disc . The major advantage of using the biaxial flexure strength test is that spurious edge failures routinely associated with three-point flexure strength tests are eliminated . A further advantage is the test offers controllable specimen geometry and simple sample preparation in a ‘one-hit’ irradiation compared with the complexity of the overlapping irradiation protocol specified for bar-shaped specimens . The considerable disparity in the stress distributions under loading and the distinct specimen fabrication procedures for the two strength tests employed suggest unique results for each methodological approach undertaken.
The three-point flexural modulus is advantageous over the flexural strength since the three-point flexural modulus is an intrinsic material property which can be linked directly to the bonding between atoms and does not change as a function of specimen volume (Eq. (2) ) as strength does . Previously, the flexural modulus was shown to be beneficial in highlighting significant differences for glass-ionomer materials undergoing minor compositional variations which were not observed in the strength data . The Vickers hardness number (VHN) provides the operator with an indication of the hardness of the top and bottom surfaces of a RBC following irradiation. A generalization that a bottom to top VHN of 80–90% is clinically acceptable intuitively makes little sense as the VHN is RBC material specific depending upon the chemistry and extent of polymerization of the matrix , the filler volume fraction, density and particle size and distribution , notwithstanding the interfacial properties mediated through the silane coupling agent between the filler and resin matrix . Additionally, the VHN is dependent on the experimental design which is intrinsically unbalanced in respect the randomness of the location of the indentation measurement (resin or filler rich area) .
The aim of the current study was to explore experimental RBCs derived from a successful commercially available RBC (Grandio, Voco GmbH, Cuxhaven, Germany) to investigate the influence of monomeric resin and filler parameters on RBC performance in terms of three-point and biaxial flexure strength, three-point flexural modulus and VHN.
2
Materials and methods
2.1
Materials
Experimental RBCs ( Table 1 ) based around the Grandio RBC formulation which consists of a BisGMA:TEGDMA monomeric blend (3:1) with a 71.4 vol% filler volume fraction of 2.4 g/cm 3 density barium–alumina borosilicate particles (1.5 μm mean diameter) were produced by the manufacturer (Voco GmbH, Cuxhaven, Germany). To investigate the influence of filler volume fraction, the Grandio RBC formulation was modified (experimental RBC G 1 ) by increasing the filler fraction from 71.4 to 74.5 vol% while maintaining the BisGMA:TEGDMA ratio, the filler density and the mean filler diameter. The BisGMA:TEGDMA monomer blend of the experimental RBC G 1 was replaced by a UDMA:aliphatic dimethacrylates (1:1) monomeric blend while maintaining the filler characteristics (mean diameter, density and volume fraction) to produce the experimental RBC G 2 .
Resin-based composite | Resin matrix | Barium–alumina borosilicate filler particles | ||||
---|---|---|---|---|---|---|
Resin composition | Ratio | Density (g/cm 3 ) | Diameter (μm) | Fraction weight % | Fraction volume % | |
Grandio | BisGMA:TEGDMA | 3:1 | 2.4 | 1.5 | 87 | 71.4 |
G 1 | BisGMA:TEGDMA | 3:1 | 2.4 | 1.5 | 89 | 74.5 |
G 2 | UDMA:aliphatic dimethacrylates | 1:1 | 2.4 | 1.5 | 89 | 74.5 |
G 3 | BisGMA:TEGDMA | 3:1 | 2.4 | 2.5 | 91 | 76 |
G 4 | BisGMA:TEGDMA | 3:1 | 2.7 | 2.5 | 89 | 71 |
G 5 | BisGMA:TEGDMA | 3.5:1 | 2.4 | 2.5 | 87 | 73 |
G 6 | Ormocer | 1 | 2.4 | 2.5 | 87 | 73 |
The Grandio RBC formulation was altered by increasing the mean filler diameter from 1.5 to 2.5 μm and the filler fraction from 71.4 to 76 vol% (experimental RBC G 3 ), with the BisGMA:TEGDMA ratio and filler density maintained, to investigate the influence of filler diameter. To investigate the influence of filler density, the BisGMA:TEGDMA monomer blend and mean filler diameter of experimental RBC G 3 was maintained and barium–alumina borosilicate particles with a density of 2.7 g/cm 3 (rather than 2.4 g/cm 3 ) were used (experimental RBC G 4 ) with the filler fraction reduced from 76 to 71 vol%. The 3:1 (BisGMA:TEGDMA) ratio of the experimental RBC G 3 was modified to a 3.5:1 ratio and the filler fraction decreased from 76 to 73 vol% (experimental RBC G 5 ) to investigate the influence of BisGMA:TEGDMA monomer blend ratio. The influence of monomer blend was investigated by replacing the BisGMA:TEGDMA monomeric blend of experimental RBC G 5 with an ormocer matrix (experimental RBC G 6 ), while maintaining the filler diameter, filler density and filler fraction ( Table 1 ).
2.2
Three-point flexure
Twenty rectangular bar-shaped specimens (length 25.0 ± 0.1 mm, height 2.0 ± 0.1 mm and thickness 2.0 ± 0.1 mm) were prepared for Grandio and the experimental RBCs (G 1 –G 6 ) by packing an excess of uncured RBC into a custom-made knife-edge split aluminum (alloy 6061) mold . The split aluminum mold consisted of open ends allowing for the expulsion of excess uncured material and minimized air entrapment on specimen fabrication . The RBCs were condensed against cellulose acetate strips prior to the application of a 1 kg load for 20 s to ensure standardized packing and a consistent surface profile. The specimens were polymerized using a hand-held light curing unit (LCU) (Optilux 501, Kerr, Orange, CA, USA) fitted with a 13 mm light tip diameter that was placed in direct contact with the cellulose acetate strips. The output intensity of the LCU operating with the 13 mm light tip diameter was 620 ± 16 mW/cm 2 when placed in direct contact with the radiometer integrated within the LCU.
The length of the rectangular-shaped specimens was irradiated in 20 s exposures using a modification of the ISO 4049 protocol . Initially, the center of the rectangular specimen was irradiated for 20 s, the light tip diameter was then moved along the specimen by three-quarters (of the 13 mm light tip diameter) and both the left and right portions of the specimens were irradiated for 20 s each. After irradiation, the specimens were carefully removed from the split-mold, checked for visible surface irregularities and stored under light-proof conditions in distilled water for 24 h at 37 ± 1 °C.
A three-point flexural test was conducted using a universal testing machine (Instron Model 5565, High Wycombe, Buckinghamshire, UK) with a 5 kN load cell. The specimens were placed with the irradiated side uppermost and loaded to failure at a crosshead speed of 1 mm/min. The three-point flexure stress ( σ 3 (MPa)) and modulus ( E (GPa)) for Grandio and the six experimental RBCs (G 1 -G 6 ) were calculated according to Eqs. (1) and (2) , respectively.
σ 3 = F max 3 l 2 b h 2
E = F max l 3 4 h 3 b f
where F max was the fracture force (N), l the support span distance (20 mm), b and h the specimen thickness and height (mm) and f the extension during loading. The thickness and height of each specimen was determined at the point of fracture using a micrometer screw gage (Mitutoyo, Kawasaki, Japan) with an accuracy of 1 μm.
2.3
Biaxial flexure
Twenty disc-shaped specimens (diameter 13.0 ± 0.1 mm, thickness 1.0 ± 0.1 mm) were prepared for each RBC (Grandio and G 1 –G 6 ) by packing an excess of uncured RBC into a commercially available nylon washer. The RBC was condensed against acetate strips, placed on the top and bottom surfaces of the washer, by centrally applying a load of 1 kg for 20 s. The discs were irradiated using the Optilux 501 LCU and 13 mm light tip diameter in ‘one-hit’ by placing the light tip in direct contact with the acetate for 20 s. The irradiated specimens were carefully removed from the nylon washers, checked for visible surface irregularities, and stored under light-proof conditions in distilled water for 24 h at 37 ± 1 °C.
A thin sheet of rubber dam was placed on a 10 mm diameter knife-edge support to accommodate slight differences in the geometry of the RBC discs. The biaxial flexure stress (BFS) was determined using a universal testing machine to apply a central load through a 4 mm ball-shaped indenter at a crosshead speed of 1 mm/min. The BFS (MPa) was calculated from the load at fracture ( P ) in Newtons from Eq. (3)
BFS = P h 2 ( 1 + υ ) 0.485 ln a h + 0.52 + 0.48
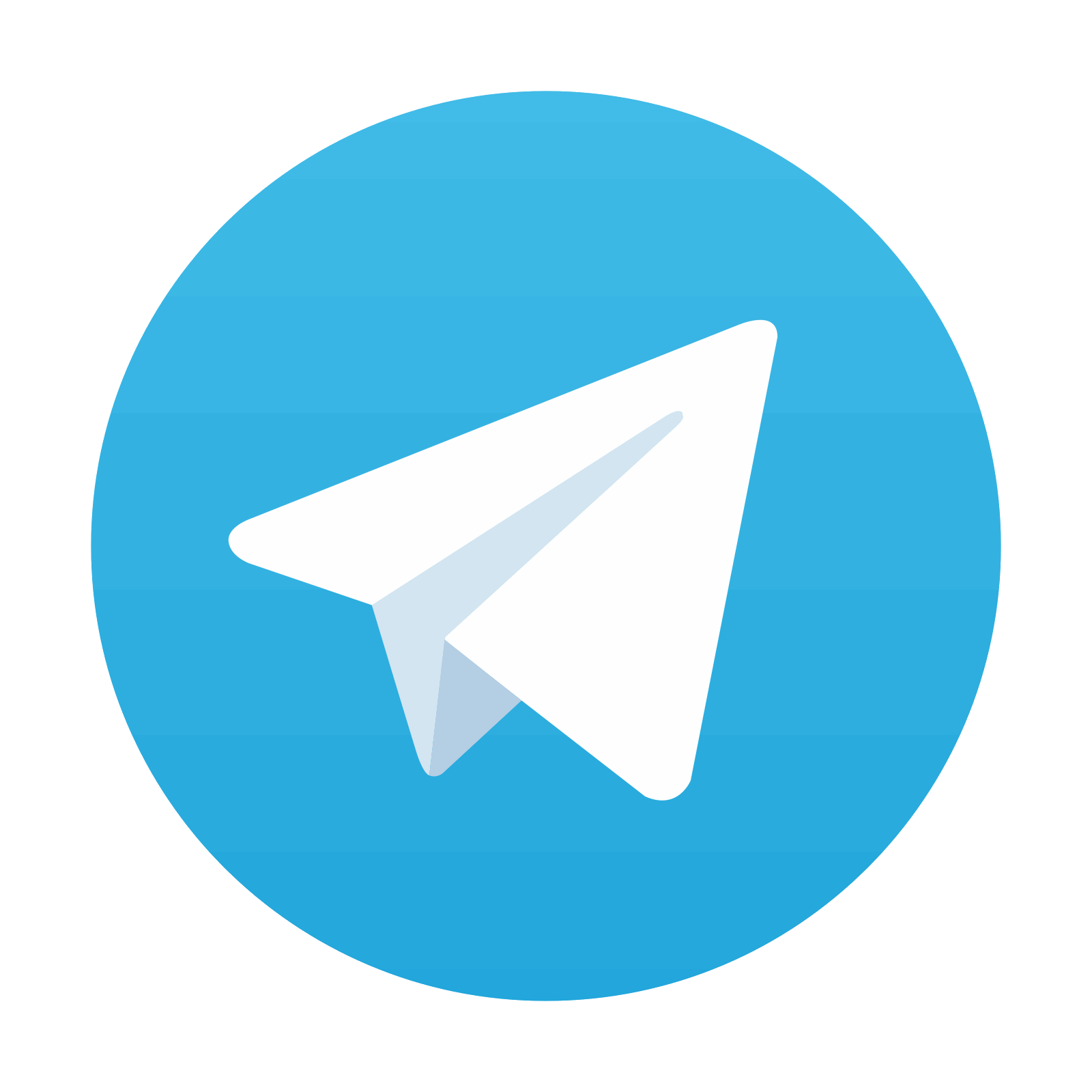
Stay updated, free dental videos. Join our Telegram channel

VIDEdental - Online dental courses
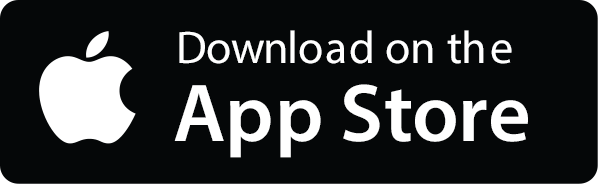
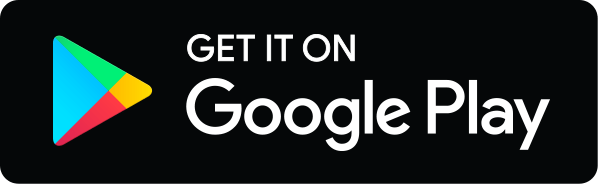