Abstract
Objectives
In this study five novel dimethacrylates of different chain lengths having rigid aromatic rings were synthesized and proposed as possible dental monomers for dental resin mixtures.
Methods
Four monomers were prepared by the reaction of glycidyl methacrylate with dicarboxylic acid esters obtained from phthalic anhydride and 1,3-propylene, 1,4-butylene, 1,5-penthylene and 1,6-hexylene glycols. The addition reaction of glycidyl methacrylate and the acidic compound was carried out in the presence of tetraethylammonium bromide. The fifth monomer was obtained from 1,5-penthylene glycol-based dimethacrylate by blocking its hydroxyl groups with acetyl groups. The monomers were photo-copolymerized with triethyleneglycol dimethacrylate (TEGDMA) in the presence of a photoinitiator which was 2,2-dimethoxy-2-phenyloacetophenone. Unfilled polymers were evaluated for photopolymerization conversion and volumetric curing shrinkage. Water sorption, water solubility, flexural strength and hardness were measured. The prepared polymers were also subjected to dynamic mechanical studies (DMA).
Results
Results show that, increasing the distance between double bonds decreases flexural modulus and hardness. As expected, the curing shrinkage increased with increasing degree of conversion. The acetylation of hydroxyl groups resulted in improved water uptake properties of the compositions. It was demonstrated that, depending upon the content of acetyl groups in the network, the glass transition temperature may be significantly lowered. In polymers with large amounts of pendant groups, β relaxation overlaps with α relaxation (glass transition temperature), and it is the former that discloses the maximum on the tan δ curve.
Significance
The systematic change in the length of the new dimethacrylates provided insight into the effects on the resultant material properties. It was shown in the article that the properties of the new monomers compare favorably with properties of the commercially available resins.
1
Introduction
The photopolymerization of multifunctional methacrylates and acrylates to form densely crosslinked networks finds wide application in dentistry such as dental composites, dental adhesives, bonding agents and cements, pit and fissure sealants .
Typical dental composites consist of dimethacrylate/diacrylate organic systems in which inorganic filling particles are imbedded. As the main component of the organic phase 2,2-bis[4-(2′-hydroxy-3′-methacryloxypropoxy)phenyl]-propane (Bis-GMA) is commonly used. Its rigid aromatic backbone structure provides superior toughness and low polymerization shrinkage of the dentin formulations although it is not without disadvantages. Bis-GMA is a highly viscous monomer and requires dilution. When polymerized in the bulk, it exhibits low degree of double bond conversion due to restriction in segmental motion . Besides, unreacted monomer species are present in the polymer matrix . Incomplete monomer conversion affects the mechanical properties of ultimate restoration whereas the elution of components from the polymer matrix worsens its biocompatibility . Diluting Bis-GMA with low viscosity alkoxyalkyl dimethacrylate esters such as triethyleneglycol dimethacrylate (TEGDMA) enables adequate filling loading and improves handling characteristics . However, it also increases water uptake and polymerization shrinkage and as a consequence deteriorates marginal adaptation of a composite .
Several approaches to increasing conversion of double bonds and thus biocompatibility and mechanical strength of dental dimethacrylates have been reported . Partial improvement of performance of dental compositions has been achieved by incorporating other low viscosity dimethacrylate monomers like 1,6-bis(2′-methacryloxyethoxycarbonylamino)-2,4,4-trimethylhexane (UDMA) or hydroxyl free analogs of Bis-GMA . Recently, incorporating thiol-ene-based monomers into compositions with conventional dimethacrylates (and thus changing the chain growth mechanism of polymerization to a step growth one) has been found to increase the overall conversion without compromising other properties like volumetric shrinkage or water sorption . Also, employing dimethacrylates and urethane dimethacrylates with high molecular weights is an alternative .
The aim of this work is to investigate the influence of the chemical structure of dimethacrylate esters of different chain lengths on the degree of conversion, polymerization shrinkage and mechanical strength of dental compositions.
Carrying out the experiments, four new hydroxyl-containing monomers of similar chemical origin to Bis-GMA as well as one hydroxyl-free in which the reactive methacrylate groups are relatively far apart were synthesized and identified. An increased flexibility of the monomers in respect to Bis-GMA has been achieved by incorporating a flexible hydrocarbon chain between the aromatic rings in the middle of their structures. The new monomers were photo-copolymerized with TEGDMA under the same conditions in order to obtain comparable results. The properties of new compositions were compared with those of Bis-GMA/TEGDMA analog preparation. Also, acetyloxypropylene dimethacrylate (Acet-GDMA) was evaluated as a dental diluent.
2
Materials and methods
2.1
Materials and instruments
Triethyleneglycol dimethacrylate (TEGDMA, 94%, from Merck KgaA Frankfurt), glycidyl methacrylate (GMA, 97%, from Sigma–Aldrich Chemie GmbH), tetraethylammonium bromide (analytically pure, from Merck KgaA Frankfurt), phthalic anhydride (analytically pure, from Merck KgaA Frankfurt), 1,3-propylene, 1,4-butylene, 1,5-penthylene and 1,6-hexylene glycols (99%, from Sigma–Aldrich Chemie GmbH), hydroquinone (analytically pure, from Merck KgaA Frankfurt), 2,2-dimethoxy-2-phenyloacetophenone (Irgacure 651, UV polymerization initiator, from Ciba Specialty Chemicals), sulfuric acid (98%, from POCh Gliwice), acetic anhydride (analytically pure, from POCh Gliwice), ethyl acetate (analytically pure, from POCh Gliwice), anhydrous magnesium sulfate (analytically pure, from POCh Gliwice), anhydrous calcium chloride (analytically pure, from POCh Gliwice), sodium hydrogen carbonate (analytically pure, from POCh Gliwice), and zinc chloride (analytically pure, from POCh Gliwice) were used as received. Acetyloxypropylene dimethacrylate (a mixture of isomers) was prepared in my laboratory. The methodology is described elsewhere .
1 H NMR spectra of purified methacrylate monomers were recorded at 20 °C using an FT-NMR Bruker Avance (Germany) spectrometer operating at the 1 H resonance frequency of 300 MHz. Chemical shifts were referenced to tetramethyl silane serving as an internal standard. FTIR spectra were recorded by using an FTIR spectrophotometer (Perkin-Elmer 1725X). A small drop of each sample containing the photoinitiator—2,2′-dimethoxy-2-phenyloacetophenone (Irgacure 651) was analyzed after being placed on a KBr crystal. UV irradiated and postcured polymeric samples were powdered and analyzed in the form of KBr pellets. Elementary analysis was carried out using the Perkin Elmer CHN 2400 apparatus. An empty aluminum pan was used as a reference. Viscosities of the methacrylate monomers and resins were measured by means of a rotating spindle rheometer (Brookfield, model DV-III) using appropriate spindles and standard solutions. Viscosity was measured at various spindle speeds (5–150 rpm) and only readings obtained around 50% torque were recorded and expressed as Pa s. Refractive indices were evaluated at 20 °C by means of a Carl Zeiss Jena refractometer according to PN-88/C-89082/06 specification. pH level was measured by a basic titration method (PN-87/C-89082/11 specification).
The effect of temperature on viscoelastic properties of the obtained polymers in the area of linear dependence between stress and strain was determined using a DMA Q800 apparatus produced by TA (USA) calibrated according to the producer’s recommendation. Thermomechanical properties of the cured polymers were evaluated from storage modulus ( E ′) and tan δ curves obtained at constant frequency (10 Hz). Measurements for all samples were made in the temperature range 0–200/250 °C at a constant heating rate of 4 °C/min. Rectangular profiles of the sizes: (2 ± 0.2 mm) × (12 ± 0.2 mm) × (35 mm) were used in the measurements. Flexural properties were determined in a three-point loading configuration using a Zwick Roell Strength Machine (model Z010). Specimen dimensions were: (10 ± 0.2 mm) × (4 ± 0.2 mm) × (75 mm). Measurements were carried out at room temperature with a crosshead speed of 5 mm/min. At least six specimens were tested for every datum point (PN-82/C-89051). Hardness according to Brinell was determined by means of a hardness tester HPK (PN-84/C-89030).
2.2
Syntheses of dimethacrylates
Syntheses of dimethacrylate esters were carried out in two stages. The general procedure is described for 1,3-bis[(2′-hydroxy-3′-methacryloxypropoxy)-phthaloxy]-propane (PhA-13).
Stage I—the synthesis of phthalic anhydride and 1,3-propylene glycol adduct. The addition was carried out in a three-necked round-bottomed 500 mL flask equipped with a heating jacket, stirrer, thermometer and air cooler. The reagents used at the ratio of 1 mole of ethylene glycol and 2 mole of phthalic anhydride were heated up to 120 °C. The mixture was then kept at 125 °C for 2 h. The reaction was controlled by estimation of the PH level. Stage I ended when the pH level dropped twice from its start value of 602 mg KOH/g to 300 mg KOH/g.
Stage II—the reaction of glycidyl methacrylate with acidic ester prepared in stage I. The acidic ester obtained in stage I was cooled down to 80 °C and glycidyl methacrylate in the amount corresponding to the determined pH level was added to the flask. The synthesis was carried out in the presence of tetraethylammonium bromide (0.1 wt.%) as a catalyst and hydroquinone (0.01 wt.%) as an inhibitor of radical polymerization at 105–115 °C for about 6 h. Stage II ended when the pH level decreased below 5 mg KOH/g. The same method was utilized to prepare 1,4-bis[(2′-hydroxy-3′-methacryloxypropoxy)-phthaloxy]-butane (PhA-14), 1,5-bis[-(2′-hydroxy-3′-methacryloxypropoxy)-phthaloxy]-pentane (PhA-15) and 1,6-bis[-(2′-hydroxy-3′-methacryloxypropoxy)-phthaloxy]-hexane (PhA-16).
The acetylated dimethacrylate 1,5-bis[-(2′-acetoxy-3′-methacryloxypropoxy)-phthaloxy]-pentane (Acet-PhA-15) was obtained by reacting acetic anhydride with PhA-15. To a mixture of PhA-15 (60 g, 0.087 m) and acetic anhydride (18 g, 0.176 m), sulfuric acid (5 drops, 0.05 g) was added with stirring at room temperature. The temperature was then kept at 75–80 °C for 3 h. After cooling the mixture was washed with distilled water. Then it was dissolved in ethyl acetate and washed repeatedly with 5 wt.% of aqueous NaHCO 3 , and distilled water, and then dried over anhydrous magnesium sulfate. After filtration, the solvent was removed under reduced pressure (1–2 mmHg) at 60 °C. A yellowish liquid was obtained (43 g, 64%).
Fig. 1 shows the structures of all monomers used in this study. The purified products were identified using both FTIR and 1 H NMR. FTIR (PhA-13, PhA-14, PhA-15 and PhA-16): 3490 cm −1 (O–H), 2930 cm −1 (C–H), 1721 cm −1 (C O), 1637 cm −1 (C C), 1607 cm −1 (aromatic rings), 1050 cm −1 (C–O), 840 cm −1 (C C). FTIR (Acet-PhA-15): 2930 cm −1 (C–H), 1721 cm −1 (C O), 1637 cm −1 (C C), 1607 cm −1 (aromatic rings), 1050 cm −1 (C–O), 840 cm −1 (C C). 1 H NMR: (CDCl 3 ): PhA-13: δ 2.2 (2H), 4.2 (4H), 1.9 (6H), 3.8, 4.3, 4.5 (10H), 5.6, 6.2 (4H), 7.5, 7.7 (8H—aromatic rings); PhA-14: δ 1.8 (4H), 4.2 (4H), 1.9 (6H), 3.8, 4.3, 4.5 (10H), 5.6, 6.2 (4H), 7.5, 7.7 (8H—aromatic rings); PhA-15: δ 1.5 (2H), 1.8 (4H), 4.2 (4H), 1.9 (6H), 3.8, 4.3, 4.5 (10H), 5.6, 6.2 (4H), 7.5, 7.7 (8H—aromatic rings); Acet-PhA-15: δ 1.5 (2H), 1.8 (4H), 2.0 (6H), 4.3 (4H), 1.9 (6H), 5.3, 4.4, 4.5 (10H), 5.6, 6.2 (4H), 7.5, 7.7 (8H—aromatic rings); PhA-16: δ 1.5 (4H), 1.8 (4H), 4.2 (4H), 1.9 (6H), 3.8, 4.3, 4.5 (10H), 5.6, 6.2 (4H), 7.5, 7.7 (8H—aromatic rings).

CHN analysis of the obtained dimethacrylate esters is as follows. The theoretical composition of dimethacrylate ester PhA-13: %C: 60.36; %H: 5.53; %O: 34.11; the determined composition: %C: 60.21; %H: 5.67; %O: 34.12. The theoretical composition of dimethacrylate ester PhA-14: %C: 60.89; %H: 5.71; %O: 33.40; the determined composition: %C: 60.68; %H: 5.82; %O: 33.50. The theoretical composition of dimethacrylate ester PhA-15: %C: 61.40; %H: 5.89; %O: 32.71; the determined composition: %C: 60.98; %H: 5.83; %O: 33.19. The theoretical composition of acetylated dimethacrylate Acet-PhA-15: %C: 60.93; %H: 5.77; %O: 33.30; the determined composition: %C: 60.99; %H: 5.48; %O: 33.53. The theoretical composition of dimethacrylate ester PhA-16: %C: 61.88; %H: 6.06; %O: 32.06; the determined composition: %C: 61.85; %H: 6.15; %O: 32.00.
2.3
Curing procedure
All new dimethacrylates as well as Bis-GMA were dissolved in TEGDMA making 50/50 wt.% solutions. Additionally, a 50/50 wt.% composition of PhA-15 and Acet-GDMA was prepared. Then, the compositions were irradiated by an ultraviolet light (340–360 nm) in the presence of a photoinitiator (Irgacure 651) used in the amount of 1 wt.%. Photopolymerization was carried out in specially designed glass molds and initiated with four TL20W/05 SLV low-pressure mercury lamps at 25 °C. The compositions in the molds were irradiated from both sides for 10 min. The obtained copolymers were subsequently postcured at 120 °C for 2 h. The same procedure was utilized to prepare the PhA-15 homopolymer.
2.4
Measurement of double bond conversion
The photopolymerization conversion (DC) of the compositions was measured by FTIR spectroscopy. Photocurable formulations containing 1 wt.% Irgacure 651 as a photoinitiator were prepared. To find the conversion degree, intensities of peaks responsible for stretching vibrations of C C (1637 cm −1 ) and C O (1720 cm −1 ) groups before and after polymerization were compared. The DC of the analyzed formulations was calculated by the following equation:
DC ( % ) = 100 × 1 − R polymer R monomer ;
where R is the surface area of C C absorption band/surface area of C O absorption band. Three trials were conducted for each composition.
2.5
Measurement of polymerization shrinkage
The volume shrinkage of photopolymerized samples was determined by the following relation:
Polymerization shrinkage ( % ) = d polymer − d monomer d polymer × 100 ,
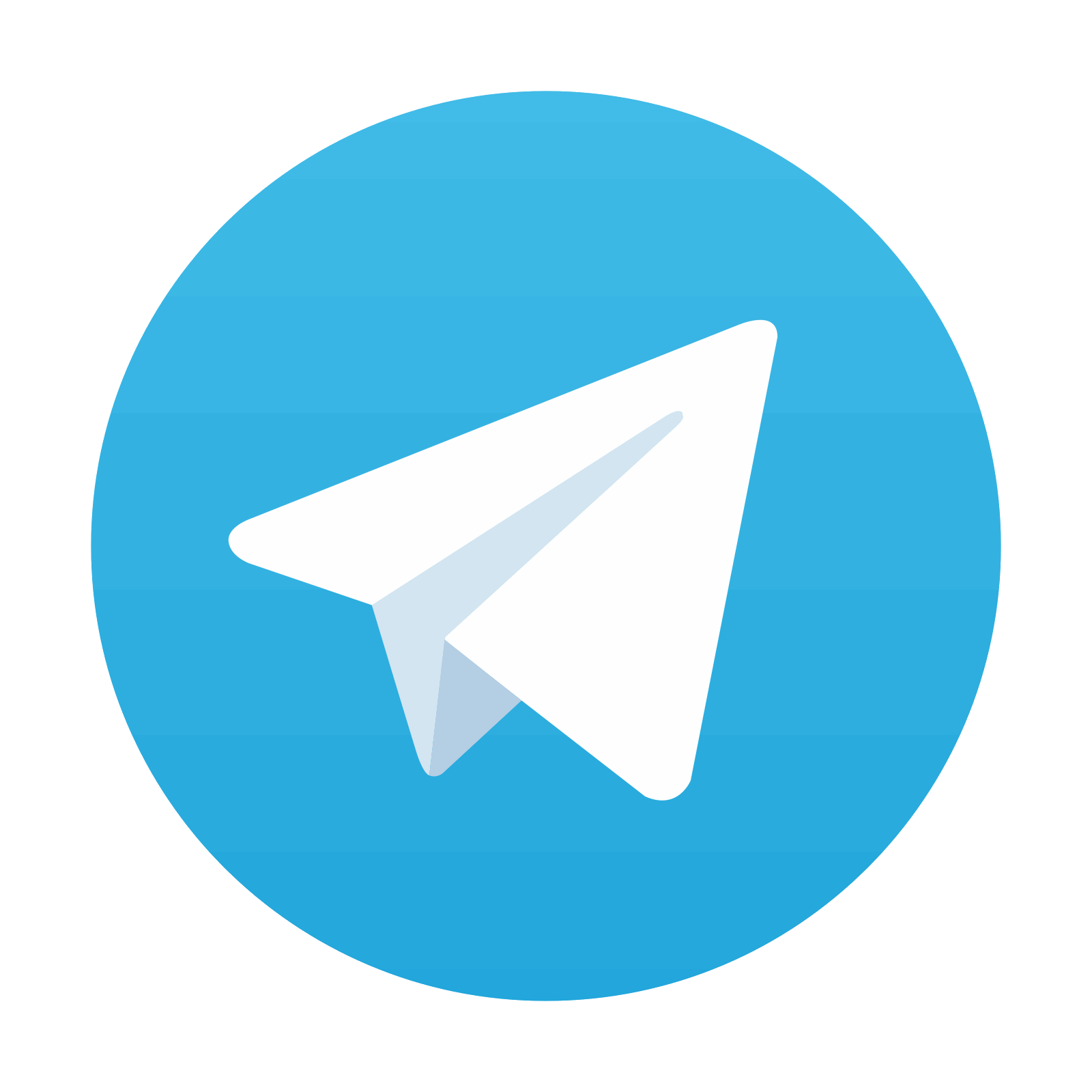
Stay updated, free dental videos. Join our Telegram channel

VIDEdental - Online dental courses
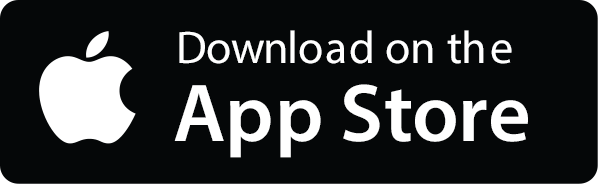
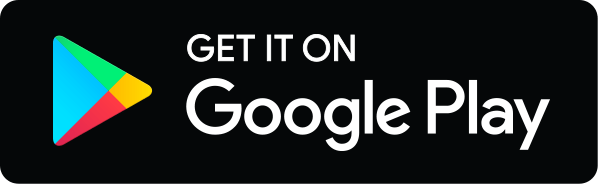