Abstract
Objectives
Self-adhesive resin cements have been recently introduced; however, there is little data available on their long-term performance. In this in vitro study, swept-source optical coherence tomography (OCT) at 1310 nm center wavelength was used for monitoring adaptation of indirect resin restorations after thermal cycling.
Methods
Resin inlays were luted to class-I cavities of extracted human teeth using three resin cements; Clearfil SA Luting (SA; Kuraray), Bistite II DC or Multibond II (Tokuyama Dental). Each cement was applied with or without pre-coating of dentin by a self-etch adhesive (Clearfil SE Bond) and a low-viscosity microfilled resin. OCT imaging was performed after 24 h, after 2000 and after 10,000 thermocycles ( n = 5). Selected samples were sectioned for interfacial observation by confocal laser scanning microscope (CLSM). Floor adaptation (percentage) was analyzed by software on 20 B-scans throughout each specimen, and subjected to statistical analysis by three-way ANOVA test at a significance level of 0.05.
Results
Resin cement type, resin coating and thermal aging all significantly affected adaptation ( p < 0.05). Initially, SA showed the highest adaptation; however, thermal aging significantly affected its sealing. The best results for all the cements were consistently achieved when the resin coating technique was applied where no deterioration of interfacial integrity was observed in the coated groups. CLSM closely confirmed OCT findings in all groups.
Significance
OCT could be used for monitoring of composite inlays with several interfacial resin layers. The application of a direct bonding agent in the resin-coating technique improved interfacial sealing and durability of all resin cements.
1
Introduction
The aesthetic aspect of dental treatment has become increasingly popular in the recent years, especially with the development of improved materials and adhesive techniques using composite resins. The indirect composite resin restoration technique involves extra-oral fabrication of an inlay and its placement with a resin cement. It has been reported that for large cavities, indirect restorations bear advantages over direct techniques such as improvements in anatomic form, contour, fracture resistance and wear resistance . Furthermore, extra-oral fabrication aids in the relief of residual stresses and ensures that the negative effects of polymerization shrinkage are confined to the thin layer of resin cement .
On the other hand, it is believed that the viscous resin cements may not provide dentin bonding comparable to dentin-bonding system (DBS) used for direct composite . This may affect the sealing ability of these cements and lead to lower penetration to tooth substrate and hence, lower bonding performances in comparison to DBS. Therefore, a resin coating technique for indirect restorations was introduced in which DBS and a low viscosity microfilled resin are applied to seal dentin surface after preparation, decreasing pulp irritation and postoperative sensitivity and improving bond strength . Meanwhile, the effectiveness of this technique for the newly introduced resin cement products (such as self-adhesive resin cements) has not been investigated. The self-adhesive resin cement is proposed to simplify the cementation procedure; it bonds to dentin in one step without the need of conditioning or pre-treatment (priming) of the surface .
Adhesion tests have been routinely used for laboratory evaluation of these biomaterials. However, the success of a restoration also greatly depends on its sealing ability of the dental tissue in an actual cavity . Different methods are conventionally used to evaluate the marginal integrity and sealing of restorations. The most common method is detecting dye penetration depth under a stereoscopic microscope and/or scanning electron microscope (SEM). However, these methods are considered as destructive methods since they require sample sectioning, and may be subjective. More recently, three-dimensional and in-depth imaging methods have been introduced and utilized for characterization of dental composites . Optical coherence tomography (OCT) can provide noninvasive, high resolution cross-sectional images for biologic microstructures and materials based on light backscattering from within the structure. Dental composites and hard tissues are scattering media and therefore can be suitable substrates for OCT imaging . Tooth-restoration interface under direct resin restorations has been investigated using this technique ; however, there are few reports on evaluation of indirect restorations.
Thermal cycling procedure has been accepted as an effective means of artificially aging composite restorations to study their interfacial characteristics in the long-term. In this regard, imaging of resin restorations by OCT before and after thermal aging appears to be an attractive research method. Therefore, the aim of this laboratory study was to evaluate the effect of thermal cycling and resin coating technique on the adaptation of indirect composite inlays luted with resin cements under OCT, and confirmation of OCT findings by cross-sectional confocal laser scanning microscopy (CLSM). The null hypotheses tested were as follows: (1) there was no difference in the interfacial sealing of the composites inlays between different resin cements; (2) the resin coating could not improve the interfacial integrity; and (3) There were no changes in the interfacial integrity of different test groups after thermal aging.
2
Materials and method
2.1
Specimen preparation
For this study, thirty extracted human third molars, free of cracks, caries and restorations were selected after the patients’ informed consent, as approved by the Institutional Review Board of Tokyo Medical and Dental University, Human Research Ethics Committee, protocol no. 725. The root structure was removed below the cement-enamel junction and in order to expose a flat dentin substrate; the occlusal thirds were removed by trimming the crowns at right angles to the long axis of the teeth using a model trimmer (Y-230; Yoshida, Tokyo, Japan). Round class I cavities were prepared on the flat occlusal surfaces by using a cylindrical diamond bur attached to a high-speed air turbine under water coolant (carborundum points, 50 μm grain size, SHOFU, Kyoto, Japan). Finishing diamond burs were used afterward to have a fine surface finish (SF114, SHOFU, Kyoto, Japan). To maintain cutting efficacy, the bur was replaced every five preparations. The cavity was approximately 4 mm in width and 2 mm in depth. The teeth were then randomly divided into two groups of fifteen teeth each according to the surface treatment. For the first group (control group), dentin surface was kept untreated. In the second group (resin-coated group), the cavity surface was prepared using the self-etching bonding system, Clearfil SE Bond (Kuraray Noritake Dental, Tokyo, Japan) and a low viscosity microfilled resin (Clearfil Protect Liner F, Kuraray Noritake Dental, Tokyo, Japan). According to the manufacturer’s instructions, SE primer was applied first to the cavity for 20 s and gently air dried. Then, SE bond was applied; mildly air dried and light cured for 20 s using a conventional halogen light curing unit (Optilux 501, Kerr, CA, USA; 550 mW/cm 2 ). After that, Protect Liner F was placed on the already cured adhesive surface with a brush and light cured for 20 s.
The cavities in both groups were then lined (covered) with a separating film (Pechiney Plastic Packaging, Chicago, IL, USA), filled with one increment of composite (Clearfil Majesty Posterior, Kuraray Noritake Dental, Tokyo, Japan), and light cured for 40 s using the light curing unit. After curing, the composite inlays were carefully removed from the cavities and checked for fit. The resin inlays were monitored under OCT prior to cementation and the defective ones were excluded and refabricated.
The prepared cavity surfaces in group 1 and the coated surfaces in group 2 were both temporized with a water-setting non-eugenol temporary filling material (Caviton EX, GC, Japan) and stored in an incubator at 37 °C in a humid condition to simulate the clinical situation for indirect composite restorations. After 24 h, the temporary filling material was carefully removed with a spoon excavator and surface was wiped with a cotton pellet containing ethanol for 10 s. The coated surfaces were then cleaned for 10 s using 37% phosphoric acid gel, rinsed and dried in order to remove any debris.
The fitting surfaces of the resin inlays were treated with 37% phosphoric acid gel for 15 s, rinsed with water and gently air dried. Then, Tokuso Ceramic Primer (Tokuyama Dental) was applied as a silane coupling agent to the surface and air-dried.
Specimens from each group were further divided into three subgroups according to the type of luting resin cement used. Table 1 lists the materials used in this study while Fig. 1 shows schematic drawing for the sample preparation.
Material (Abbreviation) Manufacturer Lot no. |
Composition | Procedure |
---|---|---|
Dentin bonding system | ||
Clearfil SE Bond (SE) Kuraray Noritake Dental 011595 |
Primer : MDP, HEMA, hydrophilic dimethacrylate, dl -camphorquinone, N,N-diethanol-p-toluidine, water. Bond : MDP, Bis-GMA, HEMA, hydrophobic dimethacrylate, dl -camphorquinone, N,N-diethanol-p-toluidine, silanated colloidal silica. |
Apply the primer for 20 s. Mild air blow. Apply adhesive and air blow gently. Light cure for 10 s. |
Low-viscosity microfilled resin | ||
Protect Liner F (PLF) Kuraray Noritake Dental 0074DA |
Bis-GMA, TEGDMA, fluoride-methyl methacrylate, camphorquinone, silanized colloidal silica, pre-polymerized organic filler. | Apply in a thin layer, light cure for 20 s. |
Resin cements | ||
Bistite II DC (BT) Tokuyama Dental 028012 |
Primer 1 (A and B) : phosphoric acid monomer, acetone, alcohol, water, initiator. Primer 2: HEMA, acetone, initiator. Resin cement pastes: Paste-A: NPGDMA, Bis-MPEPP, silica-zirconia filler. Paste-B: MAC-10, silica-zirconia filler, benzoylperoxide, photo-initiator. |
Apply primer 1A + 1B, leave for 30 s, air dry, apply primer 2, leave for 20 s, air-dry, place mixed paste A + B, light cure for 20 s. |
Clearfil SA Luting (SA) Kuraray Noritake Dental 0141AA |
Paste A : Bis-GMA, TEGDEMA, MDP, hydrophobic aromatic dimethacrylate, silanated barium glass filler, silanated colloidal silica, dl -camphorquinone, benzoyl peroxide, initiator. Paste B : Bis-GMA, hydrophobic aromatic dimethacrylate, hydrophobic aliphatic dimethacrylate, silanated barium glass filler, silanated colloidal silica, surface treated sodium fluoride, accelerators, pigments. |
Apply the cement paste mix to the restoration, place the restoration. Light cure for 2–5 s, and then remove the excess cement. Light cure for 20 s. |
Multibond II (MB) Tokuyama Dental 0780Z1 |
Primer: phosphoric acid monomer, water, acetone, UDMA, co-activator. liquid: MMA, UDMA, HEMA, MTU-6, borate catalyst. powder: PMMA, co-activator. |
Apply primer for 20 s and gently air dry for 10 s. Powder: liquid: 1:3 Mix for 5 s, apply to dentin surface. |
Indirect resin composite | ||
Clearfil Majesty Posterior (MP) Kuraray Noritake Dental 00111A |
Silanated glass ceramics, silanted silica filler, surface treated alumina microfiller, Bis-GMA, TEGDMA, hydrophobic aromatic dimethacrylate, dl -camphorquinone. | Bulk filling and light cure for 40 s. |
The resin cements used in this study were the dual-cure with self-etching primer Bistite II DC (Tokuyama Dental), the self-adhesive Clearfil SA Luting cement (Kuraray Noritake Dental, Tokyo, Japan) and the MMA-based self-etching chemically-cured Multibond II (Tokuyama Dental). Each of the three cements was applied according to the manufacturer’s instructions.
2.2
Thermocycling procedure
All specimens were then stored at 37 °C in humid condition for 24 h prior to the initial OCT imaging. Then, all the specimens were thermocycled for 10,000 cycles, which was roughly estimated to represent one year of clinical function approximately . They were fatigued between 5 °C and 55 °C with a dwell time of 30 s in each temperature, and a transfer time of 2 s between baths (Cool Line CL200 and Cool Mate TE200, Yamato Scientific Co., Tokyo, Japan). The specimens were subjected to OCT evaluation to detect any changes in the adaptation of the resin restorations after 2000 cycles and after completing 10,000 cycles.
2.3
OCT system
A swept-source OCT system (Santec OCT-2000, Santec Co., Komaki, Japan), was used in this study. The spectral bandwidth of the optical source is over 100 nm centered at 1310 nm at a 20 kHz sweep rate. The probe power is within the safety limits defined by American National Standard Institute. The sensitivity of this system and the shot-noise limited sensitivity are 106 and 119 dB, respectively. The axial resolution of the system is 11 μm in air, which corresponds to 7 μm in tissue assuming a refractive index of approximately 1.5. The lateral resolution depends on the objective lens at the probe and was 17 μm in this study. Backscattered light carrying information about the microstructure of the sample is collected, returned to the system, digitized in time scale and then analyzed in the Fourier domain to reveal the depth information of the subject. The system analyzes the frequency components of backscattered light from the sample and creates real-time high resolution 2-D image.
2.4
OCT imaging and analysis
Specimens were subjected to serial 2D scans 24 h after cementation, and after 2000 and 10,000 thermal cycles. To ensure the repeatability of the OCT scans for the same specimen, small holes were drilled on the specimen surface to make sure that specimens were placed at the same orientation as accurately as possible.
In order to capture OCT image, the specimen was positioned on a metal stage with a 3–5° tilt to avoid peculiar surface reflections. The surface of the specimen was blot dried using air duster to standardize the tooth surface hydration condition . Then, the focus light beam was projected onto the tooth surface at 90° and scanned across the cavity in three dimensions using OCT probe. In this manner, 20 serial 2D sections at 200 μm interval were obtained. The size of each image was 2000 × 1019 pixels corresponding to 5 mm × 6.6 mm ( x , z ). For the data analysis purpose, each of the 20 serial 2D sections was digitally analyzed using ImageJ (ver. 1.42q, National Institutes of Health, Bethesda, MD, USA). A custom computer code was developed as a plugin for ImageJ based on a binarization process previously reported , to facilitate image analysis procedure and distinguish pixel clusters with higher brightness indicating gap or unsealed interface at the cavity floor. The total cavity adaptation (including resin cement and/or coating interface) was calculated as
Cavity adaptation % = 1 − ∑ gap length at all cross-sections ∑ cavity floor length at all cross-sections × 100
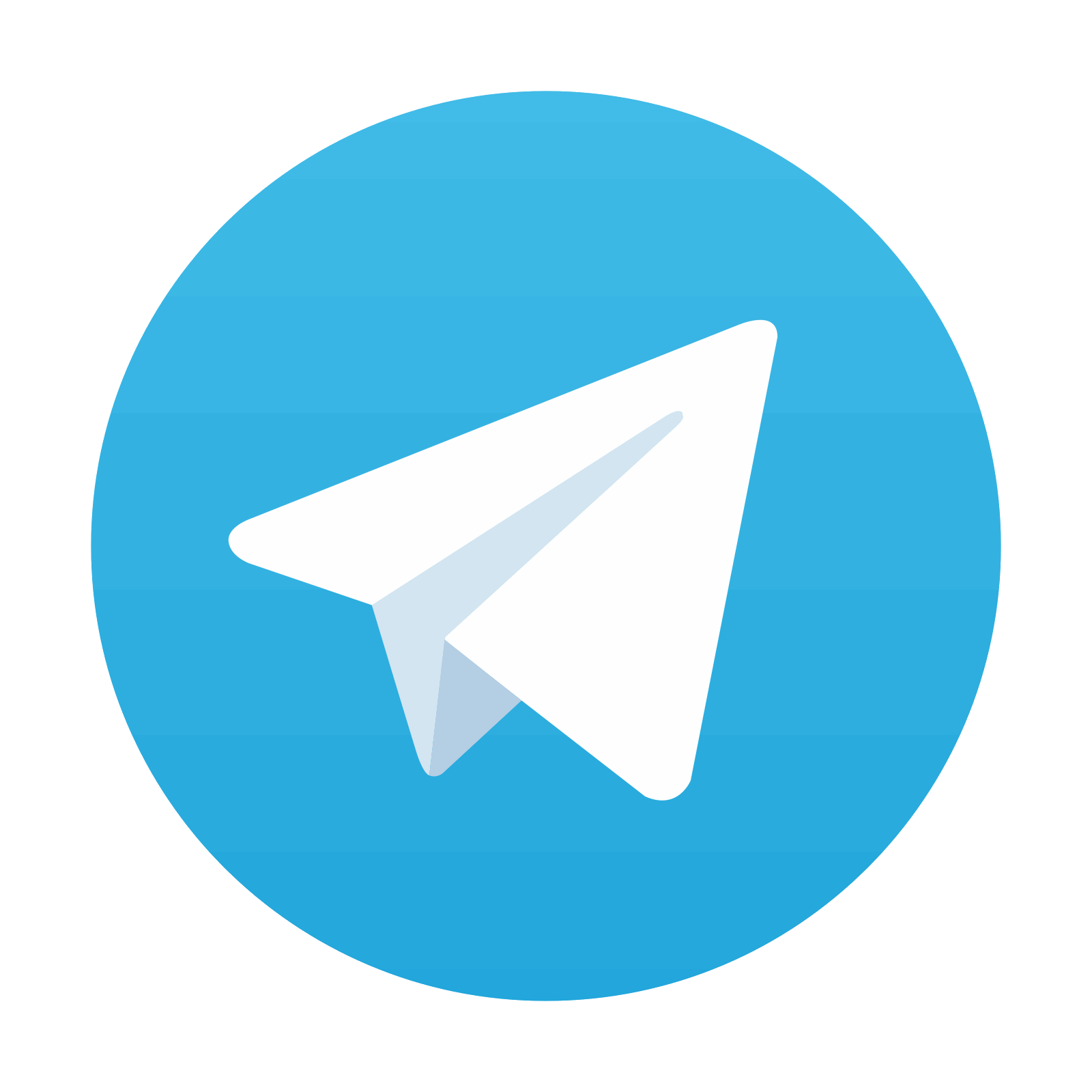
Stay updated, free dental videos. Join our Telegram channel

VIDEdental - Online dental courses
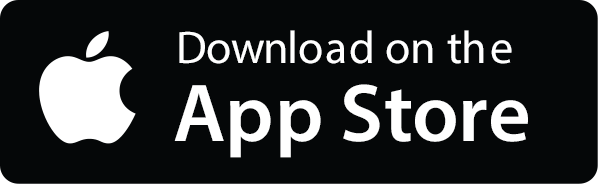
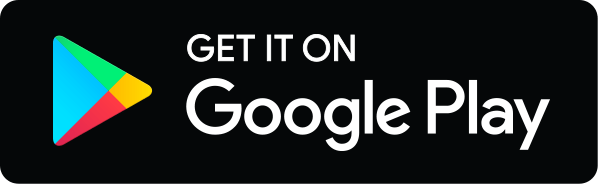