Abstract
Objective
Amorphous bioglass 45S5 has been used for many years as bone substitute material. Bioactive glasses are also suitable as coating materials for implants in order to improve the bone ongrowth behavior. We hypothesize that both the apatite formation on the surface and the chemical stability can be improved by crystallization of the bioglass.
Methods
Synthesized amorphous bioglass 45S5 specimens as well as samples which were crystallized at 1000 °C were stored in simulated body fluid for 1, 7, and 14 days. The respective apatite formation was gravimetrically determined and characterized by SEM and XRD analysis. Moreover, the degradation behavior was studied after storage in distilled water.
Results
The weight of the crystallized samples decreased 6.3% less than that of the amorphous samples. Calcium silica and calcium carbonate layers were found on amorphous bioglass after 7 and 14 days. However, apatite formation was observed only on the crystallized 45S5 samples after storage.
Significance
We conclude that the chemical resistance can be improved and, in parallel, a pronounced apatite formation on the surface of 45S5 can be obtained by controlled crystallization of the material for the particular test setup. Therefore, crystallized bioactive glasses should be considered to be promising coating material for dental implants.
1
Introduction
A bioactive behavior of the surface of an implant in direct bone contact can improve the bonding between the implant and the surrounding bone tissue. Bioglass 45S5, developed by Hench, exhibits a pronounced bioactivity . Amorphous bioactive glass is suitable especially to fill bone defects, but can also be used as coating material for implants in direct bone contact in order to enhance the osseointegration . The crystallized variant of bioglass 45S5, Na 2 Ca 2 Si 3 O 9 , reveals good osseointegration characteristics confirmed also in in vivo studies . In the contact of bioglass to bone, a bone-like apatite with good bone-bonding characteristics is formed on the surface of the coating. Kokubo and Takadama confirmed that such in vivo apatite formation can be simulated in vitro using so-called simulated body fluids (SBF) . The bioactivity of a material is thereby defined as the potential to form bone-like hydroxyapatite when transplanted into bone tissue. SBF is a non-cellular solution that imitates human blood plasma. Therefore, SBF consists of ions in the same concentration range as human blood, but does not contain proteins, hormones, glucose, and vitamins. It was initially developed by Kokubo to reproduce in vitro apatite formation on bioactive materials such as bioactive glasses and other biomaterials. Thus, an initial prediction can be made about the bioactivity and the corrosion behavior of a novel biomaterial . SBF solutions cause structural and chemical changes to the surface of the material. In principle, three processes occur in parallel: leaching, degradation, and precipitation . The leaching process is characterized by a release of alkaline metals and alkaline earth metals, specifically by exchange of the cations H + and H 3 O + . The ion exchange increases the pH-value at the interface up to 7.4 . The degradation of the material occurs in parallel with the breakdown of silica-oxygen bondings by hydroxy ions. This breaking down occurs locally. Subsequently, silicon in the form of silicic acid, Si(OH) 4 , is released into the solution. The degradation will decrease if the glass consists of more than 60 wt.% of SiO 2 due to the respective high level of oxygen bridges within the glass structure . The hydrated silicic acid (SiOH) formed at the surface is surrounded by at least one hydroxy group bound to a silicon atom (silanole) by polymerization of neighboring organic silicon compounds. Thereby a silicic acid gel layer forms. In parallel, calcium and phosphorus are released from the glass by precipitation and form a calcium phosphate-rich phase on the surface. This calcium phosphate phase in the gel layer is initially amorphous (a-CaP). Subsequently, the CaP phase crystallizes into a carbonated hydroxyapatite (HCA) structure by the carbonate anions from the solution . The mechanisms of in vitro nucleation and crystal growth of the HCA layer are comparable to the in vivo situation and are accelerated by hydrated silicic acid . A bonding to the tissue is only possible when biologically active HCA is formed. The rate of tissue bonding correlates with the amount of HCA that is formed . In the current study, the bioactivity and the degradation behavior of amorphous and crystallized 45S5 in SBF solution and in distilled water is investigated in order to reveal differences in the reaction behavior of the two material variants. The forming of hydroxyapatite on the surface of the materials and the characteristics of the layers were studied in detail for different storage periods. We hypothesize that apatite formation on the surface and chemical stability can be improved by the crystallization of the bioglass.
2
Materials and methods
A bioactive glass, type 45S5 with the chemical oxides SiO 2 , Na 2 O, CaO, and P 2 O 5 , was synthesized as described in the following glass-forming process. The chemical components SiO 2 (33.67 g), Na 2 CO 3 (31.34 g), CaCO 3 (26.38 g), and CaHPO 4 (8.61 g) were homogenized using a tumbling mixer. The homogeneous powder was melted in a platinum crucible at 1350 °C for 1.5 h. The melted glass was rapidly poured into water to cool it and avoid the crystallization process. The solidified glass was then homogenized again in a tumbling mixer. The homogenized glass powder was melted again using the same parameters as described above. The melt was cast into graphite molds that had been preheated to 350 °C and the glass bars were stored at 450 °C for 24 h to relax possible residual stresses. The glass bars were then cut into small bars (1.0 mm × 2.0 mm × 1.5 mm) using a diamond charged saw (Buehler, Düsseldorf, Germany). The samples were ground using SiC grinding paper. One batch of samples was treated in a ceramic furnace at 1000 °C for 1 h to crystallize them. The optimum crystallization temperature for the bioglass was determined using DSC analysis (DSC404, Netzsch, Selb, Germany). The samples were embedded in fired alumina powder during the thermal process to avoid deformations due to nonhomogeneous heat distribution. The chemical composition of the synthesized bioglass 45S5 was determined by X-ray fluorescence (XRF) analysis (PW2400, Pananalytical, Kassel, Germany). X-ray diffraction (XRD) measurements (PW 3710, Philips, Eindhoven, The Netherlands) were then performed on the amorphous and on the heat-treated samples to determine their crystallinity. Samples of both material variants were stored in SBF solution according to Kokubo and in distilled water for 1, 7, and 14 days, respectively, in order to study in vitro the apatite formation and the degradation behavior. The composition of the SBF solution is given in Table 1 . In contrast to Kokubo, the samples were stored in 1.5 l of the SBF solution (37 °C) for 1, 7, and 14 days, respectively, rinsed with distilled water, dried, and examined for crystal formation. In parallel, one batch of samples of both material variants was stored in distilled water to analyze their degradation behavior. The fluids were stirred during storage. The corrosion reactions of the samples in both SBF and distilled water were compared. Finally, gravimetrical examinations were performed to determine the loss of weight caused by corrosion reactions and degradation, on the one hand, and the increase of weight due to apatite formation, on the other hand. Scanning electron microscopy (SEM) analyses (FEI ESEM XL30 FEG, Philips, Eindhoven, The Netherlands) were performed to characterize morphological changes after corrosion, and formation and growth of apatite. The chemical composition was determined using energy dispersive X-ray spectroscopy (EDX). The crystalline phases formed were analyzed by XRD. Optical emission spectrometry (ICP-OES) technique (ICP-Spectrometer Plasma 2000, Perkin Elmer, Rodgau, Germany) was used for elemental analysis of the solutions.
Chemical component | Company | Amount for 1 l SBF |
---|---|---|
NaCl (sodium chloride) | VWR International, Leuven, Belgium | 7.996 g |
NaHCO 3 (potassium hydrogen carbonate) | Bernd Kraft, Duisburg | 0.350 g |
KCl (potassium chloride) | Merck, Darmstadt | 0.224 g |
K 2 HPO 4 ·3H 2 O | Bernd Kraft, Duisburg | 0.228 g |
MgCl 2 ·6H 2 O | Merck, Darmstadt | 0.305 g |
1 kmol/m 3 HCl (hydrogen chloride) | Merck, Darmstadt | 40.0 cm 3 |
CaCl 2 , (calcium chloride) | Merck, Darmstadt | 0.278 g |
Na 2 SO 4 (sodium sulfate) | Merck, Darmstadt | 0.071 g |
(HOCH 2 ) 3 CNH 2 | Merck, Darmstadt | 6.057 g |
1 kmol/m 3 HCl (hydrogen chloride) | Merck, Darmstadt | Amount determined by pH-value measurement |
2
Materials and methods
A bioactive glass, type 45S5 with the chemical oxides SiO 2 , Na 2 O, CaO, and P 2 O 5 , was synthesized as described in the following glass-forming process. The chemical components SiO 2 (33.67 g), Na 2 CO 3 (31.34 g), CaCO 3 (26.38 g), and CaHPO 4 (8.61 g) were homogenized using a tumbling mixer. The homogeneous powder was melted in a platinum crucible at 1350 °C for 1.5 h. The melted glass was rapidly poured into water to cool it and avoid the crystallization process. The solidified glass was then homogenized again in a tumbling mixer. The homogenized glass powder was melted again using the same parameters as described above. The melt was cast into graphite molds that had been preheated to 350 °C and the glass bars were stored at 450 °C for 24 h to relax possible residual stresses. The glass bars were then cut into small bars (1.0 mm × 2.0 mm × 1.5 mm) using a diamond charged saw (Buehler, Düsseldorf, Germany). The samples were ground using SiC grinding paper. One batch of samples was treated in a ceramic furnace at 1000 °C for 1 h to crystallize them. The optimum crystallization temperature for the bioglass was determined using DSC analysis (DSC404, Netzsch, Selb, Germany). The samples were embedded in fired alumina powder during the thermal process to avoid deformations due to nonhomogeneous heat distribution. The chemical composition of the synthesized bioglass 45S5 was determined by X-ray fluorescence (XRF) analysis (PW2400, Pananalytical, Kassel, Germany). X-ray diffraction (XRD) measurements (PW 3710, Philips, Eindhoven, The Netherlands) were then performed on the amorphous and on the heat-treated samples to determine their crystallinity. Samples of both material variants were stored in SBF solution according to Kokubo and in distilled water for 1, 7, and 14 days, respectively, in order to study in vitro the apatite formation and the degradation behavior. The composition of the SBF solution is given in Table 1 . In contrast to Kokubo, the samples were stored in 1.5 l of the SBF solution (37 °C) for 1, 7, and 14 days, respectively, rinsed with distilled water, dried, and examined for crystal formation. In parallel, one batch of samples of both material variants was stored in distilled water to analyze their degradation behavior. The fluids were stirred during storage. The corrosion reactions of the samples in both SBF and distilled water were compared. Finally, gravimetrical examinations were performed to determine the loss of weight caused by corrosion reactions and degradation, on the one hand, and the increase of weight due to apatite formation, on the other hand. Scanning electron microscopy (SEM) analyses (FEI ESEM XL30 FEG, Philips, Eindhoven, The Netherlands) were performed to characterize morphological changes after corrosion, and formation and growth of apatite. The chemical composition was determined using energy dispersive X-ray spectroscopy (EDX). The crystalline phases formed were analyzed by XRD. Optical emission spectrometry (ICP-OES) technique (ICP-Spectrometer Plasma 2000, Perkin Elmer, Rodgau, Germany) was used for elemental analysis of the solutions.
Chemical component | Company | Amount for 1 l SBF |
---|---|---|
NaCl (sodium chloride) | VWR International, Leuven, Belgium | 7.996 g |
NaHCO 3 (potassium hydrogen carbonate) | Bernd Kraft, Duisburg | 0.350 g |
KCl (potassium chloride) | Merck, Darmstadt | 0.224 g |
K 2 HPO 4 ·3H 2 O | Bernd Kraft, Duisburg | 0.228 g |
MgCl 2 ·6H 2 O | Merck, Darmstadt | 0.305 g |
1 kmol/m 3 HCl (hydrogen chloride) | Merck, Darmstadt | 40.0 cm 3 |
CaCl 2 , (calcium chloride) | Merck, Darmstadt | 0.278 g |
Na 2 SO 4 (sodium sulfate) | Merck, Darmstadt | 0.071 g |
(HOCH 2 ) 3 CNH 2 | Merck, Darmstadt | 6.057 g |
1 kmol/m 3 HCl (hydrogen chloride) | Merck, Darmstadt | Amount determined by pH-value measurement |
3
Results
3.1
Amorphous and crystallized bioglass 45S5
The chemical composition of the synthesized bioglass in comparison to the theoretical composition of 45S5 initially developed by Hench is given in Table 2 . DSC analysis revealed a glass transition temperature of 550 °C and a crystallization temperature of approx. 770 °C ( Fig. 1 ). The typical diffraction pattern of an amorphous material (black curve) and the pattern for the crystallized glass (gray curve) were observed by XRD ( Fig. 2 ). Na 2 Ca 2 Si 3 O 9 was determined as the main crystal phase.
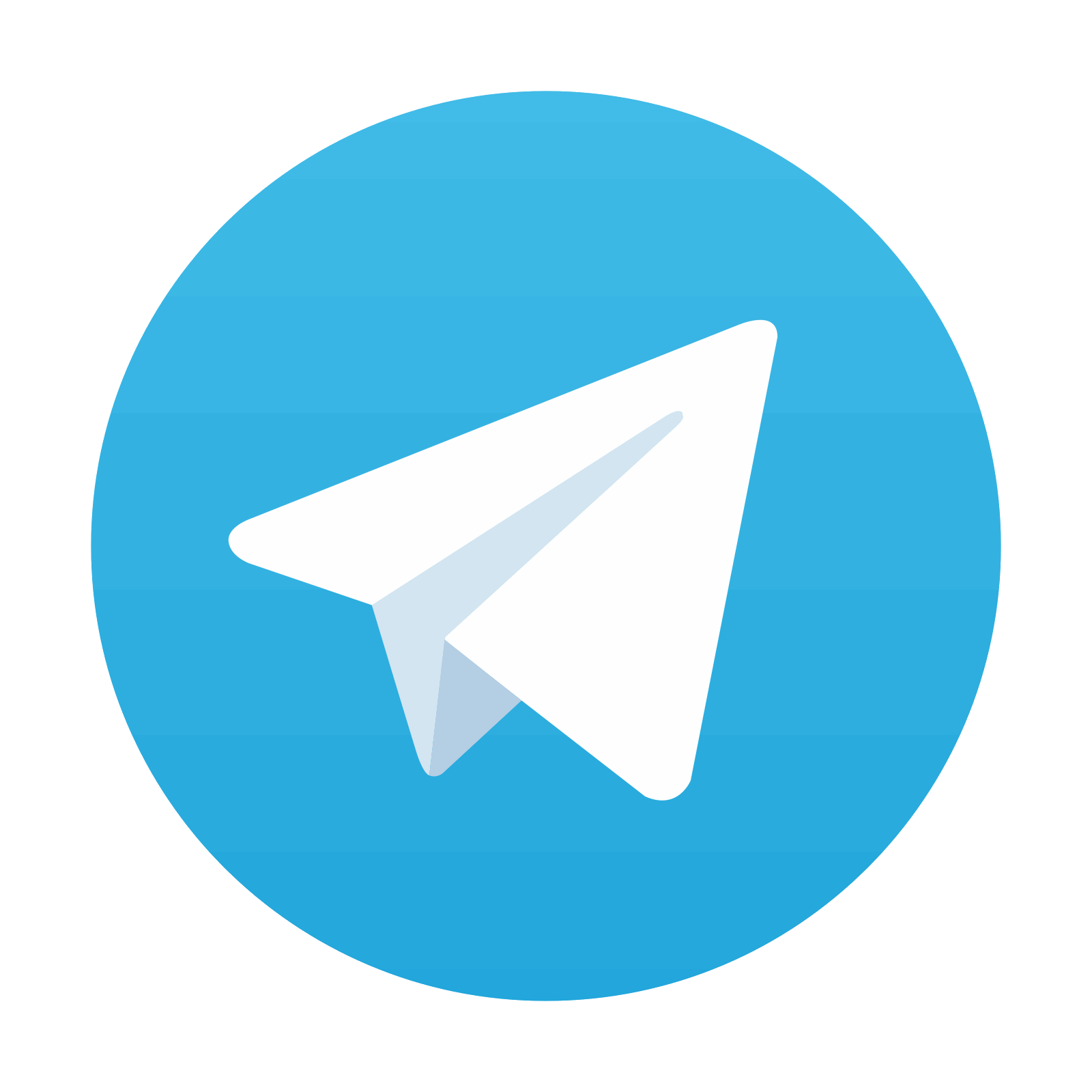
Stay updated, free dental videos. Join our Telegram channel

VIDEdental - Online dental courses
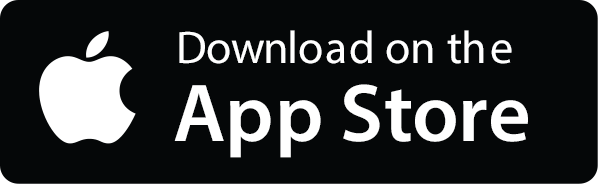
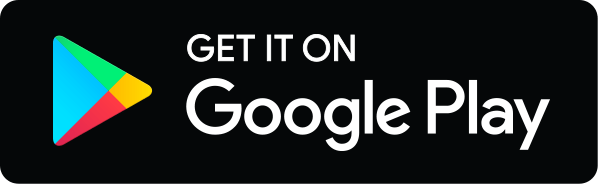
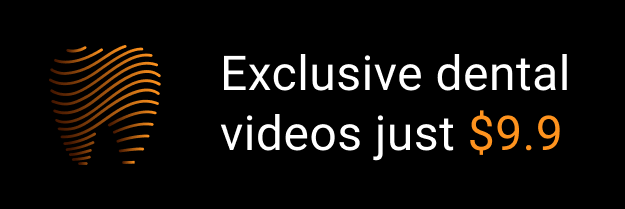