Abstract
Objectives
The objectives of this study were to synthesize two new siloxane-methacrylate (SM) monomers for application in dentin adhesives and to investigate the influence of different functionality of the siloxane-containing monomers on the adhesive photopolymerization, water sorption, and mechanical properties.
Methods
Two siloxane-methacrylate monomers (SM1 and SM2) with four and eight methacrylate groups were synthesized. Dentin adhesives containing BisGMA, HEMA and the siloxane-methacrylate monomers were photo-polymerized. The experimental adhesives were compared with the control adhesive (HEMA/BisGMA, 45/55, w/w) and characterized with regard to degree of conversion (DC), water miscibility of the liquid resin, water sorption and dynamic mechanical analysis (DMA).
Results
The experimental adhesives exhibited improved water miscibility as compared to the control. When cured in the presence of 12 wt% water to simulate the wet environment of the mouth, the SM-containing adhesives showed DC comparable to the control. The experimental adhesives showed higher rubbery modulus than the control under dry conditions. Under wet conditions, the mechanical properties of the formulations containing SM monomer with increased functionality were comparable with the control, even with more water sorption.
Significance
The concentration and functionality of the newly synthesized siloxane-methacrylate monomers affected the water miscibility, water sorption and mechanical properties of the adhesives. The experimental adhesives show improved water compatibility compared with the control. The mechanical properties were enhanced with an increase of the functionality of the siloxane-containing monomers. The results provide critical structure/property relationships and important information for future development of durable, versatile siloxane-containing dentin adhesives.
1
Introduction
During the last 20 years, the primary motivation for changing the resin chemistry of restorative composites was to reduce the polymerization shrinkage . The polymerization shrinkage occurs during the curing of the composite in the tooth cavity and may lead to the formation of marginal gaps. The silorane-based composite, introduced by 3 M in 2007, exhibits the lowest polymerization shrinkage (1.4 vol%) of the current composite restorative materials .
The hybrid monomer systems introduced by 3 M contain both siloxane and oxirane structural units. The main benefits of these resins are the very low polymerization shrinkage, the good biocompatibility, the very low water solubility of the monomers, and low water sorption of the formed polymer networks . This siloxane-based composite does not, however, exhibit the lowest polymerization stress . Other limitations, including increased exothermic effect (twice as high as dimethacrylate-based composites with similar filler load), the delay in establishing mechanical properties comparable to dimethacrylates, and the lower refractive index, have inhibited broad market acceptance of the silorane-based composite . The lower bond strength of the silorane composite with commercial dentin adhesives has raised concerns about the compatibility of these materials.
Siloxane-containing materials have been widely used as biomaterials in medical and pharmaceutical applications, due to their biocompatibility and low toxicity . For example, polysiloxane has a high refractive index and can be used as the accommodating intraocular lens by the polymerization of siloxane-methacrylate monomers . Siloxane-containing cross-linking agents are often used in drug delivery systems . Moreover, in the presence of siloxane groups, the stability of restorative materials can be improved . In this context, if siloxane groups could be incorporated into the dentin adhesive, it is expected that the durability of the dentin adhesives and compatibility with the silorane composite would be improved. However, polymers with siloxane groups are usually soft, and their mechanical strength may be low because of the flexibility of the siloxane groups . Therefore, modification of siloxane with methacrylate to take advantage of both the stability of siloxane groups and good mechanical properties of methacrylates could be a solution.
The twofold objectives of this work were: (1) to synthesize and characterize two new siloxane-methacrylate monomers with four and eight methacrylate groups and (2) to investigate the influence of chemical structure of siloxane-containing monomers used in dentin adhesives on the following properties: degree of conversion, water miscibility, water sorption and dynamic mechanical properties. To our knowledge, this investigation marks the first study of siloxane-methacrylate monomers used in dentin adhesives. The results provide critical structure/property relationships for siloxane-methacrylate monomers and important information for future development of durable, versatile siloxane-containing dentin adhesives.
2
Materials and methods
2.1
Materials
2,2-Bis[4-(2-hydroxy-3-methacryloxypropoxy)phenyl]-propane (BisGMA, Polysciences, Warrington, PA) and 2-hydroxyethylmethacrylate (HEMA, Acros Organics, NJ) were used as received without further purification, as monomers in dentin adhesives. Two siloxane methacrylate monomers (SM1 and SM2) were synthesized in our lab. The control adhesive resin consisted of HEMA and BisGMA with a mass ratio of 45/55. This control was used to compare with the experimental adhesive resins with HEMA/BisGMA/SM = 45/55 − x / x (w/w) ratio. The control and experimental adhesives were also formulated with 12 wt% water to simulate bonding in the mouth. The concentration of water was based on the total final weight of the adhesive resin. Camphorquinone (CQ, 0.5 wt%), ethyl-4-(dimethylamino)benzoate (EDMAB, 0.5 wt%) and diphenyliodonium hexafluorophosphate (DPIHP,1.0 wt%) were obtained from Aldrich (Milwaukee, WI, USA) and used as a three-component-photoinitiator system without further purification. 2,4,6,8-Tetramethyl-2,4,6,8-tetrakis(propyl glycidyl ether)cyclotetrasiloxane (TPGTS), glycerol dimethacrylate (GDMA, assay 85%, mixture of isomers), ethyl acetate, boron trifluoride diethyl etherate (BF 3 O(C 2 H 5 ) 2 ), anhydrous magnesium sulfate (MgSO 4 ), and all other chemicals were purchased from Sigma–Aldrich at reagent grade and used without further purification.
2.2
Synthesis of siloxane-methacrylate (SM) monomers
2,4,6,8-Tetramethyl-2,4,6,8-tetrakis(propyl glycidyl ether)cyclotetrasiloxane (TPGTS, 10 g, 0.0143 mol), 2-hydroxyethylmethacrylate (HEMA, 10 g, 0.076 mol) or glycerol dimethacrylate (GDMA, mixture of isomers, 13.75 g, 0.06 mol) and ethyl acetate (EA, 100 mL) were mixed together. Boron trifluoride diethyl etherate (0.2 mL) was added into this solution. The reaction was allowed to continue at room temperature for about 12 h, and it was monitored by thin layer chromatography (mobile phase: dichloromethane/hexane, 50/50, wt%). The product-containing solution was washed with distilled water and dried over anhydrous MgSO 4 . The solvent was removed with a rotary evaporator at 35–40 °C. The process for the synthesis of the two siloxane methacrylate monomers (SM1 and SM2) is shown in Scheme 1 . The yields of SM1 and SM2, which were colorless and viscous, were 92.4% and 95.0%, respectively. The structure of the synthesized compounds (SM1, SM2) was confirmed using FTIR (Spectrum 400, Perkin-Elmer, Waltham, MA), 1 H NMR and 13 C NMR (FT-400 MHz Bruker Spectrometer, DMSO as solvent) spectroscopies.
2.3
Preparation of adhesive formulations
The preparation of the control adhesive formulations has been reported . As shown in Table 1 , the control adhesive formulation (C0) consisted of HEMA and BisGMA with a mass ratio of 45/55 which is similar to widely used commercial dentin adhesives. In the experimental adhesive formulations (E-SM), siloxane-methacrylates were used as co-monomers to partially replace the BisGMA ( Table 1 ). The siloxane-methacrylates were included at 5, 15, and 25 wt%. The abbreviations E1, E2, and E3 represent 5, 15, and 25 wt% of either SM1 or SM2. Water at 12 wt% was added into C0, E3-SM1 and E3-SM2 to simulate the moist environment of the mouth. The composition of the three component initiator system was CQ (0.5 wt%), EDMAB (0.5 wt%) and DPIHP (1.0 wt%) with respect to the total amount of monomer . The resin mixtures were prepared in a brown glass vial. The solutions containing the monomers/photoinitiators were mixed for 48 h at room temperature to promote complete dissolution and formation of a homogeneous solution.
2.4
Real-time conversion and maximal polymerization rate
Real-time in situ monitoring of the photopolymerization of the adhesive formulations was performed using an infrared spectrometer (Spectrum 400 Fourier transform infrared spectrophotometer, Perkin-Elmer, Waltham, MA) at a resolution of 4 cm −1 . One drop of adhesive solution was placed on the diamond crystal top-plate of an attenuated total reflectance (ATR) accessory (Pike, GladiATR, Pike Technology, Madison, WI) and covered with a mylar film. A 40-s-exposure to the commercial visible-light-polymerization unit (Spectrum ® , Dentsply, Milford, DE), at an intensity of 550 mW cm −2 , was initiated after 50 spectra had been recorded. Real-time IR spectra were recorded continuously for 600 s after light curing began. A time-based spectrum collector (Spectrum TimeBase, Perkin-Elmer) was used for continuous and automatic collection of spectra during polymerization. Three replicates were obtained for each adhesive formulation. To determine degree of conversion, heavy water (deuterium oxide, 99.9%, D 2 O) (Cambridge Isotope Laboratories, Inc., Andover, MA, USA) was used in this study to reduce interference from the overlapping water peak at 1640 cm −1 . The change of the band ratio profile (1637 cm −1 (C C)/1608 cm −1 (phenyl)) was monitored. DC was calculated using the following equation, which is based on the decrease in the absorption intensity band ratios before and after light curing. The average of the last 50 values of time-based data points is reported as the DC value at 10 min.
DC ( % ) = 1 − absorbanc e 1637 cm − 1 sample / absorbanc e 1608 cm − 1 sample absorbanc e 1637 cm − 1 monomer / absorbanc e 1608 cm − 1 monomer × 100
The maximal polymerization rate was determined using the maximum slope of the linear region of the DC–time plots .
2.5
Viscosity measurement
Brookfield DV-II + Pro viscometer with a cone/plate set up was used to measure the viscosity of the formulations at varying shear rate 45–75 s −1 . For each formulation viscosity of three samples was measured at 25.0 ± 0.2 °C. The sample volume was kept constant to 0.5 mL.
2.6
Preparation of adhesive polymer specimens for property analyses
The preparation of polymer specimens for property analyses has been reported . In brief, round beams with a diameter of 1 mm and a length of at least 15 mm were prepared by injecting the adhesive formulations into glass molds (Fiber Optic Center, Inc., part no.: ST8100, New Bedford, MA). Fifteen specimens were prepared for each formulation. The samples were light polymerized with an LED light curing unit for 40 s (LED Curebox, 200 mW/cm 2 irradiance, Prototech, and Portland, OR). The polymerized samples were stored in the dark at room temperature for two days to provide adequate time for post-cure polymerization. The samples were subsequently extracted from the glass molds. The resultant beam specimens were used for water sorption, dynamic mechanical analysis and microscale morphologic characterization.
2.7
Water miscibility
Water miscibility is the property of the liquid monomer resin to mix with water, forming a homogeneous solution. In principle, the main focus is usually on the solubility of water in different formulations of the monomer resin. About 0.5 g of each neat resin was weighed into a brown vial, and water was added in increments of approximately 0.005 g until the mixture was visually observed to be turbid. The percentage of water in the mixture was noted ( w 1 ). The mixture was then back-titrated using the neat resin until the turbidity disappeared, and the percentage of water in the mixture was again noted ( w 2 ). Three samples were tested for each formulation and water miscibility of the liquid formulation was calculated as the average of w 1 and w 2 .
2.8
Water sorption
The water sorption protocol has been reported . In brief, water sorption was measured using cylindrical beam specimens (1 mm × 15 mm). Five specimens were prepared for each adhesive formulation.
Prewash . Each beam specimen was weighed in air ( m 1dry ) using an analytical balance with a resolution of 0.01 mg (Mettler Toledo, XS205 dualRange, Greifensee, Switzerland). The specimens were immersed in deionized water and stored at room temperature. The water was changed daily. After five days of prewash, the polymer specimens were allowed to dry in the vacuum chamber at 37 °C until a constant weight ( m 3dry ) was obtained. The solubility during the prewash experiment was calculated as:
Solubility 1 ( % ) = 100 m 1 dry − m 3 dry m 1 dry
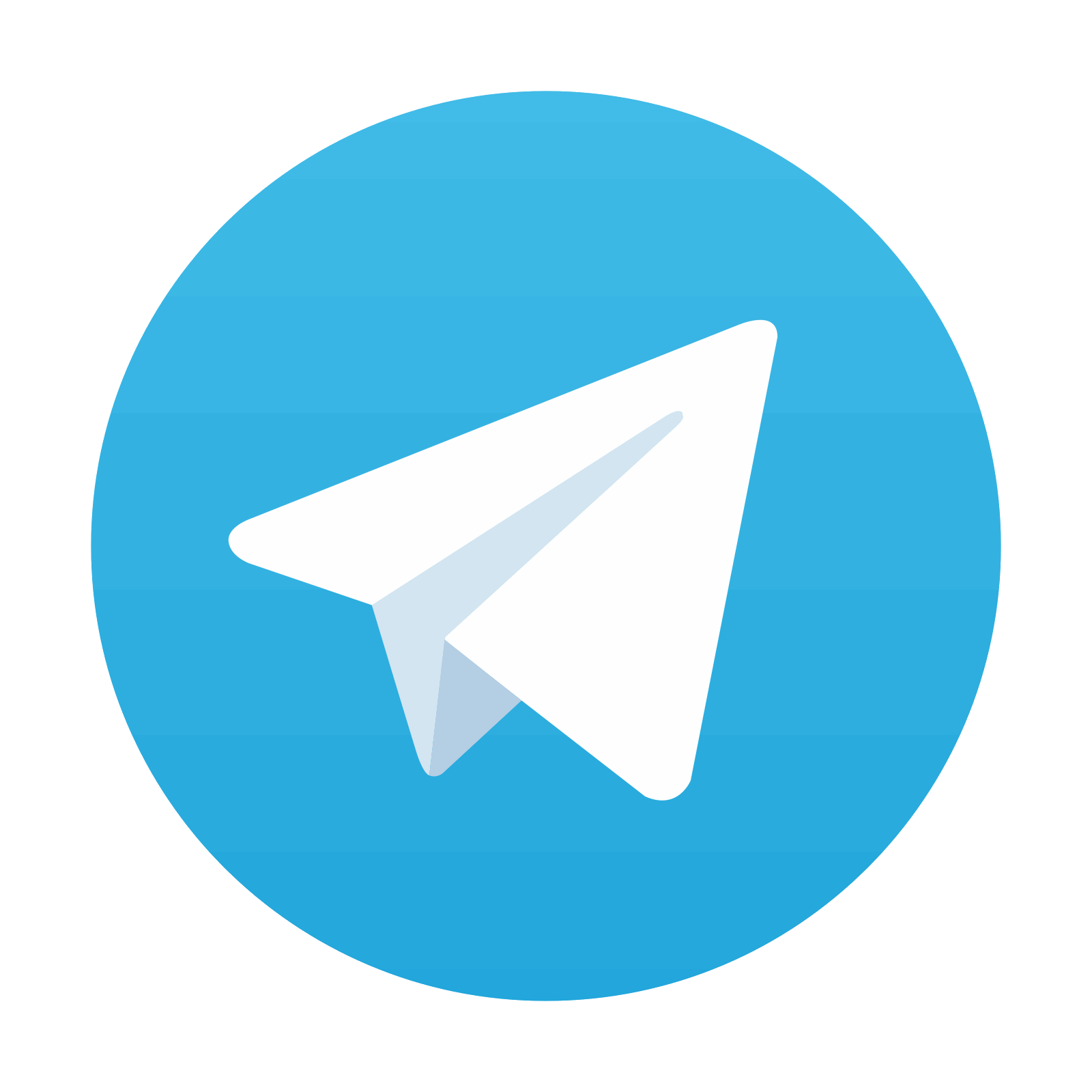
Stay updated, free dental videos. Join our Telegram channel

VIDEdental - Online dental courses
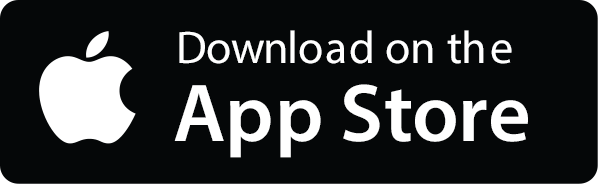
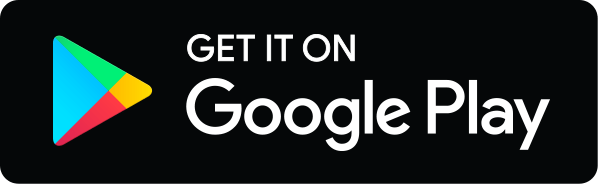