Abstract
Objective
This study evaluated the effect of adding diphenyliodonium hexafluorphosphate (DPI) as a third component of the free-radical photoinitiator system of model resin cements on their photopolymerization kinetics/stress and fundamental properties.
Methods
A model resin cement containing a 1:1 mass ratio of 2,2-bis[4-(2-hydroxy-3-methacryloxypropoxy)phenyl]propane and triethyleneglycol dimethacrylate was obtained. Camphorquinone (1 mol%) and 2-(dimethylamino)ethyl methacrylate (2 mol%) were added to monomer blend. Six mixtures were obtained by incorporation of 0 (control), 0.25, 0.5, 1, 2, or 4 mol% of DPI. The cements were loaded with a 60% mass fraction of silanated glass fillers. Polymerization kinetics (using Fourier-transform near-infrared spectroscopy), flexural strength and modulus, water uptake/solubility, and polymerization stress were assessed. Data were statistically analyzed using one-way ANOVA and Student–Newman–Keuls’ test ( P ≤ 0.05).
Results
In the DPI-modified materials, the onset of autodeceleration occurred earlier and at higher conversion compared with the control cement. The addition of DPI also led to a more active early-stage polymerization. The flexural modulus was generally higher for DPI-containing materials. Water uptake and solubility were generally lower for materials with DPI fractions ≥0.5%. Significant increase of polymerization stress was observed only in the group with 1 mol% of DPI compared with the control cement.
Significance
The effect of DPI on the photopolymerization reactivity of the cement is concentration-dependent; the use of DPI could result in better polymerization efficiency of resin-based cements.
1
Introduction
Resin cements are used in several clinical procedures including cementation of ceramic restorations and luting of glass fiber posts into root canals. Although self- and dual-cure materials are available, photoactivated cements have the advantages of longer working time and increased color stability . In order to obtain high bond strength after cementation, adequate light energy reaching the cement layer is necessary . It has been demonstrated that situations with attenuated curing light may impair the polymerization mechanism . It has been also shown that the negative effect of the low irradiance may be overcome by increasing the light exposure time .
Another possibility to improve the polymerization potential of resin cements is to increase the reactivity of the photoactivated material by using more effective photoinitiator systems. Previous investigations reported on the use of onium salts to enhance the curing kinetics of adhesive systems . Diaryliodonium salts, with a complex metal halide as a weakly nucleophilic counterion, are efficient photoinitiators for cationic polymerization . The low bond energy of the C I bond allows, under light exposure, allows decomposition of the excited iodonium into an aryliodo radical-cation, a reactive aryl radical and an anion . Although these photoinitiators are active when irradiated in a light range below 300 nm, it has been shown that the combined use of an onium salt and camphorquinone may promote decomposition of the salt, enhancing the free-radical polymerization of (di)methacrylates .
The use of onium salts in three-component initiating systems for dental resin cements could potentially render the material more prone to cure in situations with attenuated curing light, such as beneath ceramic restorations or within the confines of a root canal. However, it has been shown that the rate of polymerization has a direct impact on the stress state during polymerization , thus the polymerization stress (PS) can also be increased. The purpose of this study was to investigate the effect of adding an onium salt on the photopolymerization kinetics, PS and fundamental properties of a model dental resin cement. The hypothesis tested was that the addition of the onium salt would enhance the polymerization without being detrimental to the other material characteristics.
2
Materials and methods
2.1
Formulation of the model resin cements
A model dimethacrylate comonomer blend based on a 1:1 mass ratio of 2,2-bis[4-(2-hydroxy-3-methacryloxypropoxy)phenyl]propane and triethyleneglycol dimethacrylate (TEGDMA) (Esstech Inc., Essington, PA, USA) was loaded with a 1 mol% of camphorquinone (Esstech) and 2 mol% of 2-(dimethylamino)ethyl methacrylate (Sigma–Aldrich, St. Louis, MO, USA). From the model blend six mixtures were obtained by incorporation of 0 (control), 0.25, 0.5, 1, 2 or 4 mol% of diphenyliodonium hexafluorphosphate (DPI, Sigma–Aldrich). The mixtures were loaded with a 60% mass fraction of silanated barium borosilicate glass fillers (Esstech, 0.7 μm average size). All chemicals were used as received.
2.2
Photopolymerization kinetics
Real-time monitoring of the polymerization kinetics was carried out using Fourier-transform near-infrared spectroscopy (Nexus 670; Nicolet Instrument, Madison, WI, USA). The area of the methacrylate CH 2 peak (6163 cm −1 ) was recorded with 4 scans per spectrum and 4-cm −1 resolution. To monitor the change of the carbon–carbon double bond concentration during polymerization, a series run collected the predefined peak area as a function of time. The detailed experimental procedure has been described previously . Samples were irradiated for 40 s with a light-emitting diode (LED) curing unit (Radii-Cal; SDI, Bayswater, Victoria, Australia) at an irradiance of 645 mW/cm 2 . Three specimens were tested for each material. Degree of C C conversion (DC) vs. time data were plotted and sigmoidal non-linear regressions were used for curve fitting. As the coefficient of determination was greater than 0.99 for all curves, the rate of polymerization ( R p ) was calculated using these data-fitted plots. Based on the kinetics results, the material with 4% DPI was not further tested, as no significant improvement in DC was observed.
2.3
Flexural strength and modulus
Flexural strength tests for all materials were performed using bar specimens with dimensions of 7 mm in length, 2 mm in width, and 1 mm in height ( n = 10). The reduced bar dimensions were necessary to match the length of the bar with the diameter of the light guide tip of the curing unit; also, the reduced dimension allowed polymerization of the specimens using a single photoactivation exposure. A 3-point bending test was carried out 24 h after irradiation on a mechanical testing machine (model 4411; Instron, Canton, MA, USA) using a span width of 5 mm and a crosshead speed of 0.5 mm/min. The flexural strength ( σ ) and flexural modulus ( E f ) were calculated from the load–displacement trace.
2.4
Water uptake and solubility
Five cylindrical specimens (6 mm in diameter × 1 mm thick) photopolymerized for 40 s on each side were obtained for each material. The specimens were dry-stored at 37 °C and repeatedly weighed after 24-h intervals using an analytical balance (Discovery DV215CD; Ohaus Corporation, Pine Brook, NJ, USA) accurate to 0.01 mg until a constant mass was obtained. The samples were then individually placed in sealed vials, immersed in distilled water, and stored at 37 °C. After 21 days, the surface water of the specimens was removed by blotting with absorbent paper and waving the specimen in the air for 10 s, and the weighing procedures were repeated. The specimens were then dry-stored again at 37 °C and reweighed after 24-h intervals until a constant final dry mass was obtained. Water uptake ( W up ) and solubility ( W sl ) were calculated from the percentage differences in mass gain or loss during the sorption and desorption cycles.
2.5
Polymerization stress
The detailed experimental setup has been previously described . Briefly, two poly(methyl methacrylate) rods (28 or 13 mm in length × 6 mm in diameter) had one of their flat surfaces sandblasted with 250-μm alumina particles. On the shorter rod, in order to allow the highest possible light transmission, the opposite surface was polished with SiC paper and alumina paste. The sandblasted surfaces were treated with methyl methacrylate (JET; Clássico, São Paulo, SP, Brazil) and coated with a layer of unfilled resin (Scotchbond; 3M ESPE), which was photoactivated for 30 s. The rods were attached to a universal testing machine (model 5565; Instron) having a 1-mm distance between the surfaces. This distance was kept constant by attaching an extensometer to both rods (model 2630-101; Instron). The LED light guide tip was placed in contact with the polished surface of the shorter rod. The resin cements were placed between the rods and formed into a cylinder. Photoactivation was carried out for 40 s through the rod and the stress monitored for 10 min. Polymerization stress (PS) values were calculated in MPa. Five specimens were tested for each material.
2.6
Statistical analysis
Data for DC, maximum rate of polymerization ( Rmaxp
R p max
), σ , E f , W sp , W sl and PS were separately submitted to one-way analysis of variance and the Student–Newman–Keuls’ post hoc test ( P < 0.05).
2
Materials and methods
2.1
Formulation of the model resin cements
A model dimethacrylate comonomer blend based on a 1:1 mass ratio of 2,2-bis[4-(2-hydroxy-3-methacryloxypropoxy)phenyl]propane and triethyleneglycol dimethacrylate (TEGDMA) (Esstech Inc., Essington, PA, USA) was loaded with a 1 mol% of camphorquinone (Esstech) and 2 mol% of 2-(dimethylamino)ethyl methacrylate (Sigma–Aldrich, St. Louis, MO, USA). From the model blend six mixtures were obtained by incorporation of 0 (control), 0.25, 0.5, 1, 2 or 4 mol% of diphenyliodonium hexafluorphosphate (DPI, Sigma–Aldrich). The mixtures were loaded with a 60% mass fraction of silanated barium borosilicate glass fillers (Esstech, 0.7 μm average size). All chemicals were used as received.
2.2
Photopolymerization kinetics
Real-time monitoring of the polymerization kinetics was carried out using Fourier-transform near-infrared spectroscopy (Nexus 670; Nicolet Instrument, Madison, WI, USA). The area of the methacrylate CH 2 peak (6163 cm −1 ) was recorded with 4 scans per spectrum and 4-cm −1 resolution. To monitor the change of the carbon–carbon double bond concentration during polymerization, a series run collected the predefined peak area as a function of time. The detailed experimental procedure has been described previously . Samples were irradiated for 40 s with a light-emitting diode (LED) curing unit (Radii-Cal; SDI, Bayswater, Victoria, Australia) at an irradiance of 645 mW/cm 2 . Three specimens were tested for each material. Degree of C C conversion (DC) vs. time data were plotted and sigmoidal non-linear regressions were used for curve fitting. As the coefficient of determination was greater than 0.99 for all curves, the rate of polymerization ( R p ) was calculated using these data-fitted plots. Based on the kinetics results, the material with 4% DPI was not further tested, as no significant improvement in DC was observed.
2.3
Flexural strength and modulus
Flexural strength tests for all materials were performed using bar specimens with dimensions of 7 mm in length, 2 mm in width, and 1 mm in height ( n = 10). The reduced bar dimensions were necessary to match the length of the bar with the diameter of the light guide tip of the curing unit; also, the reduced dimension allowed polymerization of the specimens using a single photoactivation exposure. A 3-point bending test was carried out 24 h after irradiation on a mechanical testing machine (model 4411; Instron, Canton, MA, USA) using a span width of 5 mm and a crosshead speed of 0.5 mm/min. The flexural strength ( σ ) and flexural modulus ( E f ) were calculated from the load–displacement trace.
2.4
Water uptake and solubility
Five cylindrical specimens (6 mm in diameter × 1 mm thick) photopolymerized for 40 s on each side were obtained for each material. The specimens were dry-stored at 37 °C and repeatedly weighed after 24-h intervals using an analytical balance (Discovery DV215CD; Ohaus Corporation, Pine Brook, NJ, USA) accurate to 0.01 mg until a constant mass was obtained. The samples were then individually placed in sealed vials, immersed in distilled water, and stored at 37 °C. After 21 days, the surface water of the specimens was removed by blotting with absorbent paper and waving the specimen in the air for 10 s, and the weighing procedures were repeated. The specimens were then dry-stored again at 37 °C and reweighed after 24-h intervals until a constant final dry mass was obtained. Water uptake ( W up ) and solubility ( W sl ) were calculated from the percentage differences in mass gain or loss during the sorption and desorption cycles.
2.5
Polymerization stress
The detailed experimental setup has been previously described . Briefly, two poly(methyl methacrylate) rods (28 or 13 mm in length × 6 mm in diameter) had one of their flat surfaces sandblasted with 250-μm alumina particles. On the shorter rod, in order to allow the highest possible light transmission, the opposite surface was polished with SiC paper and alumina paste. The sandblasted surfaces were treated with methyl methacrylate (JET; Clássico, São Paulo, SP, Brazil) and coated with a layer of unfilled resin (Scotchbond; 3M ESPE), which was photoactivated for 30 s. The rods were attached to a universal testing machine (model 5565; Instron) having a 1-mm distance between the surfaces. This distance was kept constant by attaching an extensometer to both rods (model 2630-101; Instron). The LED light guide tip was placed in contact with the polished surface of the shorter rod. The resin cements were placed between the rods and formed into a cylinder. Photoactivation was carried out for 40 s through the rod and the stress monitored for 10 min. Polymerization stress (PS) values were calculated in MPa. Five specimens were tested for each material.
2.6
Statistical analysis
Data for DC, maximum rate of polymerization ( Rmaxp
R p max
), σ , E f , W sp , W sl and PS were separately submitted to one-way analysis of variance and the Student–Newman–Keuls’ post hoc test ( P < 0.05).
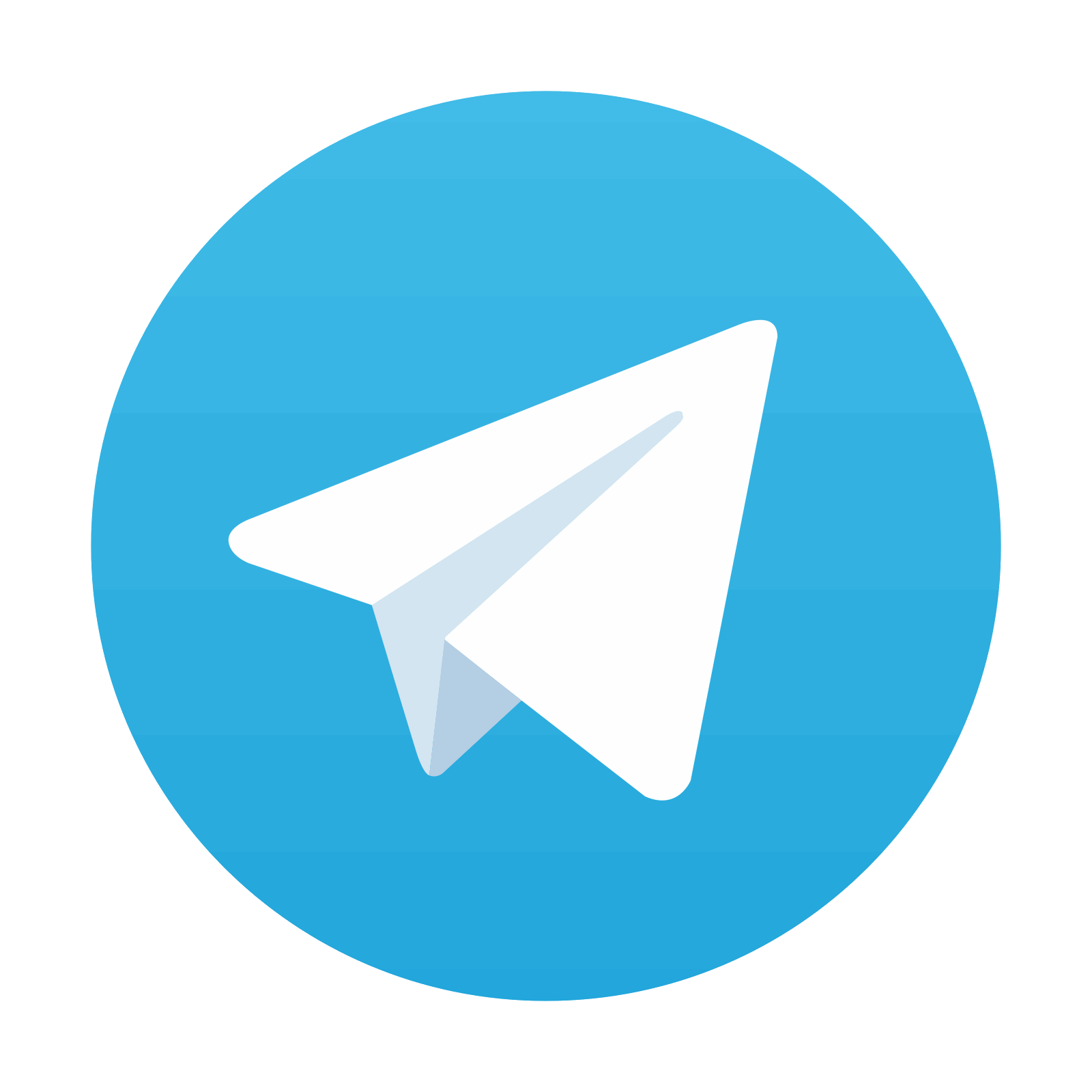
Stay updated, free dental videos. Join our Telegram channel

VIDEdental - Online dental courses
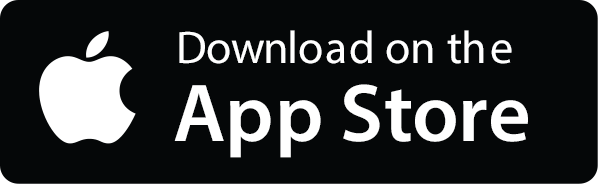
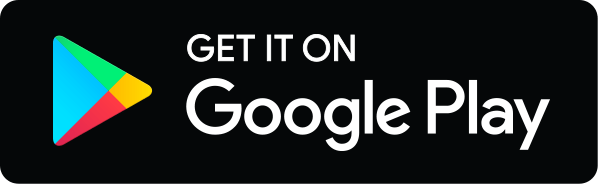