Abstract
Objectives
The purpose of this study was to compare the mechanical and rheological properties of resin-based pit and fissure sealants to flowable resin composites in order to define clinical indications based on these properties.
Methods
Eight flowable resin composites (Admira Flow, Filtek Supreme XT Flow, FlowLine, Grandio Flow, Point-4 Flowable, Premise Flowable, Revolution Formula 2, X-Flow) and four resin-based pit and fissure sealants (Clinpro, Delton FS + , Estiseal F, Guardian Seal) were used in this study. Their filler weight content was measured by thermogravimetric analysis. Mechanical properties were measured: dynamic and static moduli of elasticity, flexural strength and Vickers microhardness. Rheological measurements were performed using a dynamic oscillation rheometer.
Results
Flowable resin composites have by far better mechanical properties than pit and fissure sealants, except for Delton FS + . All the materials tested are non-Newtonian, shear thinning fluids. They all showed elasticity even at the lowest frequencies but elasticity differs pretty much from one material to another.
Significance
: Resin-based pit and fissure sealants seem appropriate for preventive pit and fissure sealing. For enlarged fissures, it can be assumed that flowable resin composites with low elasticity at low frequency are more appropriate.
1
Introduction
Although occlusal surfaces represent only 12.5% of the total surfaces of the permanent dentition, they account for almost 50% of the caries in school children . Tooth surfaces with pits and fissures are thus extremely vulnerable to the development of caries . Pit and fissure sealants were introduced in the 1960s as an attempt to prevent occlusal dental caries. From then, their effectiveness has been demonstrated several times .
Three main types of materials are available as pit and fissure sealants: glass ionomer cements, compomers and resins. Since the retention rate of glass ionomer cements, as well as resin modified glass ionomer cements, used as pit and fissure sealants is quite poor , the fact that they release active F − in the surrounding enamel is very important. Indeed, they exert a cariostatic effect even after they have disappeared macroscopically and this effect can reasonably be associated to increased levels of F − on the enamel surface as well as to remnants of the material in the depths of the fissures . For these reasons, glass ionomer cements should be considered more a F − vehicle than a traditional fissure sealant and despite their lower retention rate as compared with resin-based pit and fissure sealants, there is today no conclusive evidence that one material is superior to the other in preventing dental caries .
Compomers were developed to combine the advantages of both glass ionomer cements and resins. Only little scientific literature is available concerning the use of compomers as pit and fissure sealants. It is known that the incorporation of fluoride into resin and its release may compromise the integrity of the material and that the amount of F − released from compomers is considerably less than for glass ionomers . However, Puppin-Rontani et al. showed comparability with resin-based fissure sealants after a two year clinical study .
The caries preventive action of resin-based materials relies on the establishment of an effective mechanical obstacle to the leakage of nutrients to cariogenic microorganisms in the deeper parts of fissures . The major criterion of success of these materials is thus based on their retention rate unlike glass ionomer sealants. They may be either filled or unfilled, and chemically or light-cured. Many studies have demonstrated the effectiveness of chemically cured resin-based sealants in terms of retention rate . The performance levels of light-cured resin-based sealants are similar to those of chemically cured ones within an observation period of up to 5 years . However, for longer observation periods, chemically cured sealants seem to give better results even if for obvious practical reasons light-cured materials are by far more popular among practitioners nowadays than chemically cured ones. The addition of filler particles to resin-based pit and fissure sealants seem to have minor effect on clinical results and both filled and unfilled materials have similar retention rates but this is still discussed .
The use of flowable resin composites as pit and fissure sealants has also been suggested, as their formulations are quite close to those of traditional resin-based sealants and their physical and mechanical properties are supposed to be better. Their performances have been demonstrated to be comparable to those of resin-based sealants in both in vivo and in vitro studies where retention rate as well as microleakage and microtensile bond strength were evaluated.
The purpose of this study was to compare physical, mechanical and rheological properties of traditional resin-based pit and fissure sealants and flowable resin composites in order to determine their ability to resist to occlusal stresses and to efficiently seal dental pits and fissures. Their inorganic fraction was also characterized. Dynamic and static moduli of elasticity, flexural strength and Vickers microhardness were measured. The inorganic fraction was analyzed using scanning-electron microscopy (SEM) and thermogravimetric analysis (TGA). Rheological measurements were performed using a dynamic oscillatory rheometer. The tested null hypothesis was that both pit and fissure sealant and flowable resin composites are efficient in pit and fissure sealing considering their physical, mechanical and rheological properties.
2
Materials and methods
2.1
Materials tested
Eight commercially available flowable resin composites and four commercially available resin-based pit and fissure sealants were tested in this study. They are listed in Table 1 .
Material | Manufacturer | Shade | Batch |
---|---|---|---|
Flowable composites | |||
Admira Flow | Voco (Cuxhaven, Germany) | A2 | 500244 |
Filtek Supreme XT Flow | 3M-ESPE (St. Paul, MN, USA) | A3 | 680934 |
FlowLine | Heraeus-Kulzer (Weihrheim, Germany) | A2 | 10103 |
Grandio Flow | Voco (Cuxhaven, Germany) | A3 | 570666 |
Point-4 Flowable | Kerr (Orange, CA, USA) | A3 | 408419 |
Premise Flowable | Kerr (Orange, CA, USA) | A3 | 06-124102 |
Revolution Formula 2 | Kerr (Orange, CA, USA) | A3 | 4-1336 |
X-Flow | Dentsply-De Trey (Konstanz, Germany) | A3 | 502000636 |
Pit and fissure sealants | |||
Clinpro | 3M-ESPE (St. Paul, MN, USA) | N/A | 5FG |
Delton FS + | Dentsply-De Trey (Konstanz, Germany) | N/A | 40825 |
Estiseal F | Heraeus-Kulzer (Weihrheim, Germany) | N/A | 10027 |
Guardian Seal | Kerr (Orange, CA, USA) | N/A | 403412 |
2.2
Percentage and morphology of the inorganic fraction
2.2.1
Weight percentage of fillers
The weight percentage of fillers was determined using Thermogravimetric Analysis (TGA/SDTA861 e , Mettler-Toledo, Greinfensee, Switzerland). It evaluated weight changes as a function of time and temperature during a thermal program ranging from 30 °C to 900 °C at the rate of 10 °C/min in normal atmosphere followed by air cooling to room temperature. The calculated ratio between the final weight of the sample and its nominal weight is assigned to the inorganic fraction. Three samples of each material were analyzed.
2.2.2
Fillers morphology
The fillers morphology was determined using SEM. Unpolymerized monomers were removed by a washing technique: 0.5 g of each material was dissolved in 4 ml of acetone and centrifuged for 5 min at 700 × g . This process was repeated three times using acetone and three more with chloroform for a further washing and to ensure a complete elimination of the unpolymerized resin. The remaining fillers – including prepolymerized fillers – were suspended in 2.5 ml of absolute ethanol, smeared on a glass slide (7.5 cm × 2.5 cm) and dried at 37 °C during 6 h. After gold-coating, fillers were observed by SEM (Leica Stereoscan S-260, Cambridge, UK) at 5000 and 10,000 magnifications, operating at 20 kV under low-vaccuum.
2.3
Mechanical properties
Five specimens of each material were prepared in metallic molds of 25 mm × 2 mm × 2 mm as specified in ISO-4049 specification . After filling the mold to excess, their surface was covered by a mylar strip and a glass slide, then pressure was applied to extrude excess materials. Samples were light-cured using two conventional curing devices (XL 3000, 3M-ESPE, St Paul, MN, USA, 650 mw/cm 2 ) in order to enlarge the irradiated surface by four overlapping irradiations for a total of 60 s on both sides. The same specimen was used to measure the following properties: dynamic modulus, static modulus and flexural strength, Vickers microhardness. Specimens were stored for 7 days in water at 37 °C before measurement.
2.4
Dynamic modulus
The dynamic modulus of elasticity was measured non-destructively by the impulse excitation technique . Each sample was set in flexural vibration by a light mechanical impulse. The signal produced was captured via a microphone placed underneath the sample by a special signal analyzer, the Grindosonic (Lemmens Elektronics, Haasrode, Belgium). Modulus of elasticity (GPa) was calculated from the fundamental frequency, the mass, the width, the length and the thickness of the bar as follows:
E d = 0.9465 m f t 2 b L 3 t 3 T 1
where m is the mass of the bar, b the width of the bar, L the length of the bar, t the thickness of the bar, f t the fundamental frequency of the bar in flexion – which appears on the screen of the machine, and T 1 a correction factor for fundamental flexural mode to account for finite thickness of the bar, Poisson’s ratio and other constants. Poisson’s ratio is the ratio of transverse strain (contraction) to axial strain (elongation) in a uniaxial tensile test . When applied to dental resin composites, its value lies around 0.30 , that value has been taken as the mean value for all the materials tested in this study.
2.5
Static modulus and flexural strength
The static modulus was measured using a three-point bending setup according to the ISO-4049 specification . Samples were loaded in an Instron machine (Instron 5566, High Wycombe, UK) at a speed rate of 0.75 mm/min until fracture occurred. The load and the corresponding deflection were recorded and used to calculate the modulus (GPa) and the strength (MPa):
E s = F l 3 4 b h 3 d
where F is the load, l the distance between the supports, b the width of the bar, h the thickness of the bar and d the deflection corresponding to the load F .
The flexural strength was calculated as follows:
δ = 3 F l 2 b h 2
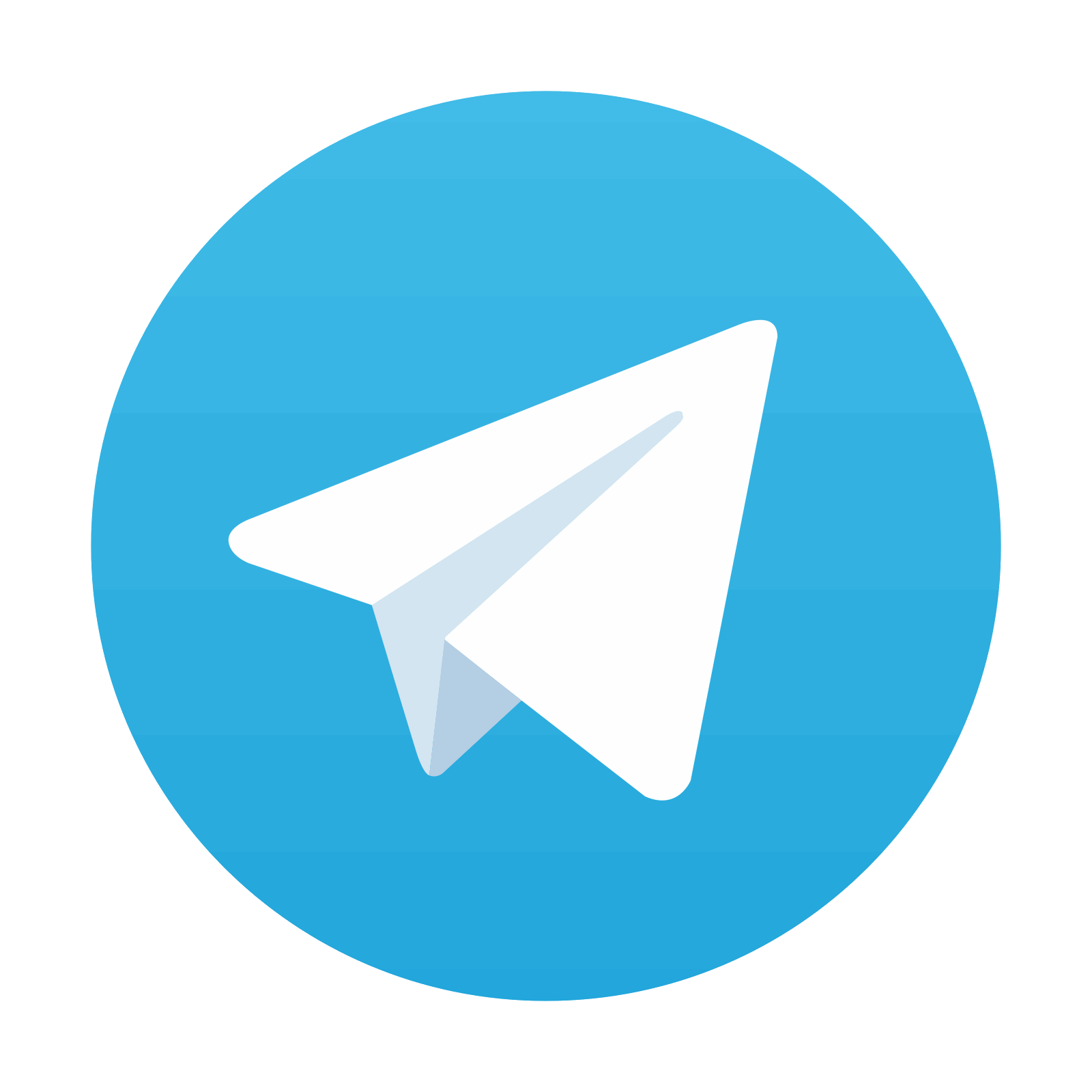
Stay updated, free dental videos. Join our Telegram channel

VIDEdental - Online dental courses
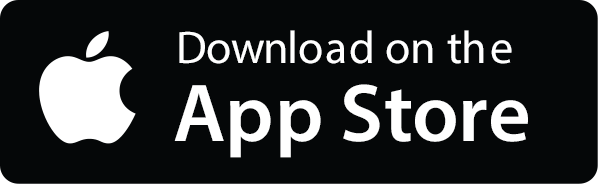
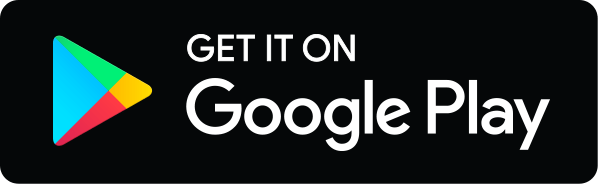